DOI:
10.1039/C6QO00116E
(Research Article)
Org. Chem. Front., 2016,
3, 953-956
Synthesis of biaryl sultams using visible-light-promoted denitrogenative cyclization of 1,2,3,4-benzothiatriazine-1,1-dioxides†
Received
23rd March 2016
, Accepted 5th June 2016
First published on 7th June 2016
Abstract
A novel approach has been reported for the preparation of biaryl sultams using visible-light-promoted denitrogenative cyclization of 1,2,3,4-benzothiatriazine-1,1-dioxides. A variety of biaryl sultams have been prepared with the assistance of a photocatalyst under visible light at room temperature with satisfactory yields. Both stepwise and one-pot procedures have been developed, thus improving the practicability of this synthetic strategy.
Introduction
Cyclic sulfonamides, also named sultams, are important motifs in many natural products and biologically active compounds.1 In particular, biaryl sultams have found use as anti-inflammatory drugs (Fig. 1).2,3 For example, carbapenem-derived biaryl sultam A provides for potent binding to the target penicillin binding proteins (PBPs), including the normally low-affinity PBP2a of MRS and the overproduced, low-affinity PBP5 of resistant enterococci.2 The related quinoline-derived biaryl sultam B plays an active role in the NF-κB pathway, which has provided a favorable target for pharmacological intervention for chronic inflammation, neurodegenerative diseases, and certain types of cancer.3 Therefore, a variety of methods for the synthesis of biaryl sultams have been reported.4–6 One of the most popular methods for the synthesis of biaryl sultams based on the C–C bond formation is the intramolecular arylation of the 2-halobenzenesulfonamide derivative I catalyzed by palladium or with the assistance of a single-electron-transfer pathway (Fig. 1a).4 Palladium-catalyzed intramolecular oxidative coupling of sulfonanilide II is also feasible (Fig. 1b).5 Alternatively, the synthetic strategy based on the C–N bond formation is also known (Fig. 1c).6a Intramolecular oxidative C–H amination of an elaborated precursor 2-phenylarylsulfonamide III under metal-free conditions can give a biaryl sultam. Despite these advances, the development of a simple and novel protocol from easily synthesized starting materials towards the biaryl sultam motif is still desirable.
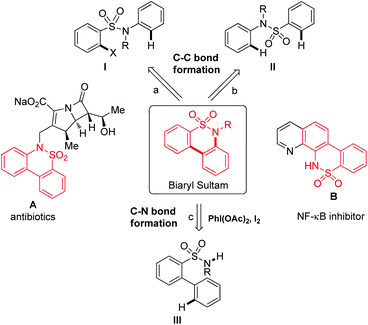 |
| Fig. 1 Biaryl sultam-containing biologically active molecules and their known synthetic strategies. | |
Results and discussion
Visible light photoredox catalysis, which takes advantage of the redox potential of the photocatalyst under irradiation, has recently emerged as a powerful tool to promote useful organic transformations.7 Very recently, we have developed the synthesis of isoquinolones using visible-light-promoted denitrogenative alkyne insertion of 1,2,3-benzotriazinones via a single-electron-transfer pathway (Scheme 1a).8 The reduced 1,2,3-benzotriazinone can prompt extrusion of a molecular dinitrogen giving the key aryl radical anion intermediate. This intermediate can undergo radical alkyne insertion followed by cyclization and oxidation to give the isoquinolone. Inspired by this work, we planned to synthesize benzosultams using visible-light-promoted denitrogenative alkyne insertion of 1,2,3,4-benzothiatriazine-1,1-dioxides (Scheme 1b).9 When triazine 1a and phenylacetylene were subjected to our established photoredox conditions, no benzosultam IV was observed. Interestingly, biaryl sultam 2a was isolated in very good yield (84%) instead. This phenomenon indicated that the alkyne was not involved in this reaction. When this reaction was repeated without adding phenylacetylene, biaryl sultam 2a was obtained with even better yield (91%). These preliminary results prompted us to explore this denitrogenative cyclization of 1,2,3,4-benzothiatriazine-1,1-dioxides under visible light photoredox catalysis systematically.
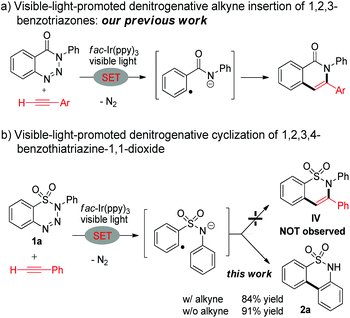 |
| Scheme 1 Visible-light-promoted denitrogenative cyclization of triazines. | |
A solution of triazine 1a in DMSO was irradiated by blue LEDs in the presence of the photocatalyst fac-Ir(ppy)3 for 2 h at room temperature. Then the sultam 2a was generated in 91% isolated yield (Table 1, entry 1). When a variety of solvents (DMA, DMF, MeOH, MeCN, CH2Cl2 and THF) were tested, no improved yield was observed (entries 2–7). The photocatalysts were then examined. When Ru(bpy)3Cl2 was used as the photocatalyst, the yield was as high as 98% (entry 8). Other photocatalysts, such as Ir(dFCF3ppy)2(dtbbpy)PF6, Ru(Phen)(PF6)2, Ir(ppy)2(dtbbpy)PF6, and Ru(bpy)3(PF6)2, and organic dyes, were also suitable in this reaction with 88–96% yields (entries 9–14). Control experiments verified the necessity of the photocatalyst and visible light irradiation. Without the photocatalyst, the desired product 2a was obtained in 13% isolated yield in 2 h (entry 15).10 Almost no conversion was observed without visible light irradiation and the starting material was fully recovered (entry 16).
Table 1 Reaction condition optimizationa
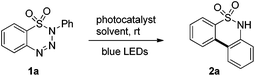
|
Entry |
Photocatalyst |
Solvent |
2a/%b |
Reaction conditions: a solution of 1a (0.2 mmol), and photocatalyst (0.002 mmol, 1.0 mol%) in the indicated solvent (2.0 mL) was irradiated by blue LED strips at rt for 2 h.
Isolated yield.
Yield for the recovered 1a.
In the dark.
|
1 |
Ir(ppy)3 |
DMSO |
91 |
2 |
Ir(ppy)3 |
DMA |
79 |
3 |
Ir(ppy)3 |
DMF |
77 |
4 |
Ir(ppy)3 |
MeOH |
73 |
5 |
Ir(ppy)3 |
CH3CN |
67 |
6 |
Ir(ppy)3 |
CH2Cl2 |
30 |
7 |
Ir(ppy)3 |
THF |
22 |
8 |
Ru(bpy)3Cl2 |
DMSO |
98 |
9 |
Ir(dFCF3ppy)2(dtbbpy)PF6 |
DMSO |
81 |
10 |
Ru(Phen)(PF6)2 |
DMSO |
96 |
11 |
Ir(ppy)2(dtbbpy)PF6 |
DMSO |
88 |
12 |
Ru(bpy)3(PF6)2 |
DMSO |
96 |
13 |
Fluorescein |
DMSO |
90 |
14 |
Eosin Y |
DMSO |
89 |
15 |
None |
DMSO |
13 (75c) |
16d |
Ru(bpy)3Cl2 |
DMSO |
2 (97c) |
With the established optimized reaction conditions in hand, we proceeded to explore the scope of this transformation, and the results are summarized in Scheme 2. The steric effect can affect this transformation drastically. ortho-, meta-, and para-methoxyl groups on the aniline subunit gave significantly different yields of biaryl sultams 2j, 2k, and 2b. Triazene with the para-methoxyl group afforded the biaryl sultam 2b in excellent yield while ortho-methoxyl aniline-derived triazine gave the sultam 2j in only 46% yield. meta-Methoxyl aniline-derived triazine gave a 1
:
1 mixture of two regioisomers 2k and 2k′ in 87% combined yield. The electronic property was then investigated and it did not affect this transformation significantly. Substituted 1,2,3,4-benzothiatriazine-1,1-dioxides with electron-donating, electron-neutral and electron-withdrawing groups on the aniline subunit worked smoothly to produce the corresponding biaryl sultams 2b–2i in good yields (82–96%). The acetyl (2h) and ester groups (2i) can tolerate this reaction. Biaryl sultams 2l and 2m could also be prepared by means of this method in quite good yields.
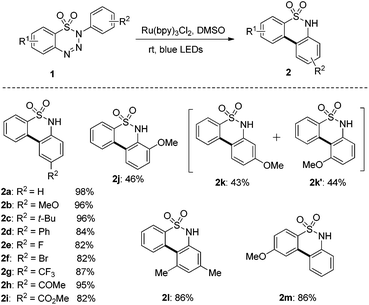 |
| Scheme 2 Synthesis of biaryl sultams from 1,2,3,4-benzothiatriazine-1,1-dioxide. Reaction conditions: a solution of 1 (0.2 mmol) and Ru(bpy)3Cl2 (0.002 mmol, 1.0 mol%) in dry DMSO (2.0 mL) was irradiated by blue LED strips at rt for 2 h. Yields are for the isolated products. | |
1,2,3,4-Benzothiatriazine-1,1-dioxides were prepared by diazotization of the corresponding 2-amino-N-phenylbenzenesulfonamides and the yields for these precursors were not always satisfactory (see the ESI†). Some of them decompose slowly during storage. In order to overcome this disadvantage and improve the overall efficiency of this method, we next investigate the possibility of the synthesis of biaryl sultams from 2-amino-N-phenylbenzenesulfonamide with the generation of triazines in situ. To our delight, when 2-amino-N-phenylbenzenesulfonamides were subjected to our established conditions in the presence of t-BuONO, the sequential diazotization3,11 and denitrogenative cyclization worked quite well in a one-pot manner. The corresponding sultams were prepared with improved overall yields compared to the stepwise procedure (Scheme 3). More importantly, sultam 2o, which cannot be synthesized by a stepwise procedure due to the instability of its corresponding triazine, can be prepared in satisfactory yield by a one-pot procedure from the aniline precursor.
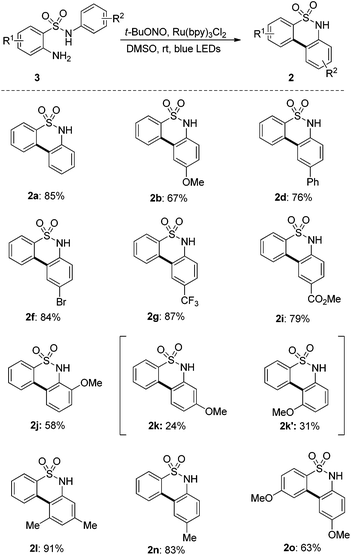 |
| Scheme 3 Synthesis of biaryl sultams from amino sulfonamides. Reaction conditions: a solution of 3 (0.2 mmol), t-BuONO (0.3 mmol) and Ru(bpy)3Cl2 (0.002 mmol, 1.0 mol%) in dry DMSO (2.0 mL) was irradiated by blue LED strips for 1.5 h. Yields are for the isolated products. | |
To gain some insights into the mechanism of this reaction, the Stern–Volmer analysis was carried out to determine the quencher of the excited photocatalyst.7a The luminescence of Ru(bpy)3Cl2 at 452 nm could be readily quenched by 1a following the Stern–Volmer kinetics (Fig. 2). The luminescence quenching is probably due to the photo-induced electron transfer.
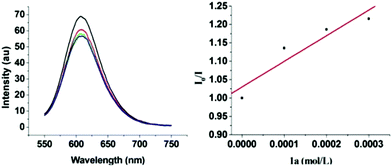 |
| Fig. 2 Luminescence quenching of Ru(bpy)3Cl2 with excitation at 452 nm by 1a (left) and the Stern–Volmer plot (right). | |
On the basis of the experimental observation and our previous work on the denitrogenative alkyne insertion of 1,2,3-benzotriazinones,8 a plausible mechanism is proposed (Fig. 3). First, the photocatalyst RuII is irradiated to the excited state RuII* under blue LEDs. The excited state RuII* is then oxidatively quenched by 1a with concomitant generation of the RuIII complex and radical anion 4 respectively. The radical anion 4 undergoes an intramolecular homolytic aromatic substitution (HAS) process to form the radical anion 5. Radical 5 is oxidized by the RuIII complex to yield cation 6 with the regeneration of the photocatalyst RuII. Finally, sultam 2a is generated after the loss of proton.
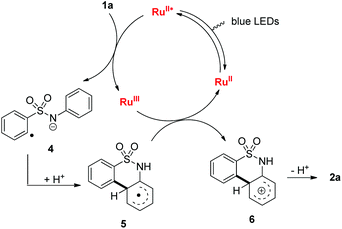 |
| Fig. 3 Proposed mechanism. | |
Conclusions
In summary, we have described the synthesis of biaryl sultams by visible-light-promoted denitrogenative cyclization of 1,2,3,4-benzothiatriazine-1,1-dioxides. Both stepwise and one-pot procedures worked quite well. The method presented here provides a convenient route to the preparation of biaryl sultams.
Acknowledgements
Financial support from the National Natural Science Foundation of China (21472084 and 81421091), the Qing Lan Project of Jiangsu Province and the Shanghai Institute of Organic Chemistry, CAS is acknowledged.
Notes and references
-
(a) M. Jimenez-Hopkins and P. R. Hanson, Org. Lett., 2008, 10, 2223–2226 CrossRef CAS PubMed;
(b) J. Drews, Science, 2000, 287, 1960–1964 CrossRef CAS PubMed;
(c) A. Scozzafava, T. Owa, A. Mastrolorenzo and C. T. Supuran, Curr. Med. Chem., 2003, 10, 925–953 CrossRef CAS PubMed;
(d) S. Sailes, H. Hanessian and E. Therrien, Tetrahedron, 2003, 59, 7047–7056 CrossRef;
(e) H. Tanimuka, M. Inui, S. Hariguch and Z. Kaneko, Biochem. Pharmacol., 1965, 14, 961–970 CrossRef;
(f) G. J. Wells, M. Tao, K. A. Josef and R. Bihovsky, J. Med. Chem., 2001, 44, 3488–3503 CrossRef CAS PubMed;
(g) F. Saczewski, J. Slawinski, A. Kornicka, Z. Brzozowski, E. Pomarnacka, A. Innocenti, A. Scozzafava and C. T. Supuran, Bioorg. Med. Chem. Lett., 2006, 16, 4846–4851 CrossRef CAS PubMed;
(h) Z. Brzozowski, F. Saczewski and N. Neamati, Bioorg. Med. Chem. Lett., 2006, 16, 5298–5302 CrossRef CAS PubMed;
(i) M. Sellstedt and F. Almqvist, Org. Lett., 2009, 11, 5470–5472 CrossRef CAS PubMed;
(j) J. Moeker, T. S. Peat, L. F. Bornaghi, D. Vullo, C. T. Supuran and S. A. Poulsen, J. Med. Chem., 2014, 57, 3522–3531 CrossRef CAS PubMed;
(k) S. Debnath and S. Mondal, J. Org. Chem., 2015, 80, 3940–3948 CrossRef CAS PubMed;
(l) S. Bag, R. Tulsan, A. Sood, H. Cho, H. Redjeb, W. H. Zhou, H. LeVine III, B. Török and M. Török, Bioorg. Med. Chem. Lett., 2015, 25, 626–630 CrossRef CAS PubMed.
- R. R. Wilkening, R. W. Ratcliffe, K. J. Wildonger, L. D. Cama, K. D. Dykstra, F. P. DiNinno, T. A. Blizzard, M. L. Hammond, J. V. Heck, K. L. Dorso, E. St Rose, J. Kohler and G. G. Hammond, Bioorg. Med. Chem. Lett., 1999, 9, 673–678 CrossRef CAS PubMed.
-
(a) Y. L. Xie, G. L. Gong, Y. D. Liu, S. M. Deng, A. Rinderspacher, L. Branden and D. W. Landry, Tetrahedron Lett., 2008, 49, 2320–2323 CrossRef CAS;
(b) Y. L. Xie, S. X. Deng, C. J. Thomas, Y. D. Liu, Y. Q. Zhang, A. Rinderspacher, W. W. Huang, G. L. Gong, M. Wyler, E. Cayanis, N. Aulner, U. Tobben, C. Chung, S. Pampou, N. Southall, D. Vidovic, S. Schurer, L. Branden, R. E. Davis, L. M. Staudt, J. Inglese, C. P. Austin, D. W. Landry, D. H. Smith and D. S. Auld, Bioorg. Med. Chem. Lett., 2008, 18, 329–335 CrossRef CAS PubMed.
-
(a) C. B. Bheeter, J. K. Bera and H. Doucet, Adv. Synth. Catal., 2012, 354, 3533–3538 CrossRef CAS;
(b) D. K. Rayabarapu, A. Zhou, K. O. Jeon, T. Samarakoon, A. Rolfe, H. Siddiqui and P. R. Hanson, Tetrahedron, 2009, 65, 3180–3188 CrossRef CAS PubMed;
(c) S. Rousseaux, S. I. Gorelsky, B. K. W. Chung and K. Fagnou, J. Am.
Chem. Soc., 2010, 132, 10692–10705 CAS;
(d) N. Conde, F. Churruca, R. SanMartin, M. T. Herrero and E. Dominguez, Adv. Synth. Catal., 2015, 357, 1525–1531 CrossRef CAS;
(e) Z. J. Liu and R. C. Larock, Tetrahedron, 2007, 63, 347–355 CrossRef CAS PubMed.
- J. K. Laha, K. P. Jethava and N. Dayal, J. Org. Chem., 2014, 79, 8010–8019 CrossRef CAS PubMed.
-
(a) Y. W. Li, Q. P. Ding, G. Y. S. Qiu and J. Wu, Org. Biomol. Chem., 2014, 12, 149–155 RSC;
(b) S. A. Glover, A. Goosen, C. W. Mccleland and J. L. Schoonraad, J. Chem. Soc., Perkin Trans. 2, 1986, 645–653 RSC;
(c) P. S. Dewar, A. R. Forrester and R. H. Thomson, J. Chem. Soc., Perkin Trans. 1, 1972, 2862–2865 RSC.
- For selected reviews of visible-light photoredox catalysis, see:
(a) C. K. Prier, D. A. Rankic and D. W. C. MacMillan, Chem. Rev., 2013, 113, 5322 CrossRef CAS PubMed;
(b) Y. Xi, H. Yi and A. Lei, Org. Biomol. Chem., 2013, 11, 2387 RSC;
(c) J. Xuan and W. J. Xiao, Angew. Chem., Int. Ed., 2012, 51, 6828 CrossRef CAS PubMed;
(d) J. M. R. Narayanam and C. R. J. Stephenson, Chem. Soc. Rev., 2011, 40, 102 RSC;
(e) T. P. Yoon, M. A. Ischay and J. Du, Nat. Chem., 2010, 2, 527 CrossRef CAS PubMed;
(f) K. Zeitler, Angew. Chem., Int. Ed., 2009, 48, 9785 CrossRef CAS PubMed.
- H. Wang and S. Yu, Org. Lett., 2015, 17, 4272–4275 CrossRef CAS PubMed.
-
(a) T. Miura, Y. Nishida, M. Morimoto, M. Yamauchi and M. Murakami, Org. Lett., 2011, 13, 1429–1431 CrossRef CAS PubMed;
(b) T. Miura, M. Yamauchi, A. Kosaka and M. Murakami, Angew. Chem., Int. Ed., 2010, 49, 4955–4957 CrossRef CAS PubMed.
- This reaction could go to completion with 99% yield when the reaction time was prolonged to 14 h.
-
(a) Z. H. Xia, J. B. Huang, Y. M. He, J. J. Zhao, J. Lei and Q. Zhu, Org. Lett., 2014, 16, 2546–2549 CrossRef CAS PubMed;
(b) G. J. Wu, Y. F. Deng, C. Q. Wu, Y. Zhang and J. B. Wang, Angew. Chem., Int. Ed., 2014, 53, 10510–10514 CrossRef CAS PubMed;
(c) D. P. Hari, P. Schroll and B. König, J. Am. Chem. Soc., 2012, 134, 2958–2961 CrossRef CAS PubMed;
(d) T. Hering, D. P. Hari and B. König, J. Org. Chem., 2012, 77, 10347–10352 CrossRef CAS PubMed;
(e) T. B. Xiao, X. C. Dong, Y. C. Tang and L. Zhou, Adv. Synth. Catal., 2012, 354, 3195–3199 CrossRef CAS;
(f) Y. Liu, J. L. Zhang, R. J. Song and J. H. Li, Eur. J. Org. Chem., 2014, 1177–1181 CrossRef CAS;
(g) H. Canoyelo and A. Deronzier, J. Chem. Soc., Perkin Trans. 2, 1984, 1093–1098 RSC.
Footnote |
† Electronic supplementary information (ESI) available. See DOI: 10.1039/c6qo00116e |
|
This journal is © the Partner Organisations 2016 |
Click here to see how this site uses Cookies. View our privacy policy here.