DOI:
10.1039/C5QO00248F
(Research Article)
Org. Chem. Front., 2016,
3, 43-46
Cu-catalyzed sequential C–N bond formations: expeditious synthesis of tetracyclic indoloindol-3-ones†
Received
7th August 2015
, Accepted 2nd November 2015
First published on 3rd November 2015
Abstract
The tetracyclic indoloindol-3-one core has been forged from easily accessible 2,2′-bis-bromochalcones employing a reaction cascade comprising Cu-catalyzed SNAr with azide; nitrene C–H insertion and intramolecular Ullmann reaction with all three C–N bond formations in one-go.
The synthesis of substituted indoles and of azaaurones [2-(benzylidene)indol-3-ones, also referred to as indoxyls] is an important aspect in organic synthesis mainly due to the occurrence of these substructural units in various biologically active natural products and/or clinically relevant entities.1,2 The tetracyclic indoloindol-3-one (Fig. 1) resulting from the fusion of these two scaffolds has been recently revealed by Biosynth AG as dye and as fluorogenic indicator for external stimuli such as temperature or vibrations, with a potential to act as fluorophore for bioconjugation.3 There are only very few reports on the synthesis of this type of scaffold in the literature.4–7 A general method available for the synthesis of this skeleton founded upon the coupling of the arynes with α-aminoesters has been independently reported by the Larock5 and Ramtohul6 groups (eqn (1)/(2), Fig. 1). Recently, we have documented a simple approach for the synthesis of 3-indolones and 2-aroylindoles comprising a one-pot construction of both C–N bonds present in these scaffolds employing the Cu-catalyzed SNAr of aryl halides using azide and the subsequent formation of the next C–N bond either via the intramolecular addition of a carbon centered nucleophile onto azide or the generation of nitrene followed by its insertion across the C–H bond.8 To further extend the scope of this approach, we presumed that if a suitable leaving group is available at the ortho position, the subsequent Ullmann coupling will complete the formation of the third C–N bond, thus leading to these indoloindol-3-one scaffolds in one pot – with the synchronized construction of all the three C–N bonds in one-go (eqn (3), Fig. 1).9 We focus on the feasibility of this one-pot [Cu]-catalysed synthesis of fused tetra cyclic indoloindol-3-one scaffolds from 2,2′-bis-bromochalcones.
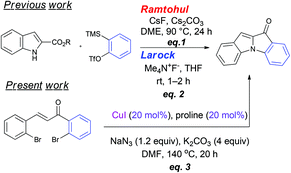 |
| Fig. 1 Methods for indoloindol-3-one synthesis. | |
Initial experiments with simple bisbromochalcone 1a
10 under the previously established conditions [20 mol% each of CuI and L-proline, of K2CO3 (4 equiv.) and NaN3 (1.2 equiv.) in NMP at 110 °C for 12 h] led to the formation of 2-aroylindole as one of the products. We speculated that the second cyclisation leading to the indolone ring is more demanding and increased the reaction temperature from 110 °C to 140 °C.11 This change gave the first optimistic result of fabrication of the parent indoloindol-3-one scaffold 2a. Subsequently, we moved towards the development of ambient reaction conditions. The optimisation of reaction conditions started from solvent screening. Various solvents were screened and DMF was found to be a better solvent with improvement in yield from 30% to 52% (Table 1, entries 1–4). Afterwards, the focus was shifted to check the copper (Cu) source other than CuI. However, it was found that CuI is the best copper source for the present cascade reaction (Table 1, entries 4–7).The presence of the base K2CO3 (4.0 equiv.) is required. However, other bases such as Cs2CO3, Na2CO3, NaOH and t-BuOK did not show any improvement in the yield (Table 1, entries 8–11). Increasing the concentration of the ligand L-proline from to 20 to 50 mol% did not show any fruitful effect on the reaction. The change in the azide concentration from 1.5 equiv. to 2.5 equiv. was also carried out but these changes did not improve the results (Table 1, entries 12–14). Hence the concentration of azide was kept as it is. The final reaction conditions were ascertained as, 20 mol% each of CuI and L-proline, 4 equiv. K2CO3 and 1.2 equiv. NaN3 in DMF at 140 °C for 20 h.
Table 1 Optimisation of reaction conditions
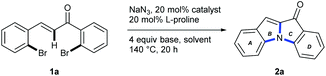
|
No. |
Solvent |
Catalyst (20 mol%) |
Base (4 equiv.) |
NaN3 (equiv.) |
Yield (%) |
Na-ascorbate used.
|
1 |
NMP |
CuI |
K2CO3 |
1.2 |
38 |
2 |
DMSO |
CuI |
K2CO3 |
1.2 |
30 |
3 |
DMA |
CuI |
K2CO3 |
1.2 |
35 |
4
|
DMF
|
CuI
|
K
2
CO
3
|
1.2
|
52
|
5 |
DMF |
CuBr |
K2CO3 |
1.2 |
23 |
6 |
DMF |
CuO |
K2CO3 |
1.2 |
10 |
7 |
DMF |
CuSO4 a |
K2CO3 |
1.2 |
26 |
8 |
DMF |
CuI |
Cs2CO3 |
1.2 |
36 |
9 |
DMF |
CuI |
Na2CO3 |
1.2 |
2 |
10 |
DMF |
CuI |
NaOH |
1.2 |
25 |
11 |
DMF |
CuI |
tBuOK |
1.2 |
32 |
12 |
DMF |
CuI |
K2CO3 |
1.5 |
45 |
13 |
DMF |
CuI |
K2CO3 |
2 |
42 |
14 |
DMF |
CuI |
K2CO3 |
2.5 |
44 |
Next, the generality of the current reaction has been examined by keeping mainly electron donating groups and halogens on either of the rings. Initially, the substrates were selected where the nature of the substituent on the ‘A’ ring was varied from methoxy to trimethoxy. With these substrates, the product yields improved marginally. Even the substrates with halogen groups were compatible under these conditions. In general, the yields are moderate for this one-pot three-step cascade (Table 2, entries 2b–2d). Similarly, when the same electron donating groups were placed on the other aryl ring, the comparative yields of the products improved. Interestingly, with the substrate having the 3,4,5-trimehoxyaroyl unit (Table 2, entry 2f), the demethylation of one of the methoxy groups was seen to occur.12 The symmetric 1H spectral pattern observed for this aryl unit in the 1H NMR spectrum of 2f revealed that the demethylation of the p-OMe occurred selectively. To check the generality of this demethylation, the scope of substrates by varying the groups on the ring “A” and by keeping the trimethoxy group on the ring “D” has been examined.
Table 2 Synthesis of various bis-bromochalcones and scope of the Cu-catalyzed sequential 3 × C–N bond formation
The results were consistent with respect to demethylation. The single crystal X-ray analysis of one of the compounds, 2i (Fig. 2), supported the assigned structure. In general, with these substrates having the trimethoxy groups present on the ring “D” the product yields are better than with the substrates where the trimethoxy groups are present on the ring A (Table 2, entries 2g–2i). However, substrates with trimethoxyaryl units took longer reaction times compared to the rest of the substrates. This may be due to the steric hindrance caused by one of the methoxy groups being ortho to bromine for the SNAr reaction or for the Ullmann coupling.
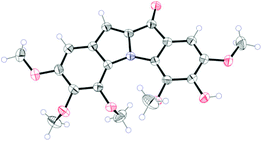 |
| Fig. 2 Single crystal X-ray structure of 2i (the ellipsoids are drawn at 50% probability. | |
Conclusions
In conclusion, a simple catalytic protocol for the preparation of the tetracyclic indoloindolone derivatives from easily accessible bis-bromochalcones has been developed. This Cu-catalyzed process presumably involves the following set of reactions – (i) SNAr with azide making the first C–N bond; (ii) the Cu-catalyzed conversion of azide to nitrene and subsequent intramolecular insertion of nitrene across the C–H bond with the net formation of second C–N bonds to give indole; and (iii) finally the intramolecular Ullmann reaction to form the third C–N bond thus leading to the formation of an indol-3-one ring. More detailed investigations on understanding the complete course of this reaction, improving the yields by exploring the possible combination of leaving groups, are the objectives of our future exploration in this context.
Acknowledgements
We thank DBT for funding this project.
Notes and references
- For selected reviews on indole syntheses, see:
(a)
J. A. Joule, Fused Five-Membered Hetarenes with One Heteroatom, in Science of Synthesis, ed. E. J. Thomas, Georg Thieme Verlag, Stuttgart, 2000, pp. 361–652 Search PubMed;
(b) T. L. Gilchrist, J. Chem. Soc., Perkin Trans. 1, 2001, 2491 RSC;
(c) L. Ackermann, Synlett, 2007, 507 CrossRef CAS;
(d)
A. R. Katritzky and A. F. Pozharskii, in Handbook of Heterocyclic Chemistry, Pergamon, Oxford, 2003 Search PubMed;
(e) K. Kruger, A. Tillack and M. Beller, Adv. Synth. Catal., 2008, 350, 2153 CrossRef;
(f) S. Cacchi, G. Fabrizi and A. Goggiamani, Org. Biomol. Chem., 2011, 9, 641 RSC;
(g) R. Vicente, Org. Biomol. Chem., 2011, 9, 6469 RSC;
(h) S. Cacchi and G. Fabrizi, Chem. Rev., 2011, 111, 215 CrossRef PubMed;
(i) N. K. Kaushik, N. Kaushik, P. Attri, N. Kumar, C. H. Kim, A. K. Verma and E. H. Choi, Molecules, 2013, 18, 6620 CrossRef CAS PubMed.
- For selected papers on azaaurones, see:
(a) M. Hooper and W. N. Pitkethl, J. Chem. Soc., Perkin Trans. 1, 1972, 1607 RSC;
(b) Z. W. An, M. Catellani and G. P. Chiusoli, J. Organomet. Chem., 1990, 397, 371 CrossRef CAS;
(c) B. Gerby, A. Boumendjel, M. Blanc, P. P. Bringuier, P. Champelovier, A. Fortune, X. Ronot and J. Boutonnat, Bioorg. Med. Chem. Lett., 2007, 17, 208 CrossRef CAS PubMed;
(d) F. Souard, S. Okombi, C. Beney, S. Chevalley, A. Valentin and A. Boumendjel, Bioorg. Med. Chem., 2010, 18, 5724 CrossRef CAS PubMed;
(e) N. Batail, M. Genelot, V. Dufaud, L. Joucla and L. Djakovitch, Catal. Today, 2011, 173, 2 CrossRef CAS;
(f) V. L. Gein, V. V. Tatarinov, N. A. Rassudikhina, M. I. Vakhrin and E. V. Voronina, Pharm. Chem. J., 2011, 45, 231 CrossRef CAS;
(g) C. Zwergel, F. Gaascht, S. Valente, M. Diederich, D. Bagrel and G. Kirsch, Nat. Prod. Commun., 2012, 7, 389 CAS;
(h) H. Watanabe, M. Ono, H. Kimura, K. Matsumura, M. Yoshimura, Y. Okamoto, M. Ihara, R. Takahashi and H. Saji, Bioorg. Med. Chem. Lett., 2012, 22, 5700 CrossRef CAS PubMed;
(i) M. C. Sharma, S. Sharma, P. Sharma and A. Kumar, Med. Chem. Res., 2014, 23, 181 CrossRef CAS.
-
U. Spitz, L. Wick, C. Weymuth, A. Bayer and G. Schabert, Eur. Patent application, EP2256103A1, 2010 Search PubMed.
- D. A. Shirley and P. A. Roussel, J. Am. Chem. Soc., 1953, 75, 375 CrossRef CAS.
- D. C. Rogness and R. C. Larock, Tetrahedron Lett., 2009, 50, 4003 CrossRef CAS PubMed.
- R. D. Giacometti and Y. K. Ramtohul, Synlett, 2009, 2010 CAS.
-
(a) D. Hong, Z. Chen, X. Lin and Y. Wang, Org. Lett., 2010, 12, 4608 CrossRef CAS PubMed;
(b) S. Hazra, B. Mondal, R. N. De and B. Roy, RSC Adv., 2015, 5, 22480 RSC.
-
(a) Y. Goriya and C. V. Ramana, Chem. Commun., 2013, 49, 6376 RSC;
(b) Y. Goriya and C. V. Ramana, Chem. Commun., 2014, 50, 7790 RSC.
- For selected papers SNAr with azide and subsequent intramolecular nitrogen atom transfer reactions, see:
(a) M. R. Kumar, A. Park, N. Park and S. Lee, Org. Lett., 2011, 13, 3542 CrossRef CAS PubMed;
(b) Y. Kim, M. R. Kumar, N. Park, Y. Heo and S. Lee, J. Org. Chem., 2011, 76, 9577 CrossRef CAS PubMed;
(c) K. Li, J. Chen, J. Li, Y. Chen, J. Qu, X. Guo, C. Chen and B. Chen, Eur. J. Org. Chem., 2013, 6246 CrossRef CAS;
(d) Y. Ou and N. Jiao, Chem. Commun., 2013, 49, 3473 RSC;
(e) X. Shang, S. Zhao, W. Chen, C. Chen and H. Qiu, Chem. – Eur. J., 2014, 20, 1825 CrossRef CAS PubMed;
(f) F. C. Jia, C. Xu, Z. W. Zhou, Q. Cai, D. K. Li and A. X. Wu, Org. Lett., 2015, 17, 2820 CrossRef CAS PubMed;
(g) B. Li, S. H. Guo, J. Zhang, X. Y. Zhang and X. S. Fan, J. Org. Chem., 2015, 80, 5444 CrossRef CAS PubMed.
-
(a) R. A. Bunce, V. L. Taylor and H. D. Reeves, Org. Prep. Proced. Int., 1989, 21, 337 CrossRef CAS;
(b) G. Markopoulos, L. Henneicke, J. Shen, Y. Okamoto, P. G. Jones and H. Hopf, Angew. Chem., Int. Ed., 2012, 51, 12884 CrossRef CAS PubMed.
- For related intramolecular Cu-catalyzed N-arylation in oxindole synthesis, see:
(a) B. Lu and D. Ma, Org. Lett., 2006, 8, 6115 CrossRef CAS PubMed;
(b) Y.-X. Jia and E. P. Kündig, Angew. Chem., Int. Ed., 2009, 48, 1636 CrossRef CAS PubMed;
(c) J. E. M. N. Klein, A. Perry, D. S. Pugh and R. J. K. Taylor, Org. Lett., 2010, 12, 3446 CrossRef CAS PubMed;
(d) C. L. Moody, V. Franckevičius, P. Drouhin, J. E. M. N. Klein and R. J. K. Taylor, Tetrahedron Lett., 2012, 53, 1897 CrossRef CAS;
(e) V. A. Ignatenko, N. Deligonul and R. Viswanathan, Org. Lett., 2010, 12, 3594 CrossRef CAS PubMed;
(f) T. Kukosha, N. Trufilkina and M. Katkevics, Synlett, 2011, 2525 CAS;
(g) J.-C. Hsieh, A.-Y. Cheng, J.-H. Fu and T.-W. Kang, Org. Biomol. Chem., 2012, 10, 6404 RSC;
(h) J.-C. Hsieh, Y.-C. Chen, A.-Y. Cheng and H.-C. Tseng, Org. Lett., 2012, 14, 1282 CrossRef CAS PubMed;
(i) Y.-H. Lee, Y.-C. Chen and J.-C. Hsieh, Eur. J. Org. Chem., 2012, 247 CrossRef CAS;
(j) Y.-H. Jhan, T.-W. Kang and J.-C. Hsieh, Tetrahedron Lett., 2013, 54, 1155 CrossRef CAS.
-
(a) O. Diels and F. Bunzl, Ber., 1905, 38, 1486 CrossRef;
(b) R. Burewell Jr., Chem. Rev., 1954, 54, 615 CrossRef.
Footnote |
† Electronic supplementary information (ESI) available. CCDC 1410863. For ESI and crystallographic data in CIF or other electronic format see DOI: 10.1039/c5qo00248f |
|
This journal is © the Partner Organisations 2016 |
Click here to see how this site uses Cookies. View our privacy policy here.