DOI:
10.1039/C6GC90029A
(Editorial)
Green Chem., 2016,
18, 1836-1838
Reflection and perspective on green chemistry development for chemical synthesis—Daoist insights
Western philosophy established the foundations of natural science. The rapid development of science and technology in our modern society is the manifestation of the great success of this philosophy. The ability to synthesize organic compounds in the laboratory set the stage for modern organic chemistry. The completion of total syntheses of complex molecules such as Vitamin B12, palytoxin, and taxol in the last century are representative of the many great achievements in chemical syntheses.1 Methods such as “retrosynthetic analysis” provide “blueprints” to tackle almost any complex organic molecule in a logical way, albeit in many cases through lengthy sequences of classical chemical transformations.2 However, with growing environmental, health and safety concerns of chemical products and processes as well as the consciousness of resource and energy sustainability, synthetic chemists began to shift from “what to synthesize?” to “how to synthesize?”, in terms of resource efficiency and process safety, which led to the birth of Green Chemistry under the leadership of Paul T. Anastas in 1991 in the US EPA Office of Pollution Prevention and Toxics.3
The past 25 years
The onset of Green Chemistry for chemical syntheses is marked by the concepts of atom-economy4 and the E-factor,5 together with the development of greener solvents such as water6 and supercritical CO2
7 to replace conventional organic solvents for reactions and processes. Atom-economy allows academic researchers to think about reaction design, focusing on overall incorporation of all atoms of the starting materials and reagents into the final products rather than the traditional percent yield of the final products only; whereas the E-factor provides a powerful tool for industry to compare different manufacturing sectors and processes in terms of overall material utilization efficiency in the form of total waste compared to final products. Early research on using water as a solvent was marked for its high reaction rate through “hydrophobic effects”,8 and the development of reactions traditionally regarded as “highly water-sensitive” such as “Grignard-type reactions”, Lewis acid catalysis and some transition-metal catalysed reactions, in water, which allow not only the use of water as a solvent but also simplifying protection–deprotection of various acid functionalities such as hydroxyl groups and carboxylic acids.9 The use of supercritical CO2 allows the ready removal of this solvent without leaving traces in the product, with excellent examples of its use in decaffeinated coffee, dry cleaning, and chemical reactions.10 Catalysis (homogeneous, heterogeneous, and biocatalysis) has also played a key role in organic syntheses11 since the beginning of Green Chemistry, with the 2005 Nobel Prize specifically noted for its Green Chemistry relevance.12
The creation of the concepts of Step-Economy13 and Reaction-Mass Efficiency,14 the advances in ionic liquids15 and fluorous solvents,16 as well as the study of biodegradable polymers represents some of the major developments in the field in academic research and in industrial applications related to chemical syntheses in subsequent years.
The publication of the 12 Principles of Green Chemistry (Fig. 1) in 1998 by Anastas and Warner was a turning point in the field.17 These principles provide scientists and engineers with logistical guidelines to design chemical reactions, products, and processes in terms of Green Chemistry. Since then, the field has been growing exponentially, assisted by the launch of Green Chemistry by the Royal Society of Chemistry (the flagship journal in the field).18
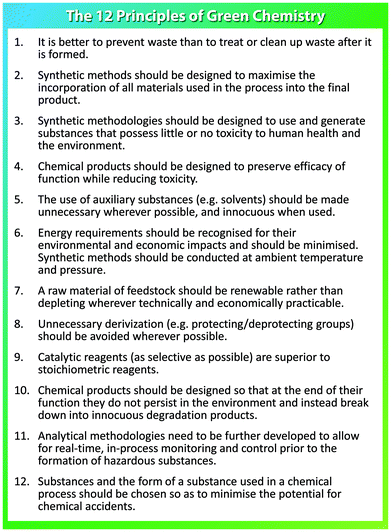 |
| Fig. 1 The 12 Principles of Green Chemistry | |
Subsequently, a wave of innovations in Green Chemistry has been seen across all facets of chemical science: from mining to biomass conversion, from materials to energy, from green separations to green engineering, from green reactor design to flow chemistry, and from directed evolution to earth abundant metal catalysts. During this time, the pharmaceutical industry has played a leading role in promoting greener chemical synthesis in industry with the establishment of the Pharmaceutical Roundtable by the Green Chemistry Institute of the American Chemical Society. The specific top challenges put up by the Pharmaceutical Roundtable provide academia with reactions for innovations, which will potentially benefit the entire pharmaceutical sector. Prominent innovations in synthetic chemistry during this period are represented by “Click Chemistry”,19 organocatalysis,20 Cross-Dehydrogenative Coupling (CDC) reactions,21 “synthesis-in-flow”,22 microwave synthesis,23 mechanosynthesis,24 visible light photo-catalysis,25 directed evolution26 and others. New concepts for solvent design such as the “smart solvent” and “switchable solvents”,27 as well as MeTHF have also appeared.
The future challenges
In contrast to western philosophy, eastern philosophy such as Daoism emphasizes natural harmony and balance,28 which resonates with the contemporary notion of sustainability. The potential benefit of Daoism in modern science has been recognized in physics early last century in the development of quantum theory etc.29 Its potential impact in chemistry is much less recognized. The principles of Green Chemistry and chemical sustainability echo with the Daoist philosophy, which can provide some perspectives regarding the future development of Green Chemistry for chemical synthesis.
The core teaching of Daoism is the so-called “Wu Wei”, which literally translates into “ado” or “do nothing”. The exact meaning of “Wu Wei”, based on Daoism, can only be understood but cannot be interpreted because any interpretation is only part of the meaning. From this angle, we can envisage some future developments in Green Chemistry towards chemical syntheses. In any synthesis, one often involves starting materials, solvent, energy (for heating), separation, and the reaction itself. The future developments in each aspect will be towards “do without”.
Starting materials: the interpretation here is “without using depleting starting materials”. Thus, the direct conversion of biomass such as lignin and cellulose into chemical products in high efficiency and high selectivity with no or minimal waste will be a major endeavour in future. In addition, the efficient activation of small abundant molecules such as N2, O2, CO2, water, and methane for efficient chemical synthesis will also become more and more important.
Solvents: the notion is that “the best solvent is no solvent”. Thus, many innovative solventless system will be developed for various chemical productions. New solvent systems analogous to “reactions on water” and “switchable solvents”, in which the solvent serves its function such as “dispersing”, “mixing” or “supporting” without having to dissolve or can be removed readily by a simple switch will appear more and more.
Energy input: the future development is “heating without thermal heating” in order to overcome the barrier of activation energy for chemical transformations. Classical chemical reactions often require thermal means such as refluxing to proceed, which are highly wasteful in energy as it is mostly applied to apparatus and solvent. New energy input methods that can directly act on the specific molecules and even the specific bond will be the future challenges. In this respect, photochemistry and electrochemistry will play more and more important roles in synthesis and other unforeseen new technologies will arise from this.
Separation: the challenge will be “separation without conventional separation”. How to separate product out from complex reaction mixtures without the need for traditionally highly wasteful or energy intensive re-crystallization, distillation or chromatographic means will see major breakthroughs in future chemical synthesis. Some promising advances have already been made in membrane technology, switchable systems, magnetic systems as well as flow reactor design to combine reactions with separations.
Reaction design: the motivation is “without the reaction”. How to optimize reactions quickly and how to minimize synthetic steps in synthesis will continue to play key roles in reaction design in future. In reaction discoveries, many reactions have to be carried out one-by-one in order to generate the optimal conditions, which are both time-consuming and very wasteful. Future research will see advances in ways to identify optimal conditions with no or very few reactions through computational chemistry or through new technologies yet to be discovered. Classical synthetic chemistry relies heavily on organic halides for various reactions such as carbanion, carbocation, carbene, radical, as well as cross-coupling reactions. Due to the prevailing existence of C–H, C–C and C–OH bonds in organic matter in nature, halogen-free synthesis based on direct C–H, C–C and C–OH activations, and late-stage functionalizations30 will be pillars of future synthetic reactions. In order to achieve such novel reactivities, various catalyst systems will become more and more important. Major progress will be made in the development of earth abundant metal catalysts and non-metal catalysts. On the other hand, new biological means such as Directed Evolution will produce highly efficient catalysts that not only have the high efficiency and high selectivity of traditional biocatalysts but also feature the broad substrate scope or allow the generation of new catalysts quickly for specific needs.
Conclusion
The great advances in organic chemistry over the past century have provided us with powerful knowledge to synthesize various complex products. While we have been able to do things for >100 years, it has often been by brute force or by resorting to complicated ways of tricking our molecules to follow non-intuitive pathways. What we need in future is to redefine elegance in chemical synthesis by being far more harmonious and rational. Green Chemistry started 25 years ago inspired innovations across the entire spectrum of chemical science and led to the inventive creation of various new concepts in chemical synthesis. These concepts formed the foundation of Green Chemistry for chemical synthesis since. Looking towards the future, the eastern philosophy of Daoism provides some insights for future development in organic synthesis, including for starting materials, energy input, product separations and reaction design. The possibility of innovation in Green Chemistry is endless and some of the best Green Chemistry methods, yet to be uncovered, cannot even be predicted, as manifested by the beginning line of Tao-Te-Ching: “The Tao that can be described is not the eternal Tao”.29
References
-
K. C. Nicolaou and E. J. Sorensen, Classics in Total Synthesis, VCH, 1996 Search PubMed.
- E. J. Corey, Angew. Chem., Int. Ed., 1991, 30, 455 CrossRef.
- P. T. Anastas and M. M. Kirchhoff, Acc. Chem. Res., 2002, 35, 686 CrossRef CAS PubMed.
- B. M. Trost, Science, 1991, 254, 1471 CAS.
- R. A. Sheldon, Chem. Technol., 1994, 38 CAS.
- C.-J. Li, Chem. Rev., 1993, 93, 2023 CrossRef CAS.
- P. G. Jessop, T. Ikariya and R. Noyori, Nature, 1994, 368, 231 CrossRef CAS.
- D. C. Rideout and R. Breslow, J. Am. Chem. Soc., 1980, 102, 7816 CrossRef CAS.
-
C.-J. Li and T.-H. Chan, Organic Reactions in Aqueous Media, John Wiley & Sons, New York, 1997 Search PubMed.
- W. Leitner, Acc. Chem. Res., 2002, 35, 746 CrossRef CAS PubMed.
-
R. A. Sheldon, I. W. C. E. Arends and U. Hanefeld, Green Chemistry and Catalysis, Wiley-VCH Verlag, 2007 Search PubMed.
-
http://www.nobelprize.org/nobel_prizes/chemistry/laureates/2005/press.html
.
- P. A. Wender, V. A. Verma, T. J. Paxton and T. H. Pillow, Acc. Chem. Res., 2008, 41, 40 CrossRef CAS PubMed.
-
A. Lapkin and D. Constable, Green Chemistry Metrics. Measuring and Monitoring Sustainable Processes, Wiley, 2008 Search PubMed.
-
Handbook of Green Chemistry-Green Solvents, ed. P. T. Anastas, P. Wassersheid and A. Stark, Wiley-VCH, Weinheim, 2010 Search PubMed.
-
The Handbook of Fluorous Chemistry, ed. J. A. Gladysz, D. P. Curran and I. T. Horvath, Wiley-VCH, Weinheim, 2004 Search PubMed.
-
P. T. Anastas and J. C. Warner, Green Chemistry Theory and Practice, Oxford University Press, New York, 1998 Search PubMed.
- J. H. Clark, Green Chem., 1999, 1, 1 RSC.
- S. Narayan, J. Muldoon, M. G. Finn, V. V. Fokin, H. C. Kolb and K. B. Sharpless, Angew. Chem., Int. Ed., 2005, 44, 3275 CrossRef CAS PubMed.
- G. Lelais, W. C. David and D. W. C. MacMillan, Aldrichimica Acta, 2006, 39, 79 CAS.
- C.-J. Li, Acc. Chem. Res., 2009, 42, 335 CrossRef CAS PubMed.
- D. A. Britz, A. N. Khlobystov, J. W. Wang, A. S. O'Neil, M. Poliakoff, A. Ardavan and G. A. D. Briggs, Chem. Commun., 2004, 176 RSC; I. R. Baxendale, J. Deeley, C. M. Griffiths-Jones, S. V. Ley, S. Saaby and G. K. Tranmer, Chem. Commun., 2006, 2566 RSC.
- C. O. Kappe, Angew. Chem., Int. Ed., 2004, 43, 6250 CrossRef CAS PubMed.
- C. R. Hickenboth, J. S. Moore, S. R. White, N. R. Sottos, J. Baudry1 and S. R. Wilson, Nature, 2007, 446, 423 CrossRef CAS PubMed.
- T. P. Yoon, M. A. Ischay and J. Du, Nat. Chem., 2010, 2, 527 CrossRef CAS PubMed.
- N. J. Turner, Nat. Chem. Biol., 2009, 5, 567 CrossRef CAS PubMed.
- P. G. Jessop, D. J. Heldebrant, L. Xiaowang, C. A. Eckert and C. L. Liotta, Nature, 2005, 436, 1102 CrossRef CAS PubMed.
-
F. Capra, The Tao of Physics, Shambhala, Boston, 3rd edn, 1991 Search PubMed.
-
C. Corrigan, The Tao of Holding Space, 2008 Search PubMed.
- T. Cernak, K. D. Dykstra, S. Tyagarajan, P. Vachal, W. Shane and S. W. Krska, Chem. Soc. Rev., 2016, 45, 546 RSC.
|
This journal is © The Royal Society of Chemistry 2016 |
Click here to see how this site uses Cookies. View our privacy policy here.