DOI:
10.1039/C6QO00064A
(Research Article)
Org. Chem. Front., 2016,
3, 620-624
Oxidative trifluoromethylthiolation and thiocyanation of amines: a general approach to N–S bond formation†
Received
9th February 2016
, Accepted 8th March 2016
First published on 16th March 2016
Abstract
Herein, we described a practical and efficient one-pot oxidative trifluoromethylthiolation of amines. A panel of primary and secondary amines was functionalized in good yields at room temperature. This general approach was further applied to the thiocyanation of anilines and the corresponding products can be readily used as SCN electrophilic sources.
Over the last few years, the organofluorine research field has witnessed a rapid expansion1 which could be explained by the number of pharmaceuticals and agrochemicals containing at least one fluorine atom.2 Indeed, due to the unique features of the fluorine atom to modify the biological and physical properties of a molecule,3 its presence is often crucial for the discovery of biorelevant molecules. Consequently, a large panel of fluorinated groups is available and their introduction onto molecules can be realized by means of various transformations. In this blossoming field, the SCF3 moiety is now considered as an emerging fluorinated group thanks to its interesting features such as its high lipophilicity and its strong electron-withdrawing character.4 In this context, several original and elegant transformations to introduce the SCF3 group onto unsaturated and aliphatic derivatives have been reported.5 Very recently, special attention has been paid to the formation of the N–SCF3 bond. Indeed, trifluoromethanesulfenamides and more generally sulfenamide-containing molecules are compounds of interest. These key molecules have already demonstrated interesting applications in the rubber industry, in agrochemistry and in pharmaceutical research for instance.6 This particular interest results from the good lipophilicity of the N–SCF3 group (Hansch parameter, πR = 1.50) compared to other fluorinated groups.7 In addition, molecules bearing a N–SCF3 group appeared as versatile electrophilic sources of SCF3.5a,b As a consequence, several research groups reported elegant synthetic pathways to access these derivatives (Fig. 1). To avoid the use of toxic and volatile reagents (e.g. ClSCF3, (SCF3)2),8 Langlois, Billard and co-workers9a reported the synthesis of trifluoromethanesulfenamides using primary amines, diethylaminosulfur trifluoride (DAST™) and the Ruppert–Prakash reagent (TMSCF3). Note that secondary amines were unreactive under these conditions. Later, Billard and co-workers overcame that limitation by designing a base-mediated transamination process involving reagent I.9b In 2014, Rueping and co-workers reported the electrophilic trifluoromethylthiolation of aliphatic and benzylic amines using the Munavalli reagent II. However, in the case of anilines and secondary amines, the reaction required the additional use of a strong base (n-BuLi), limiting the scope of the process.7a Shen and co-workers designed a new SCF3 electrophilic reagent (N-trifluoromethylthiosaccharin III), which allowed the functionalization of amines within a panel of nucleophiles.9c Moreover, in 2015, the group of Shibata depicted an alternative pathway to synthesize a broad range of trifluoromethanesulfenamides by means of a copper-catalyzed transformation with the electrophilic trifluoromethanesulfonyl hypervalent iodonium ylide IV.7b In light of these considerations, we aimed at developing a general and straightforward access to trifluoromethanesulfenamides from amines. This approach avoids the employment of the commonly used electrophilic SCF3 reagents, usually prepared from a nucleophilic SCF3 source.
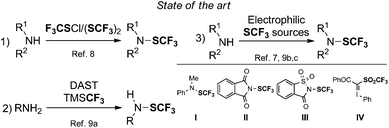 |
| Fig. 1 State of the art. | |
To build up the N–SCF3 bond, we envisioned an oxidative trifluoromethylthiolation of amine derivatives using a nucleophilic SCF3 source (AgSCF3) and an oxidant (Fig. 2).10 We hypothesized that a N-halo amine derivative could be in situ generated from the reaction between an amine and a suitable oxidant.11 The latter would then undergo an anionic metathesis with AgSCF3 to afford the expected product. To achieve this goal, we anticipated that the main synthetic issues to tackle would be: (1) the selective generation of the required N-halo (aryl)amine A prior to the formation of the unreactive electrophilic SCF3 source, resulting from the in situ reaction between the oxidant and AgSCF3
12 (Fig. 2); and (2) the possible competitive intramolecular halogenation of the aromatic ring13 over the desired anionic metathesis with the silver salt.
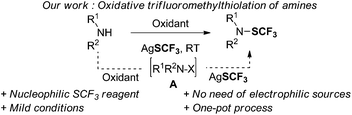 |
| Fig. 2 Proposed strategy. | |
Keeping these considerations in mind, we initially investigated the N-trifluoromethylthiolation of 4-fluoroaniline 1d in the presence of a stoichiometric amount of AgSCF3 and N-bromophthalimide (N-Br-Phth) as an oxidant in CH2Cl2 at rt. Under these reaction conditions, we were pleased to observe traces of the expected product 2d (Table 1, entry 1). Gratifyingly, the replacement of N-Br-Phth by its chlorinated analogue provided a significant enhancement of the reaction yield since 2d was obtained in 79% NMR yield (Table 1, entry 2), whereas the use of the N-chlorosaccharin provided 2d in a poor yield (Table 1, entry 3). Finally, we found that NCS was the best oxidant giving 2d in 86% NMR yield (Table 1, entry 4). Then, a screening of solvents revealed that THF was highly beneficial for the desired transformation (Table 1, entries 4–7). Moreover, we observed that the transformation turned out to be quite robust since the reaction performed under an air atmosphere gave a similar NMR yield (98%) and 2d was isolated in 92% yield (Table 1, entry 8).
Table 1 Optimization of the reaction conditionsa
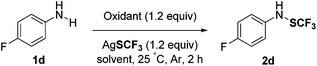
|
Entry |
Oxidant |
Solvent |
Yieldb (%) |
Reaction conditions: 1 (0.2 mmol), oxidant (0.24 mmol, 1.2 equiv.), AgSCF3 (0.24 mmol, 1.2 equiv.), solvent (0.1 M), 25 °C, argon, 2 h.
NMR yield determined by 19F NMR using α,α,α-trifluoroacetophenone as an internal standard.
Reaction run on a 0.1 mmol scale.
Reaction performed under an air atmosphere.
Isolated yield. Phth = phthalimide. Sacc = saccharin. NCS = N-chlorosuccinimide.
|
1 |
N-Br-Phth
|
CH2Cl2 |
7 |
2 |
N-Cl-Phth
|
CH2Cl2 |
79 |
3 |
N-Cl-Sacc
|
CH2Cl2 |
11 |
4 |
NCS
|
CH2Cl2 |
86 |
5c |
NCS
|
CH3CN |
41 |
6c |
NCS
|
Toluene |
83 |
7c |
NCS
|
THF |
97 |
8c,d |
NCS
|
THF |
98 (92)e |
With the best conditions in hand, we investigated the scope of the reaction (Table 2). First, a wide range of primary aniline derivatives with different substitution patterns was evaluated. The reaction proceeded smoothly with aromatic rings bearing either an electron-donating (tBu and OMe, 2b and 2c) or an electron-withdrawing group (halogens or CF3; 2d–g) showcasing the functional group tolerance of the process. The substitution pattern on the aromatic ring does not have an influence on the outcome of the reaction since meta-substituted anilines with various substituents (2h–2j) were obtained in good yields (up to 93%). Notably, the presence of halogens on the aryl group (2f, 2g, 2j and 2k) might allow further functionalizations through cross-coupling reactions, for instance. Di-and tri-substituted anilines (1k and 1l) provided the expected trifluoromethanesulfenamides 2k and 2l, albeit in lower yields. To further demonstrate the synthetic utility of such an approach, a reaction has been performed on a 3 mmol scale and the expected compound 2a was obtained in 88% yield. Interestingly, the secondary amine, N-methylaniline 1m, was converted into the expected compound 2m in a moderate yield. Importantly, this approach constitutes an alternative and straightforward access to the electrophilic Billard–Langlois reagents (2a and 2m). It is worth mentioning that heteroarylamines (2n and 2o) were also functionalized in decent yields. Moreover, primary and secondary aliphatic amines were suitable substrates giving the corresponding trifluoromethanesulfenamides in fairly good yields (2p–2u). The versatility of the method was further illustrated through the synthesis of the SCF3-containing analogues of biorelevant molecules such as L-phenylalanine and tryptamine derivatives (2s and 2t) in 42% and 83% yields, respectively. Note that in the case of 2t, the selective functionalization of the primary amine over the aromatic ring was observed. Even the diamine 1u was functionalized demonstrating the possibility to use secondary amines albeit in lower yield.14
Table 2 Scope of the trifluoromethylthiolation reaction of amine derivativesa
Reaction conditions: 1 (0.2 mmol), NCS (0.24 mmol, 1.2 equiv.), AgSCF3 (0.24 mmol, 1.2 equiv.), THF (0.1 M), 25 °C, air, 2 h.
The reaction was carried out on a 3 mmol scale.
20 h.
24 h.
12 h.
8 h.
The reaction was carried out on a 0.5 mmol scale.
4 h.
2.4 equiv. of AgSCF3 were used.
1 equiv. of Et3N was added.
2.4 equiv. of NCS were used.
|
|
Besides, organic thiocyanates are an important and versatile class of compounds with large potential for applications in several research fields.15 The intrinsic ability of the SCN moiety to be converted into other functional groups makes this functional group very appealing from a synthetic point of view. As a consequence, the development of new and efficient methodologies to synthesize these key thiocyanate building blocks has been depicted. In this context, we have been interested in extending our strategy to the N–SCN bond formation. To our delight, the above-described strategy was successfully applied to the thiocyanation of aniline derivatives showcasing the generality of this approach. Several anilines bearing different substitution patterns were functionalized in good yields (71–87%) on a large scale (2 or 3 mmol) offering an efficient synthetic access to such derivatives (Table 3). The aniline derivative 3a was synthesized in 76% yield, and even the sterically hindered substrate 3b was functionalized in good yield despite a longer reaction time. Halogen atoms like fluorine and chlorine were tolerated (3c and 3d) showcasing the functional group tolerance of the process. Interestingly, the inexpensive NaSCN can be used as a nucleophilic SCN source furnishing 3c in a similar yield (75%), a real asset for the reaction on a larger scale.16
Table 3 Scope of the thiocyanation reaction of aniline derivativesa
Reaction conditions: 1 (2 mmol), NCS (2.4 mmol, 1.2 equiv.), AgSCN (2.4 mmol, 1.2 equiv.), THF (0.1 M), 25 °C, air, 2 h.
The reaction was carried out on a 3 mmol scale.
18 h instead of 2 h.
The reaction was carried out on a 0.2 mmol scale and NaSCN was used instead of AgSCN.
|
|
As an alternative to the unstable thiocyanogen (SCN)2, N-thiocyanatosuccinimide (NTS)16,17 and in analogy with the Billard–Langlois reagent, these N-(cyanosulfanyl)aniline derivatives 3 might be considered as SCN electrophilic sources. To further demonstrate their synthetic utility, reactions with nucleophiles were carried out. Pleasingly, Friedel Crafts-type reactions with a non-protected indole and 1,3,5-trimethoxybenzene furnished the desired thiocyanates 4 and 5 in high yields. This represents a straightforward method for the introduction of the thiocyanate group onto aromatic and heteroaromatic compounds (Scheme 1).
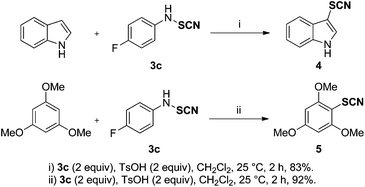 |
| Scheme 1 Application of 3c as an electrophilic SCN source. | |
From a mechanistic point of view, two plausible pathways might be envisioned for the trifluoromethylthiolation transformation: (1) the chlorination of the amine followed by an anionic metathesis with AgSCF3 or (2) the in situ formation of the SCF3 electrophilic source followed by the nucleophilic attack of the amine.18 To ascertain that there is no reaction with the electrophilic SCF3 source that might be in situ generated from NCS and AgSCF3,19 we carried out reactions of 1d and 1m in the presence of 1-(trifluoromethylthio)pyrrolidine-2,5-dione in THF (Scheme 2). Interestingly, both reactions did not provide any trace of products 2d and 2m after 2 h by 19F NMR. In light of these results, the following mechanism might be proposed: first, the chlorination of the amine 1 by NCS would lead to a plausible N-chloroamine as an intermediate. This latter might undergo an anionic metathesis in the presence of AgSCF3 to afford the expected product 2.
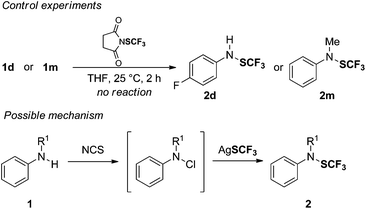 |
| Scheme 2 Control experiments and the proposed mechanism. | |
In conclusion, we have developed an oxidative trifluoromethylthiolation of amine derivatives using AgSCF3 as the nucleophilic source under mild conditions. This methodology offers an easy, safe and practical access to the valuable trifluoromethanesulfenamides. The developed strategy avoids the use of electrophilic sources, which require extra synthesis steps. The preliminary results regarding the mechanism indicate a two-step and one-pot process. Moreover, this strategy was successfully applied to the synthesis of original N-(cyanosulfanyl)aniline derivatives and their features to act as electrophilic SCN sources were demonstrated. Beyond doubt, these results would significantly broaden the toolbox for the synthesis of trifluoromethanesulfenamides and organic thiocyanate derivatives.
This work has been partially supported by INSA de Rouen, Université de Rouen, CNRS, LABEX SynOrg (ANR-11-LABX-0029), EFRD and Région Haute-Normandie (CRUNCh network). H.-Y. X. thanks the CSC for a doctoral fellowship.
Notes and references
- For selected reviews, see:
(a) For a special issue on fluorine chemistry, Chem. Rev., 2015, 115, 563; ;
(b) G. Landelle, A. Panossian, S. Pazenok, J.-P. Vors and F. R. Leroux, Beilstein J. Org. Chem., 2013, 9, 2476 CrossRef PubMed;
(c) T. Furuya, A. S. Kamlet and T. Ritter, Nature, 2011, 473, 470 CrossRef CAS PubMed;
(d) T. Liang, C. N. Neumann and T. Ritter, Angew. Chem., Int. Ed., 2013, 52, 8214 CrossRef CAS PubMed;
(e) T. Besset, T. Poisson and X. Pannecoucke, Chem. – Eur. J., 2014, 20, 16830 CrossRef CAS PubMed;
(f) C. Ni, M. Hu and J. Hu, Chem. Rev., 2015, 115, 765 CrossRef CAS PubMed;
(g) T. Besset, C. Schneider and D. Cahard, Angew. Chem., Int. Ed., 2012, 51, 5048 CrossRef CAS PubMed.
-
(a) J. Wang, M. Sánchez-Roselló, J. L. Aceña, C. del Pozo, A. E. Sorochinsky, S. Fustero, V. A. Soloshonok and H. Liu, Chem. Rev., 2014, 114, 2432 CrossRef CAS PubMed;
(b) E. A. Ilardi, E. Vitaku and J. T. Njardarson, J. Med. Chem., 2014, 57, 2832 CrossRef CAS PubMed;
(c) E. P. Gillis, K. J. Eastman, M. D. Hill, D. J. Donnelly and N. A. Meanwell, J. Med. Chem., 2015, 58, 8315 CrossRef CAS PubMed;
(d) S. Purser, P. R. Moore, S. Swallow and V. Gouverneur, Chem. Soc. Rev., 2008, 37, 320 RSC;
(e) G. Landelle, A. Panossian and F. R. Leroux, Curr. Top. Med. Chem., 2014, 14, 941 CrossRef CAS PubMed.
-
(a) D. O'Hagan, Chem. Soc. Rev., 2008, 37, 308 RSC;
(b)
R. D. Chambers, Fluorine in Organic Chemistry, Blackwell Publishing Ltd., Oxford, UK, 2004 Search PubMed;
(c)
K. Uneyama, Organofluorine Chemistry, Blackwell Publishing Ltd., Oxford, UK, 2006 Search PubMed.
-
(a) C. Hansch, A. Leo and R. W. Taft, Chem. Rev., 1991, 91, 165 CrossRef CAS;
(b) C. Hansch, A. Leo, S. H. Unger, K. H. Kim, D. Nikaitani and E. J. Lien, J. Med. Chem., 1973, 16, 1207 CrossRef CAS PubMed.
- For selected reviews, see:
(a) F. Toulgoat, S. Alazet and T. Billard, Eur. J. Org. Chem., 2014, 2415 CrossRef CAS;
(b) X.-H. Xu, K. Matsuzaki and N. Shibata, Chem. Rev., 2015, 115, 731 CrossRef CAS PubMed; for selected examples, see:
(c) S. Alazet, L. Zimmer and T. Billard, Chem. – Eur. J., 2014, 20, 8589 CrossRef CAS PubMed;
(d) B. Exner, B. Bayarmagnai, F. Jia and L. J. Goossen, Chem. – Eur. J., 2015, 21, 17220 CrossRef CAS PubMed;
(e) M. Hu, J. Rong, W. Miao, C. Ni, Y. Han and J. Hu, Org. Lett., 2014, 16, 2030 CrossRef CAS PubMed;
(f) F. Hu, X.-X. Shao, D.-H. Zu, L. Lu and Q. Shen, Angew. Chem., Int. Ed., 2014, 53, 6105 CrossRef CAS PubMed;
(g) J.-B. Liu, X.-H. Xu, Z.-H. Chen and F.-L. Qing, Angew. Chem., Int. Ed., 2015, 54, 897 CrossRef CAS PubMed;
(h) T. Yang, L. Lu and Q. Shen, Chem. Commun., 2015, 51, 5479 RSC;
(i) H.-Y. Xiong, T. Besset, D. Cahard and X. Pannecoucke, J. Org. Chem., 2015, 80, 4204 CrossRef CAS PubMed;
(j) K.-Y. Ye, X. Zhang, L.-X. Dai and S.-L. You, J. Org. Chem., 2014, 79, 12106 CrossRef CAS PubMed;
(k) Z. Huang, Y.-D. Yang, E. Tokunaga and N. Shibata, Org. Lett., 2015, 17, 1063 CrossRef PubMed;
(l) G. Danoun, B. Bayarmagnai, M. F. Gruenberg and L. J. Goossen, Chem. Sci., 2014, 5, 1312 RSC;
(m) K. Zhang, J.-B. Liu and F.-L. Qing, Chem. Commun., 2014, 50, 14157 RSC;
(n) R. Pluta, P. Nikolaienko and M. Rueping, Angew. Chem., Int. Ed., 2014, 53, 1650 CrossRef CAS PubMed. For recent examples using NaSO2CF3 as a source of SCF3, see:
(o) L. Jiang, J. Qian, W. Yi, G. Lu, C. Cai and W. Zhang, Angew. Chem., Int. Ed., 2015, 54, 14965 CrossRef CAS PubMed;
(p) Y. Yang, L. Xu, S. Yu, X. Liu, Y. Zhang and D. A. Vicic, Chem. – Eur. J., 2016, 22, 858 CrossRef CAS PubMed.
-
(a) L. Craine and M. Raban, Chem. Rev., 1989, 89, 689 CrossRef CAS; For a selected example, see:
(b) C. Lee, Y. N. Lim and H.-Y. Jang, Eur. J. Org. Chem., 2015, 5934 CrossRef CAS and references therein.
-
(a) R. Pluta and M. Rueping, Chem. – Eur. J., 2014, 20, 17315 CrossRef CAS PubMed;
(b) Z. Huang, Y.-D. Yang, E. Tokunaga and N. Shibata, Asian J. Org. Chem., 2015, 4, 525 CrossRef CAS.
- For selected examples, see:
(a) L. M. Yagupol'skii, A. V. Bezdudnyi and Y. L. Yagupol'skii, Russ. J. Org. Chem., 2006, 42, 1275 CrossRef;
(b) A. Kolasa and M. Lieb, J. Fluorine Chem., 1995, 70, 45 CrossRef CAS;
(c) M. Geisel and R. Mews, Chem. Ber., 1987, 120, 1675 CrossRef CAS;
(d) O. D. Gupta, W. A. Kamil and J. M. Shreeve, Inorg. Chem., 1985, 24, 2126 CrossRef CAS;
(e) S. Munavalli, D. K. Rohrbaugh, D. I. Rossman, F. J. Berg, G. W. Wagner and H. D. Durst, Synth. Commun., 2000, 30, 2847 CrossRef CAS;
(f) S. Munavalli, D. K. Rohrbaugh, D. I. Rossman, W. G. Wagner and H. D. Durst, Phosphorus, Sulfur Silicon Relat. Elem., 2003, 178, 107 CrossRef CAS;
(g) D. Kost and H. Egozy, J. Org. Chem., 1989, 54, 4909 CrossRef CAS;
(h) M. E. Peach, Can. J. Chem., 1967, 45, 429 CrossRef CAS.
-
(a) A. Ferry, T. Billard, B. R. Langlois and E. Bacqué, J. Org. Chem., 2008, 73, 9362 CrossRef CAS PubMed;
(b) S. Alazet, K. Ollivier and T. Billard, Beilstein J. Org. Chem., 2013, 9, 2354 CrossRef PubMed and references therein;
(c) C. Xu, B. Ma and Q. Shen, Angew. Chem., Int. Ed., 2014, 53, 9316 CrossRef CAS PubMed.
- For a relative two-step approach focusing on the trifluoromethylthiolation reaction of sulfoximines, see: C. Bohnen and C. Bolm, Org. Lett., 2015, 17, 3011 CrossRef CAS PubMed.
- R. C. Samanta and H. Yamamoto, Chem. – Eur. J., 2015, 21, 11976 CrossRef CAS PubMed.
- For examples of the in situ generation of an electrophilic SCF3 source from an oxidant and AgSCF3, see:
(a) S.-Q. Zhu, X.-H. Xu and F.-L. Qing, Eur. J. Org. Chem., 2014, 4453 CrossRef CAS;
(b) X.-L. Zhu, J.-H. Xu, D.-J. Cheng, L.-J. Zhao, X.-Y. Liu and B. Tan, Org. Lett., 2014, 16, 2192 CrossRef CAS PubMed;
(c) Note that Rueping and co-workers demonstrated that the Munavalli reagent was not suitable to perform the direct trifluoromethylthiolation of aniline derivatives without the addition of n-BuLi, see ref. 7a. Moreover, anilines 1d and 1m were unreactive with 1-((trifluoromethyl)thio)pyrrolidine-2,5-dione, see Scheme 2, and ESI† for details.
- P. Haberfield and D. Paul, J. Am. Chem. Soc., 1965, 87, 5502 CrossRef CAS.
- Note that in the case of sulfonamide derivatives, our standard procedure did not work and a sequential two-step process was required to obtain the expected compound. The use of TCCA (trichloroisocyanuric acid) instead of NCS was necessary and the reaction with AgSCF3 led to the corresponding trifluoromethylthiolated sulfonamide in only 31% yield (see the ESI,† compound 2v).
- For a review, see:
(a) T. Castanheiro, J. Suffert, M. Donnard and M. Gulea, Chem. Soc. Rev., 2016, 45, 494 RSC; For selected articles, see:
(b) D. Zhu, D. Chang and L. Shi, Chem. Commun., 2015, 51, 7180 RSC;
(c) R. Frei, T. Courant, Ma. D. Wodrich and J. Waser, Chem. – Eur. J., 2015, 21, 2662 CrossRef CAS PubMed;
(d) B. Bayarmagnai, C. Matheis, K. Jouvin and L. J. Goossen, Angew. Chem., Int. Ed., 2015, 54, 5753 CrossRef CAS PubMed;
(e) D. Yang, K. Yan, W. Wei, G. Li, S. Lu, C. Zhao, L. Tian and H. Wang, J. Org. Chem., 2015, 80, 11073 CrossRef CAS PubMed;
(f) T. Castanheiro, M. Gulea, M. Donnard and J. Suffert, Eur. J. Org. Chem., 2014, 7814 CrossRef CAS.
- M. T. Ashby and H. Aneetha, J. Am. Chem. Soc., 2004, 126, 10216 CrossRef CAS PubMed.
-
(a) F. D. Toste, V. De Stefano and I. W. J. Still, Synth. Commun., 1995, 25, 1277 CrossRef CAS;
(b) F. D. Toste and I. W. J. Still, J. Am. Chem. Soc., 1995, 117, 7261 CrossRef CAS.
- Note that at this stage, we cannot deny the in situ formation of the (SCF3)2 species from NCS and AgSCF3 (see ref. 12b) as effective electrophilic sources. However, the stoichiometry of the oxidant (1.2 equiv.) and of AgSCF3 (1.2 equiv.) makes this pathway unlikely. Indeed, to achieve the observed yields (up to 93%) at least two equivalents of oxidant should be necessary.
- C. Xu and Q. Shen, Org. Lett., 2014, 16, 2046 CrossRef CAS PubMed.
Footnote |
† Electronic supplementary information (ESI) available. See DOI: 10.1039/c6qo00064a |
|
This journal is © the Partner Organisations 2016 |
Click here to see how this site uses Cookies. View our privacy policy here.