DOI:
10.1039/C5QO00400D
(Research Article)
Org. Chem. Front., 2016,
3, 273-276
A benzo[c]carbazolyl-based phosphine ligand for Pd-catalyzed tetra-ortho-substituted biaryl syntheses†
Received
25th November 2015
, Accepted 26th December 2015
First published on 28th December 2015
Abstract
A new benzo[c]carbazolyl-based phosphine ligand has been designed and synthesized. This newly developed ligand efficiently facilitates the Pd-catalyzed tetra-ortho-substituted biaryl syntheses via Suzuki–Miyaura cross-coupling. With 1 mol% of the Pd(OAc)2/L6 catalyst, sterically congested biaryls were afforded in good-to-excellent yields. In particular, the mild reaction conditions exhibited good compatibility of heterocycles and functional groups including esters and nitrile. L6 was structurally characterized by X-ray crystallographic analysis.
Palladium-catalyzed cross-coupling has become one of the most powerful methodologies for carbon–carbon bond-forming processes and biaryl constructions.1 Recent developments have been focused on the development of novel ancillary ligands equipped with different electronic and steric profiles for improving catalytic proficiency and enabling more challenging catalytic transformations.2 While ortho-substituted biaryl motifs are attractive scaffolds in biologically active compounds and pharmaceutical intermediates,3 preparation of sterically demanding tetra-ortho-substituted biaryls was found to be a persistent challenge.2a,b,4 The first successful report of such a difficult reaction can be dated back to Buchwald's work in 2002.5 Following this pioneering work, a limited number of metal complexes employing phosphine or N-heterocyclic carbene (NHC) by Organ,6 Ackermann,7 Tang,8 Nolan,9 Tu10 and others11 were also shown to be effective in promoting the tetra-ortho-substituted biaryl cross-couplings (Fig. 1). Despite these tremendous advances, most protocols have drawbacks of high catalyst loadings and limited substrate scope while the synthetic pathways of the ancillary ligands are often lengthy or involve the use of sophisticated organic building blocks. To further understand the chemistry of this area for the organic and synthetic community, it is desirable to develop a more easily accessible and general catalyst with high efficiency. Furthermore, the relationship between ligand characteristics and catalytic performance of this reaction is rather elusive. As part of our efforts in establishing efficient sterically demanding biaryl preparation processes, we describe herein a new benzo[c]carbazolyl-based phosphine ligand specifically useful for the tetra-ortho-substituted biaryl Suzuki coupling.
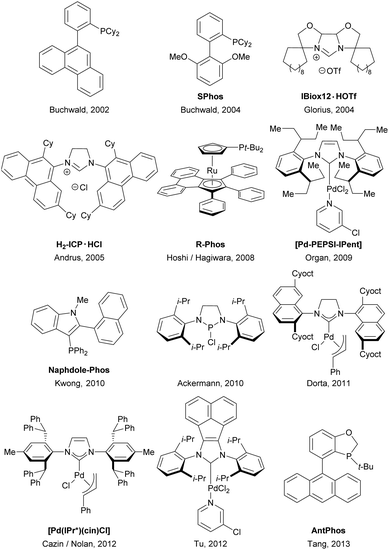 |
| Fig. 1 Palladium catalysts and ancillary ligands used in tetra-ortho-substituted biaryl syntheses. | |
In 2011, we reported PhenCar-Phos (Scheme 1) as an excellent ligand for the Pd-catalyzed tri-ortho-substituted biaryl coupling, in which a flattened carbazole ring facilitates the reductive elimination process and a flexible sp3-N–Pd coordination provides catalyst longevity.12 Owing to the initial success of PhenCar-Phos in the preparation of hindered tri-ortho-substituted biaryls, we were intrigued if the scaffold can be optimized to enable the tetra-ortho-substituted biaryl coupling. Whereas highly electron-rich and sterically congested ligands were demonstrated to promote the hindered biaryl synthesis, we envision that the success of the catalysis might not depend on the overwhelming electron richness and steric bulkiness, but the balance between these two factors. We have previously demonstrated that the optimal combination of these two aspects is the key of success for a particular catalytic reaction.13 To investigate our postulation, a series of carbazolyl-ligand bearing different dialkyl and diphenyl phosphino groups was evaluated by the model reaction between 2-bromomesitylene and 2,6-dimethylphenylboronic acid (Scheme 1). Intriguingly, PCy2-PhenCar-Phos (L1), which was successful in promoting tri-ortho-substituted biaryl synthesis,12 along with other electron-rich and bulky dialkylphosphine counterparts (L2–L4), were ineffective in this model reaction but L5 (which embodied only the –PPh2 moiety) was found to provide a modest product yield of 37%. It is well-recognized in the lore of the field that electron-rich dialkyl phosphines were able to promote demanding oxidative additions with Ar−Cl or even Ar−OMs bonds.14 As suggested, these results indicated that the reaction with L1–L4 might proceed with a facile oxidative addition followed by subsequent demanding transmetalation or reductive elimination. Taking the PhenCar-Phos ligand skeleton into account, we envisaged that the less steric bulkiness of the –PPh2 moiety in L5 could satisfy the transmetalation of the sterically congested 2,6-dimethylphenylboronic acid intermediate while facilitating a more effective reductive elimination than L4 in our system. In view of the demanding reductive elimination, we embarked to extend the carbazolyl framework in postulation to overcome this process and thus prepared the benzo[c]carbazolyl-based ligand L6. To our delight, the product yield was significantly increased to 59% with the use of the newly developed L6.
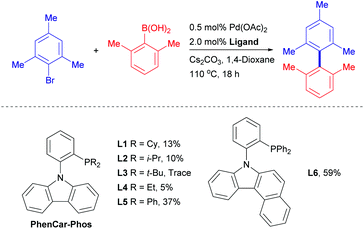 |
| Scheme 1 Ligand effect of carbazolyl-derived phosphines. Reaction conditions: Pd(OAc)2 (0.5 mol%), ligand (2.0 mol%), 2-bromomesitylene (0.3 mmol), 2,6-dimethylphenyl boronic acid (0.45 mmol), Cs2CO3 (0.9 mmol), and 1,4-dioxane (0.3 M, 1.0 mL) were stirred for 18 h at 110 °C under nitrogen. Calibrated GC yields are reported. | |
The ligand L6 was prepared by a straightforward and convenient ligand-free Cu-catalyzed C–N bond formation process between 1-bromo-2-iodobenzene and 7H-benzo[c]carbazole (Scheme 2), followed by phosphination with chlorodiphenylphosphine to give the final phosphine ligand in excellent yield. Notably, the synthetic procedure was amenable for multi-gram scaleup and the phosphino building block ClPPh2 is vastly cost-efficient.15 Single crystals of L6 suitable for X-ray diffraction were grown by liquid–liquid diffusion of hexane into a chloroform solution containing L6, and was fully characterized by crystallographic analysis (Fig. 2).
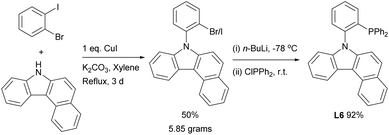 |
| Scheme 2 The synthesis of benzo[c]carbazolyl-based phosphine ligand L6. | |
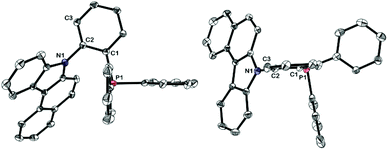 |
| Fig. 2 OPTEP diagrams of L6. All hydrogen atoms have been omitted for clarity. | |
Having the lead ligand candidate, we next carried on the optimization of reaction conditions to ensure the effectiveness of the Pd/L6 catalytic system (Table 1). Among an array of bases surveyed, K3PO4 was found to be a better base than Cs2CO3 while other inorganic and organic bases were inferior (entry 3 vs. 1 and 2 and 4–9). The presence of water equivalents in K3PO4·H2O led to a decrease in product yield to 35% (entry 4 vs. 3). It is noteworthy that the mild basic conditions with K3PO4 provided ample functional group tolerance, while ester or nitrile groups might not remain intact with the use of strong bases such as NaOt-Bu or KOH in some of the systems. With regard to solvent screening, 1,4-dioxane was found to be a more promising solvent than THF and the others (entry 3 vs. 10–13). When other palladium sources were used, the product yields experienced a significant drop of ∼20–40% (entry 3 vs. 14–17). The best metal to ligand ratio was identified to be 1
:
4 (entry 3 vs. 18–20).
Table 1 Reaction condition screening of Pd/L6 catalyst systema
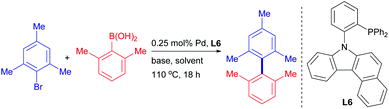
|
Entry |
Solvent |
Base |
Pd sourceb |
Yield |
Reaction conditions: Pd source (0.25 mol%), Pd : L6 = 1 : 4, 2-bromomesitylene (0.3 mmol), 2,6-dimethylphenyl boronic acid (0.45 mmol), base (0.9 mmol), and solvent (0.3 M, 1.0 mL) were stirred for 18 h at 110 °C under nitrogen. Calibrated GC yields are reported.
Mol% of the Pd monomer with respect to 2-bromomesitylene.
Pd(OAc)2 : L6 = 1 : 3.
Pd(OAc)2 : L6 = 1 : 2.
Pd(OAc)2 : L6 = 1 : 1.
|
1 |
1,4-Dioxane |
Cs2CO3 |
Pd(OAc)2 |
53 |
2 |
1,4-Dioxane |
K2CO3 |
Pd(OAc)2 |
16 |
3 |
1,4-Dioxane |
K3PO4 |
Pd(OAc)2 |
64 |
4 |
1,4-Dioxane |
K3PO4·H2O |
Pd(OAc)2 |
35 |
5 |
1,4-Dioxane |
Na3PO4 |
Pd(OAc)2 |
4 |
6 |
1,4-Dioxane |
CsF |
Pd(OAc)2 |
2 |
7 |
1,4-Dioxane |
KOH |
Pd(OAc)2 |
15 |
8 |
1,4-Dioxane |
NEt3 |
Pd(OAc)2 |
0 |
9 |
1,4-Dioxane |
DABCO |
Pd(OAc)2 |
0 |
10 |
Hexane |
K3PO4 |
Pd(OAc)2 |
2 |
11 |
THF |
K3PO4 |
Pd(OAc)2 |
57 |
12 |
Toluene |
K3PO4 |
Pd(OAc)2 |
51 |
13 |
t-BuOH |
K3PO4 |
Pd(OAc)2 |
32 |
14 |
1,4-Dioxane |
K3PO4 |
Pd(dba)2 |
30 |
15 |
1,4-Dioxane |
K3PO4 |
Pd2(dba)3 |
22 |
16 |
1,4-Dioxane |
K3PO4 |
PdCl2(ACN)2 |
46 |
17 |
1,4-Dioxane |
K3PO4 |
[PdCl(cinnamyl)]2 |
40 |
18 |
1,4-Dioxane |
K3PO4 |
Pd(OAc)2 |
62c |
19 |
1,4-Dioxane |
K3PO4 |
Pd(OAc)2 |
55d |
20 |
1,4-Dioxane |
K3PO4 |
Pd(OAc)2 |
30e |
With these promising results in hand, we next evaluated the scope of the Pd(OAc)2/L6 system in the cross-coupling of a variety of sterically hindered substrates (Table 2). Indeed, only 1 mol% of the Pd/L6 catalyst was enough to promote the reactions smoothly with full conversion of aryl halides. Sterically hindered aryl and naphthyl halides coupled well with 2,6-disubstituted phenyl or 2-methoxynaphthyl boronic acids and gave good product yields (60–80%, entries 3a–3e). Highly electron-deficient halopentafluoro benzene reacted smoothly (entries 3f–3g) and we found that the use of aryl chloride did not affect the catalytic performance in this entry. Sterically hindered heterocycles were also found to be applicable substrates in our system with satisfying yields (66–95%, entries 3h–3k). In particular, it is worthy of note that ester and nitrile groups in entries 3l–3q were compatible under these reaction conditions and the desired products were furnished in good-to-excellent yields (70–99%).
Table 2 Pd(OAc)2/L6-catalyzed sterically hindered Suzuki–Miyaura biaryl couplinga
Reaction conditions: Pd(OAc)2 (1.0 mol%), L6 (4.0 mol%), aryl halide (0.3 mmol), aryl boronic acid (0.45 mmol), K3PO4 (0.9 mmol), and 1,4-dioxane (0.3 M, 1.0 mL) were stirred for 18 h at 110 °C under nitrogen. Isolated yields are reported.
Homocoupling of 2-methoxynaphthylboronic acid was not observed when it was used in other entries.
Chloropentafluorobenzene was used instead.
|
|
Conclusions
In conclusion, the newly developed Pd(OAc)2/L6 catalyst proved to be efficient in promoting tetra-ortho-substituted biaryl synthesis using hindered aryl halides and arylboronic acids. We have demonstrated the optimization of an ineffective ligand to fit such a challenging reaction while ligands bearing electron-rich and bulky dialkyl phosphino groups (L1–L4) were inferior. The ligand skeleton of L6 can be prepared by a simple ligand-free Cu-catalyzed amination with easily accessible materials and was amenable for multigram-scale synthesis. We believe these ligands’ characteristics offer an important note for future phosphine ligand design in Pd-catalyzed sterically hindered biaryl synthesis. We envisaged that ligand L6 possesses axial chirality and future efforts will be focused on the resolution of the ligand for enantioselective catalysis.
Acknowledgements
We thank the Research Grants Council of Hong Kong (CRF: C5023-14G), the General Research Fund (PolyU 153008/14P), and the State Key Laboratory of Chirosciences for financial support. Grateful for the Equipment Grant (PolyU11/CRF/13E) for X-ray crystallographic analysis.
Notes and references
- For book chapters, see:
(a)
A. de Meijere and F. Diederich, Metal-Catalyzed Cross-Coupling Reactions, Wiley-VCH, Weinheim, 2nd edn, 2004, vol. 1–2 Search PubMed;
(b)
E. Negishi, Handbook of Organopalladium for Organic Synthesis, Wiley-Interscience, 2002, vol. 1–2 Search PubMed;
(c)
L. Ackermann, Modern Arylation Methods, Wiley-VCH, Weinheim, 2009 Search PubMed; For recent reviews, see:
(d) J.-P. Corbet and G. Mignani, Chem. Rev., 2006, 106, 2651–2710 CrossRef CAS PubMed;
(e) A. Roglans, A. Pla-Quintana and M. Moreno-Mañas, Chem. Rev., 2006, 106, 4622–4643 CrossRef CAS PubMed.
- For recent selected reviews on the development of ancillary ligands, see:
(a) C. Valente, S. Çalimsiz, K. H. Hoi, D. Mallik, M. Sayah and M. G. Organ, Angew. Chem., Int. Ed., 2012, 51, 3314–3332 CrossRef CAS PubMed;
(b) R. J. Lundgren and M. Stradiotto, Chem. – Eur. J., 2012, 18, 9758–9769 CrossRef CAS PubMed;
(c) S. M. Wong, O. Y. Yuen, P. Y. Choy and F. Y. Kwong, Coord. Chem. Rev., 2015, 293–294, 158–186 CrossRef;
(d) D. S. Surry and S. L. Buchwald, Chem. Sci., 2011, 2, 27–50 RSC;
(e) D. S. Surry and S. L. Buchwald, Angew. Chem., Int. Ed., 2008, 47, 6338–6361 CrossRef PubMed;
(f) F. Izquierdo, S. Manzini and S. P. Nolan, Chem. Commun., 2014, 50, 14926–14937 RSC;
(g) G. C. Fu, Acc. Chem. Res., 2008, 41, 1555–1564 CrossRef CAS PubMed.
-
(a) M. C. Kozlowski, B. J. Morgan and E. C. Linton, Chem. Soc. Rev., 2009, 38, 3193–3207 RSC;
(b) G. Bringmann, T. Gulder, T. A. M. Gulder and M. Breuning, Chem. Rev., 2011, 111, 563–639 CrossRef CAS PubMed;
(c) K. C. Nicolaou, P. G. Bulger and D. Sarlah, Angew. Chem., Int. Ed., 2005, 44, 4442–4489 CrossRef CAS PubMed.
-
(a) S. Vuoti, J. Autio, M. Haukka and J. Pursiainen, Inorg. Chim. Acta, 2009, 362, 4685–4691 CrossRef CAS;
(b) T. E. Barder, S. D. Walker, J. R. Martinelli and S. L. Buchwald, J. Am. Chem. Soc., 2005, 127, 4685–4696 CrossRef CAS PubMed;
(c) C. Wolf and H. Xu, J. Org. Chem., 2008, 73, 162–167 CrossRef CAS PubMed.
- J. Yin, M. P. Rainka, X.-X. Zhang and S. L. Buchwald, J. Am. Chem. Soc., 2002, 124, 1162–1163 CrossRef CAS PubMed.
-
(a) S. Calimsiz, M. Sayah, D. Mallik and M. G. Organ, Angew. Chem., Int. Ed., 2010, 49, 2014–2017 CrossRef CAS PubMed;
(b) M. G. Organ, S. Calimsiz, M. Sayah, K. H. Hoi and A. J. Lough, Angew. Chem., Int. Ed., 2009, 48, 2383–2387 CrossRef CAS PubMed.
- L. Ackermann, H. K. Potukuchi, A. Althammer, R. Born and P. Mayer, Org. Lett., 2010, 12, 1004–1007 CrossRef CAS PubMed.
-
(a) W. Tang, A. G. Capacci, X. Wei, W. Li, A. White, N. D. Patel, J. Savoie, J. J. Gao, S. Rodriguez, B. Qu, N. Haddad, B. Z. Lu, D. Krishnamurthy, N. K. Yee and C. H. Senanayake, Angew. Chem., Int. Ed., 2010, 49, 5879–5883 CrossRef CAS PubMed;
(b) Q. Zhao, C. Li, C. H. Senanayake and W. Tang, Chem. – Eur. J., 2013, 19, 2261–2265 CrossRef CAS PubMed;
(c) G. Liu, G. Xu, R. Luo and W. Tang, Synlett, 2013, 2465–2471 CAS.
- A. Chartoire, M. Lesieur, L. Falivene, A. M. Slawin, L. Cavallo, C. S. Cazin and S. P. Nolan, Chem. – Eur. J., 2012, 18, 4517–4521 CrossRef CAS PubMed.
- T. Tu, Z. Sun, W. Fang, M. Xu and Y. Zhou, Org. Lett., 2012, 14, 4250–4253 CrossRef CAS PubMed.
-
(a) L. Wu, E. Drinkel, F. Gaggia, S. Capolicchio, A. Linden, L. Falivene, L. Cavallo and R. Dorta, Chem. – Eur. J., 2011, 17, 12886–12890 CrossRef CAS PubMed;
(b) T. Hoshi, T. Nakazawa, I. Saitoh, A. Mori, T. Suzuki, J.-i. Sakai and H. Hagiwara, Org. Lett., 2008, 10, 2063–2066 CrossRef CAS PubMed;
(c) G.-Q. Li, Y. Yamamoto and N. Miyaura, Synlett, 2011, 1769–1773 CAS;
(d) C. M. So, W. K. Chow, P. Y. Choy, C. P. Lau and F. Y. Kwong, Chem. – Eur. J., 2010, 16, 7996–8001 CrossRef CAS PubMed;
(e) M. Lesieur, A. M. Z. Slawin and C. S. J. Cazin, Org. Biomol. Chem., 2014, 12, 5586–5589 RSC;
(f) M. Giannerini, V. Hornillos, C. Vila, M. Fañanás-Mastral and B. L. Feringa, Angew. Chem., Int. Ed., 2013, 52, 13329–13333 CrossRef CAS PubMed;
(g) G. Altenhoff, R. Goddard, C. W. Lehmann and F. Glorius, J. Am. Chem. Soc., 2004, 126, 15195–15201 CrossRef CAS PubMed;
(h) O. M. Demchuk, B. Yoruk, T. Blackburn and V. Snieckus, Synlett, 2006, 2908–2913 CrossRef CAS;
(i) D.-H. Lee and M.-J. Jin, Org. Lett., 2011, 13, 252–255 CrossRef CAS PubMed;
(j) S. D. Walker, T. E. Barder, J. R. Martinelli and S. L. Buchwald, Angew. Chem., Int. Ed., 2004, 43, 1871–1876 CrossRef CAS PubMed;
(k) C. Song, Y. Ma, Q. Chai, C. Ma, W. Jiang and M. B. Andrus, Tetrahedron, 2005, 61, 7438–7446 CrossRef CAS.
- S. C. To and F. Y. Kwong, Chem. Commun., 2011, 47, 5079–5081 RSC; For an example of commercial availability, see: http://www.strem.com/catalog/family/PhenCar-Phos/.
- W. C. Fu, C. M. So, W. K. Chow, O. Y. Yuen and F. Y. Kwong, Org. Lett., 2015, 17, 4612–4615 CrossRef CAS PubMed.
- For recent selected reviews on the use of dialkylarylphosphines in ArCl and ArOMs cross-couplings:
(a) C. M. So and F. Y. Kwong, Chem. Soc. Rev., 2011, 40, 4963–4972 RSC;
(b) R. Martin and S. L. Buchwald, Acc. Chem. Res., 2008, 41, 1461–1473 CrossRef CAS PubMed;
(c) M. Miura, Angew. Chem., Int. Ed., 2004, 43, 2201–2203 CrossRef CAS PubMed.
- According to the 2014 Aldrich catalogue, ClPPh2 (337.5 USD/500 g) is far less expensive than chlorodialkylphosphines such as ClPCy2 (271.5 USD/5 g) and ClP(t-Bu)2 (311.5 USD/25 g).
Footnote |
† Electronic supplementary information (ESI) available: Detailed experimental procedures and characterization data for isolated products. CCDC 1438740. For ESI and crystallographic data in CIF or other electronic format see DOI: 10.1039/C5QO00400D |
|
This journal is © the Partner Organisations 2016 |
Click here to see how this site uses Cookies. View our privacy policy here.