DOI:
10.1039/C6QO00164E
(Highlight)
Org. Chem. Front., 2016,
3, 1004-1010
New entries toward the synthesis of OCF3-containing molecules
Received
18th April 2016
, Accepted 5th June 2016
First published on 8th June 2016
Abstract
The OCF3 group is of high interest in the quest for new drugs and agrochemicals, thanks to its atypical features. This Highlight article depicts the recent and impressive advances toward the synthesis of OCF3-containing molecules.
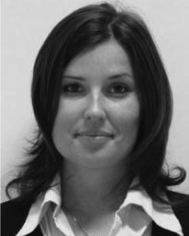 Tatiana Besset | Dr Tatiana Besset was educated in chemistry at the University of Grenoble (France) in the group of Dr Andrew E. Greene, where she obtained her doctoral degree in 2009. She then moved to the Westfälische Wilhelms Universität Münster (Germany) as a postdoctoral fellow in the group of Prof. Frank Glorius (Rh C–H bond activation). In 2011, she joined the group of Prof. Joost N. H. Reek at the University of Amsterdam (the Netherlands), as a postdoctoral fellow in collaboration with the Eastman company where she was working on supramolecular encapsulated rhodium catalysts for branched selective hydroformylation of alkenes. Since October 2012, she is working as a CNRS Researcher (CR2, Chargée de Recherche CNRS) in the “Fluorinated Biomolecules Synthesis” team at INSA Rouen, France. Her research focuses on the transition metal-catalyzed C–H bond functionalization and the development of new strategies for the synthesis of fluorinated building blocks. |
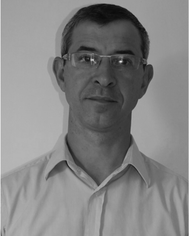 Philippe Jubault | Philippe Jubault received his Diploma in Engineering in “Chemistry and Process” from the National Institute of Applied Sciences of Rouen. In 1992, he joined the research group of Pr. Collignon and Pr. C. Feasson at the INSA of Rouen where he obtained his Ph.D. in January 1996. After one year as a post-doctoral student at Hydro-Québec (Shawinigan, Québec, Canada), he was a Marie Curie research fellow in the laboratory of Pr. V. K. Aggarwal at the University of Sheffield. In 1998, he returned to the INSA of Rouen and assumed an assistant professor position in 2000, becoming a full professor in 2008. His research interests include the development of new methodologies for the synthesis of original fluorinated building blocks bearing CF2 and SF5 groups, new methods for the synthesis of fluorocyclopropanes and the development of new heterocyclic scaffolds for medicinal chemistry. |
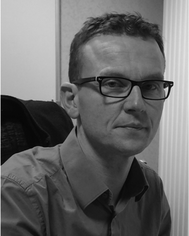 Xavier Pannecoucke | Prof. Xavier Pannecoucke was born in Lille (France) in 1967. He got his Ph.D. in BioOrganic Chemistry in 1993 from Louis Pasteur University of Strasbourg (France), under the supervision of Professors Guy Ourisson and Bang Luu. He then moved to Japan for one year to carry out his first postdoctoral studies in the laboratory of Prof. Koichi Narasaka at the University of Tokyo. He moved again to Great Britain for fourteen months to carry out his second postdoctoral studies in the laboratory of Prof. Steven V. Ley at the University of Cambridge. He then went back to France as a Maître de Conférences at the University of Rouen in the group of Prof. J.-C. Quirion. In 2003, he took the position of Professor at the INSA of Rouen, leading the group of “Fluorinated Biomolecules Synthesis” and heading COBRA laboratory from 2011 (UMR 6014). His main research interest is dealing with development of new methodologies to access fluorinated biomolecules (peptidomimetics, carbohydrates, nucleosides, heterocycles, etc.). |
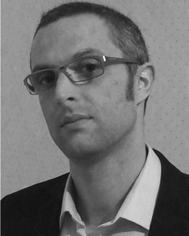 Thomas Poisson | Dr Thomas Poisson received his Ph.D. in 2008 from the University of Rouen under the mentorship of Dr Vincent Levacher, working on the development of new catalytic enantioselective protonation reactions. He then joined the group of Prof. Shū Kobayashi as a JSPS postdoctoral fellow working on asymmetric catalysis by using alkaline earth metal complexes (2008–2010, Tokyo University). In 2010, he joined the group of Prof. Magnus Rueping (RWTH-Aachen) as a postdoctoral fellow working on the design of new photocatalyzed reactions. In September 2011, he was appointed as an Assistant Professor at INSA-Rouen, within the group of “Fluorinated Biomolecules Synthesis” and defended his habilitation in 2015. The same year, he was elected Distinguished Junior Fellow of the French Chemical Society (SCF) and received the “prix jeune enseignant-chercheur” (young lecturer prize) in 2016 from the Organic Chemistry Division of the French Chemical Society (SCF). |
Introduction
Over the last five years, a resurgence of interest regarding organofluorine chemistry has been witnessed and this research area is currently blossoming.1 This tremendous expansion results from the importance of the fluorine atom in the quest for pharmaceuticals and agrochemicals. Indeed, this intriguing atom, thanks to its intrinsic properties, strongly affects the physical and biological properties of a molecule.2 As a matter of consequence, more than 20% of pharmaceuticals and 30% of agrochemicals bear at least one fluorine atom.3
Although strong efforts were dedicated to the design of new methodologies to introduce a fluorine atom or a CF3 group,4 little attention was paid to the introduction of other fluorinated groups. Only very recently, the organic chemist community has been interested in the installation of the difluoromethylated functional groups5 and the SCF3 motif,6 for instance. In comparison with the SCF3 group, for which a large panel of methodologies has been reported,7 less effort was devoted to develop new tools for the introduction of its oxygenated congener, namely the trifluoromethoxy group (OCF3).8 This statement is in sharp contrast with the impressive properties of this motif, which is nowadays widely used in the drugs and agrochemicals discovery process. Indeed, the OCF3 group increased the lipophilicity of the molecule compared to the OCH3 and CF3 groups and has a slightly lower one compared to the SCF3 group (OCF3: π = 1.04; CF3: π = 0.88; OCH3: π = −0.20; SCF3: π = 1.44).9 In addition, this substituent could provide a particular conformation to a molecule due to its specific features. From an electronic point of view, this functional group is not conjugated to an arene since the p-electrons of the oxygen atom are delocalized in the σ*-orbitals of the adjacent C–F bonds.10 Moreover, to reduce the electronic repulsions, the CF3 group rotates out of the nucleus plane and the OCF3 group connected to an arene adopts an orthogonal orientation with respect to the arene residue.11 All these interesting features confer to molecules bearing the OCF3 substituent, a special conformation and an enhanced lipophilicity. Additionally, it is worth mentioning that this motif is thermally and chemically stable toward acids, bases, reductants, oxidants and organometallic species for instance.12
Therefore, this is not surprising to find a plethora of molecules bearing that motif toward the discovery of drugs and agrochemicals. For instance Riluzole®, a drug marketed by Sanofi against amyotrophic lateral sclerosis, is available (Fig. 1). Moreover, numerous agrochemicals are bearing this motif as Flucarbazone and Triflumuron. These examples clearly highlight the high interest for this motif, which is well recognized as an important emerging fluorinated group.
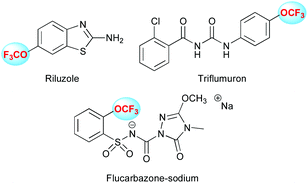 |
| Fig. 1 OCF3-containing pharmaceuticals and agrochemicals. | |
Although this fluorinated group is extremely attractive, no practical and general methodology to introduce this motif has been depicted so far. In this Highlight article, we will showcase the recent and impressive advances made to build up OCF3-containing molecules.
Historical background
From a historical point of view, Yagupolskii synthesized the first aryl–OCF3 molecules by a chlorine–fluorine exchange on the trichloroanisole obtained by chlorination of the anisole (Scheme 1, path a).13 This strategy has been widely used to prepare the trifluoromethoxyarenes. Prior to 2010, a few other approaches were depicted. The fluorination of fluoroformates (Scheme 1, path b),12a as well as the oxidative desulfurization/fluorination (Scheme 1, path c) were used to produce OCF3-containing arenes.14 It is worth mentioning that all these processes required the use of either toxic or highly corrosive reagents, which strongly hampered their synthetic utility. Finally, electrophilic trifluoromethylation of phenols was an alternative to the other methods, even though the employed electrophilic CF3 source is tricky to access (Scheme 1, path d).15
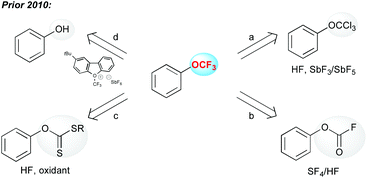 |
| Scheme 1 Synthetic methods to access OCF3-arenes prior to 2010. | |
In the following sections, the major recent progress made to functionalize OH-containing molecules with a CF3 group (O–CF3 bond formation) or to introduce the OCF3 group (C–OCF3 bond formation) onto a molecule in a straightforward fashion is depicted.
Construction of the O–CF3 bond
In line with the pioneering work reported prior to 2010 and in analogy with the construction of an O–CH3 bond, a logical retrosynthetic disconnection to access OCF3-containing molecules relied on the formation of the O–CF3 bond.
Electrophilic trifluoromethylation reaction
Togni and co-workers have reported the electrophilic trifluoromethylation of alcohols by using Togni's reagent II (1-trifluoromethyl-1,2-benziodoxol-3(1H)-one) and Zn(NTf)2 as a promoter.16 The reported methodology afforded aliphatic OCF3 derivatives smoothly. However, a major limitation was the trifluoromethylation of phenol derivatives, which predominantly provided the product resulting from a trifluoromethylation reaction at the carbon atom of the aromatic ring.17 Very recently, the group of Zou, Wu and Wu applied this strategy to the electrophilic O-trifluoromethylation of 2-pyridones, 2-quinolones and other N-heterocyclic derivatives in moderate to good yields using the Togni's reagent II (Scheme 2).18 To explain the reaction outcome, the authors proposed a radical pathway. After a proton exchange between 2-hydroxypyridine and II, the new ion pair A was formed. Then a single electron transfer (SET) provided radicals B and C, which after recombination formed the O–CF3 bond. This reaction, although restricted in terms of substrates, afforded the first general access to OCF3-containing N-heterocycles without using metals or harsh conditions.
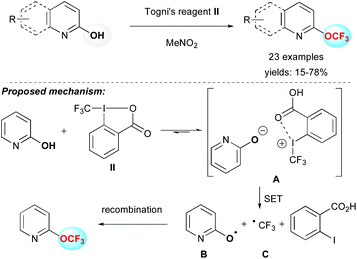 |
| Scheme 2 Synthesis of OCF3-containing N-heterocycles. | |
Oxidative trifluoromethylation reaction
Despite the undeniable interest for the above-mentioned approach, the requirement of an electrophilic CF3 source, which requires extra synthetic steps, is a considerable limitation.
Indeed, an alternative pathway to synthesize trifluoromethyl ethers relied on the use of the readily available and inexpensive Ruppert–Prakash reagent (TMSCF3) with phenols in the presence of an oxidant. In 2015, Qing and co-workers published the selective O-trifluoromethylation of phenols by means of a silver-mediated oxidative process at room temperature (Scheme 3). The presence of exogenous oxidants (Selectfluor and NFSI) turned out to have a beneficial effect on the outcome of the transformation. A panel of aryl trifluoromethylethers were efficiently prepared and the reaction proved to be tolerant to various functional groups. Note that heterocycles and electron-rich phenol derivatives were suitable substrates.19a This strategy was successfully extended to the functionalization of aliphatic alcohols using slightly modified conditions. This efficient methodology allowed the functionalization of primary, secondary and even bulkier tertiary alcohols at room temperature (Scheme 3).19b To explain the reaction outcome, a plausible mechanism was proposed. In the presence of AgOTf, an activator (CsF or KF) and 2-fluoropyridine, TMSCF3 would be converted into the Ag(I)CF3 species I. The latter species would lead to the silver intermediate II in a high oxidation state ([Ag(III)(CF3)(F)]), in the presence of exogenous oxidants (Selectfluor and/or NFSI). Subsequent ligand exchange with an alkoxide (species III) followed by a reductive elimination would afford the expected product.
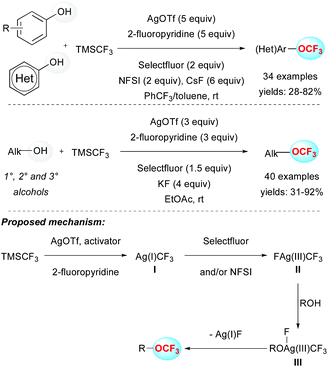 |
| Scheme 3 Formation of the O–CF3 bond through an oxidative trifluoromethylation reaction. | |
Formation of the C–OCF3 bond
Alternatively to these methods, a second retrosynthetic approach to build up OCF3-containing molecules focused on the formation of a C–OCF3 bond.
Trifluoromethoxylation reaction promoted by transition metal
In 2011, Ritter and co-workers reported a real breakthrough in the field by depicting the first efficient method to introduce the OCF3 group on aryl stannanes or aryl boronic acids in good yields (Scheme 4).20 The methodology was applied to several arene and heteroarene derivatives as well as challenging substrates like amino-acid, steroid or morphine derivatives. From a mechanistic point of view, the authors proposed the formation of a bimetallic silver complex, followed by the formation of a discrete high-valent silver complex resulting from oxidation with F-TEDA-PF6.
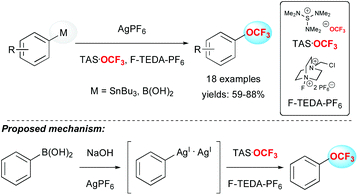 |
| Scheme 4 Introduction of the OCF3 group onto aryl stannanes and boronic acids. | |
In 2015, Liu and co-workers reported the first palladium-catalysed intramolecular aminotrifluoromethoxylation of unactivated alkenes using AgOCF3 in the presence of Selectfluor. A panel of 3-trifluoromethoxylated-5,5-disubstituted piperidines were synthesized in moderate to good yields (Scheme 5).21
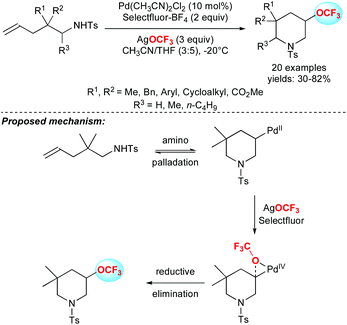 |
| Scheme 5 Palladium-catalysed aminotrifluoromethoxylation of alkenes. | |
The authors investigated the mechanism and demonstrated the involvement of a Pd(IV) species. This reactive intermediate rather favoured the preferential reductive elimination pathway over the deleterious β-fluoride elimination.
Nucleophilic trifluoromethoxylation reaction
An alternative reaction pathway to build up OCF3-containing molecules is the direct introduction of the OCF3 motif, thanks to a nucleophilic addition of the corresponding alkoxide. As a readily available source, silver trifluoromethoxide (AgOCF3) appeared as a good candidate to perform this reaction.22
The unique properties of bis(arylimino)acenaphthene derivatives (aryl-BIAN) have been used for the synthesis of a nucleophilic silver(I)trifluoromethoxide complex (Scheme 6).23 This complex reacted efficiently with organic benzyl bromides at room temperature leading to benzyl trifluoromethyl ethers in good to excellent yields. Diverse functional groups such as methoxy, nitro, nitrile, halide and heterocycles were well tolerated.
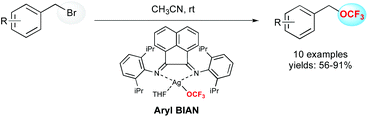 |
| Scheme 6 Nucleophilic addition of the OCF3 group on benzyl halides. | |
Very recently, the group of Qin and Zhang reported the nucleophilic trifluoromethoxylation of α-diazo ester derivatives (Scheme 7).24
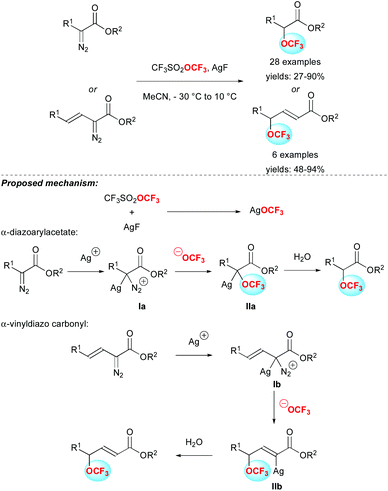 |
| Scheme 7 Nucleophilic trifluoromethoxylation of α-diazo esters. | |
The reaction of α-diazoarylacetates in the presence of AgF and CF3SO2OCF3 (TFMT) allowed easy access to α-trifluoromethoxylated esters in moderate to excellent yields. Various α-diazo-carbonyl compounds were successfully used, bearing either substituted phenyl rings or heteroaryls. Interestingly, α-diazo vinyl carbonyl derivatives furnished the corresponding γ-trifluoromethoxyl α,β-unsaturated esters. Regarding the reaction mechanism, the authors assumed the in situ formation of AgOCF3, resulting from the reaction of AgF with CF3SO2OCF3, as the initial step. Then, the Ag+ cation reacted with the diazo compounds to form intermediate I. Then, the trifluoromethoxy anion underwent a nucleophilic addition onto I to produce silver species II. A final protonation step afforded the desired product. In the case of the α-diazo vinyl carbonyl derivative, a vinylogous process is involved in the formation of the γ-trifluoromethoxyl α,β-unsaturated ester.
OCF3-migration reaction
In the course of the development of trifluoromethoxylation reagents, Ngai and co-workers reported a thermally induced OCF3 migration to generate synthetically useful ortho-trifluoromethoxylated aniline derivatives (Scheme 8).25a In this two-step sequence, protected N-aryl-N-hydroxyl amines were reacted with Togni's reagent II leading to O-trifluoromethylated adducts. Then, a thermally induced OCF3 migration occurred to form the C–OCF3 bond. This reaction tolerated a wide range of functional groups including nitrile, ketone, amide, halogens, ether and ester. Interestingly, a one-pot reaction was successfully developed, in which NaH was used as a base and the migration operated at room temperature. Finally, a gram scale synthesis demonstrated the practicability and effectiveness of this transformation.
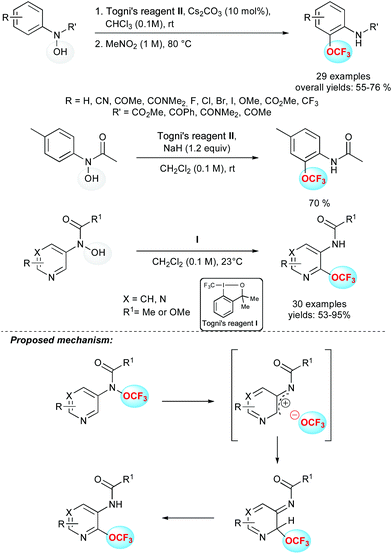 |
| Scheme 8 Synthesis of OCF3-containing molecules through an OCF3-migration. | |
This protocol has been extended to heteroaromatic substrates such as pyridines and pyrimidines.25b,c In these cases, the Togni's reagent I proved to be the most efficient and the migration operated efficiently at room temperature with substrates bearing an electron donating substituent at the para position, with respect to the acetanilide group. In the absence of such substituents, higher reaction temperatures were required. Again, a wide range of functional groups and substitution patterns were tolerated. This methodology was successfully applied to pyridines and pyrimidines bearing a wide array of heteroaryl substituents such as furan, pyrazole and benzimidazole for example. Concerning the mechanism of this trifluoromethoxylation reaction,25d experimental data supported the O-trifluoromethylation of the N-(heteroaryl)-N-hydroxylamine derivatives through a radical process, whereas the OCF3-migration step proceeded via a heterolytic cleavage of the N–OCF3 bond followed by a rapid recombination of a short-lived ion pair. Computational studies supported the proposed ion pair pathway for the OCF3-migration process.
Conclusion
In conclusion, we summarized herein, the recent progress that has been achieved toward the synthesis of OCF3-containing molecules. This Highlight article points out the efforts devoted to the development of new methodologies to build up these key molecules. However, despite this endeavour, the synthesis of OCF3-containing molecules remains in its infancy since no general method has been reported to date. Indeed, the quest for a scalable, practical and general method still appears today as a “holy grail”. In addition, the lack of safe, practical and readily available reagents still hampers the development of efficient synthetic tools. Beyond doubt, the search of original reagents as well as new transformations will continue to stimulate the creativity of the chemists to afford straightforward access to OCF3-containing molecules.
Acknowledgements
This work was partially supported by INSA Rouen, Rouen University, CNRS, EFRD, Labex SynOrg (ANR-11-LABX-0029) and Région Haute-Normandie (Crunch Network).
Notes and references
- For review, see:
(a) S. Purser, P. R. Moore, S. Swallow and V. Gouverneur, Chem. Soc. Rev., 2008, 37, 320–330 RSC
;
(b) H. Egami and M. Sodeoka, Angew. Chem., Int. Ed., 2014, 53, 8294–8308 CrossRef CAS PubMed
. For a special issue on fluorine chemistry, see: Chem. Rev., 2015, 115, 563–1306.
- D. O'Hagan, Chem. Soc. Rev., 2008, 37, 308–319 RSC
.
-
(a) J. Wang, M. Sánchez-Roselló, J. L. Aceña, C. del Pozo, A. E. Sorochinsky, S. Fustero, V. A. Soloshonok and H. Liu, Chem. Rev., 2014, 114, 2432–2506 CrossRef CAS PubMed
;
(b) E. A. Ilardi, E. Vitaku and J. T. Njardarson, J. Med. Chem., 2014, 57, 2832–2842 CrossRef CAS PubMed
.
-
(a) T. Furuya, A. S. Kamlet and T. Ritter, Nature, 2011, 473, 470–477 CrossRef CAS PubMed
;
(b) T. Liang, C. N. Neumann and T. Ritter, Angew. Chem., Int. Ed., 2013, 52, 8214–8264 CrossRef CAS PubMed
;
(c) T. Besset, C. Schneider and D. Cahard, Angew. Chem., Int. Ed., 2012, 51, 5048–5050 CrossRef CAS PubMed
;
(d) H. Liu, Z. Gu and X. Jiang, Adv. Synth. Catal., 2013, 355, 617–626 CrossRef CAS
;
(e) X.-F. Wu, H. Neumann and M. Beller, Chem. – Asian J., 2012, 7, 1744–1754 CrossRef CAS PubMed
.
-
(a) T. Besset, T. Poisson and X. Pannecoucke, Chem. – Eur. J., 2014, 20, 16830–16845 CrossRef CAS PubMed
;
(b) M.-C. Belhomme, T. Besset, T. Poisson and X. Pannecoucke, Chem. – Eur. J., 2015, 21, 12836–12865 CrossRef CAS PubMed
;
(c) C. Ni, L. Zhu and J. Hu, Acta Chim. Sin., 2015, 73, 90–115 CrossRef CAS
.
- F. Toulgoat, S. Alazet and T. Billard, Eur. J. Org. Chem., 2014, 2415–2428 CrossRef CAS
.
- For selected examples, see:
(a) J.-B. Liu, X.-H. Xu, Z.-H. Chen and F.-L. Qing, Angew. Chem., Int. Ed., 2015, 54, 897–900 CrossRef CAS PubMed
;
(b) H.-Y. Xiong, T. Besset, D. Cahard and X. Pannecoucke, J. Org. Chem., 2015, 80, 4204–4212 CrossRef CAS PubMed
;
(c) G. Danoun, B. Bayarmagnai, M. F. Gruenberg and L. J. Gooßen, Chem. Sci., 2014, 5, 1312–1316 RSC
;
(d) R. Pluta, P. Nikolaienko and M. Rueping, Angew. Chem., Int. Ed., 2014, 53, 1650–1653 CrossRef CAS PubMed
;
(e) M. Hu, J. Rong, W. Miao, C. Ni, Y. Han and J. Hu, Org. Lett., 2014, 16, 2030–2033 CrossRef CAS PubMed
;
(f) H.-Y. Xiong, X. Pannecoucke and T. Besset, Org. Chem. Front., 2016, 3, 620–624 RSC
.
- For excellent reviews, see:
(a) G. Landelle, A. Panossian, S. Pazenok, J.-P. Vors and F. R. Leroux, Beilstein J. Org. Chem., 2013, 9, 2476–2536 CrossRef PubMed
;
(b) G. Landelle, A. Panossian and F. R. Leroux, Curr. Top. Med. Chem., 2014, 14, 941–951 CrossRef CAS PubMed
;
(c) B. Manteau, S. Pazenok, J. P. Vors and F. R. Leroux, J. Fluorine Chem., 2010, 131, 140–158 CrossRef CAS
;
(d) F. R. Leroux, B. Manteau, J.-P. Vors and S. Pazenok, Beilstein J. Org. Chem., 2008, 4, 13 Search PubMed
;
(e) F. Leroux, P. Jeschke and M. Schlosser, Chem. Rev., 2005, 105, 827–856 CrossRef CAS PubMed
.
-
(a) C. Hansch, A. Leo, S. H. Unger and K. H. Kim, J. Med. Chem., 1973, 16, 1207–1216 CrossRef CAS PubMed
;
(b) A. Leo, P. Jow, C. Silipo and C. Hansch, J. Med. Chem., 1975, 18, 865–868 CrossRef CAS PubMed
.
- O. Marrec, T. Billard, J.-P. Vors, S. Pazenok and B. R. Langlois, J. Fluorine Chem., 2010, 131, 200–207 CrossRef CAS
.
- B. Manteau, P. Genix, L. Brelot, J.-P. Vors, S. Pazenok, F. Giornal, C. Leuenberger and F. R. Leroux, Eur. J. Org. Chem., 2010, 6043–6066 CrossRef CAS
.
-
(a) W. A. Sheppard, J. Org. Chem., 1964, 29, 1–11 CrossRef CAS
;
(b) P. E. Aldrich and W. A. Sheppard, J. Org. Chem., 1964, 29, 11–15 CrossRef CAS
.
-
(a) L. M. Yagupolskii, Dokl. Akad. Nauk. SSSR, 1955, 105, 100–102 CAS
;
(b) F. Mathey and J. Bensoam, Tetrahedron Lett., 1973, 25, 2253–2256 CrossRef
;
(c) A. E. Feiring, J. Org. Chem., 1979, 44, 2907–2910 CrossRef CAS
.
-
(a) M. Kuroboshi, K. Suzuki and T. Hiyama, Tetrahedron Lett., 1992, 33, 4173–4176 CrossRef CAS
;
(b) K. Kanie, Y. Tanaka, K. Suzuki, M. Kuruboshi and T. Hiyama, Bull. Chem. Soc. Jpn., 2000, 73, 471–484 CrossRef CAS
;
(c) M. Kuroboshi, K. Kanie and T. Hiyama, Adv. Synth. Catal., 2001, 343, 235–250 CrossRef CAS
;
(d) M. Shimizu and T. Hiyama, Angew. Chem., Int. Ed., 2005, 44, 214–231 CrossRef CAS PubMed
.
- T. Umemoto, K. Adachi and S. Ishihara, J. Org. Chem., 2007, 72, 6905–6917 CrossRef CAS PubMed
.
- R. Koller, K. Stanek, D. Stolz, R. Aardoom, K. Niedermann and A. Togni, Angew. Chem., Int. Ed., 2009, 48, 4332–4336 CrossRef CAS PubMed
.
- K. Stanek, R. Koller and A. Togni, J. Org. Chem., 2008, 73, 7678–7685 CrossRef CAS PubMed
.
- A. Liang, S. Han, Z. Liu, L. Wang, J. Li, D. Zou, Y. Wu and Y. Wu, Chem. – Eur. J., 2016, 22, 5102–5106 CrossRef CAS PubMed
.
-
(a) J.-B. Liu, C. Chen, L. Chu, Z.-H. Chen, X.-H. Xu and F.-L. Qing, Angew. Chem., Int. Ed., 2015, 54, 11839–11842 CrossRef CAS PubMed
;
(b) J.-B. Liu, X.-H. Xu and F.-L. Qing, Org. Lett., 2015, 17, 5048–5051 CrossRef CAS PubMed
.
- C. Huang, T. Liang, S. Harada, E. Lee and T. Ritter, J. Am. Chem. Soc., 2011, 133, 13308–13310 CrossRef CAS PubMed
.
- C. Chen, P. Chen and G. Liu, J. Am. Chem. Soc., 2015, 137, 15648–15651 CrossRef CAS PubMed
.
- For an early study, see: O. Marrec, T. Billard, J.-P. Vors, S. Pazenok and B. R. Langlois, Adv. Synth. Catal., 2010, 352, 2831–2837 CrossRef CAS
; and ref. 10.
- S. Chen, Y. Huang, X. Fang, H. Li, Z. Zhang, T. S. Andy Hor and Z. Weng, Dalton Trans., 2015, 44, 19682–19686 RSC
.
- G.-F. Zha, J.-B. Han, X.-Q. Hu, H.-L. Qin, W.-Y. Fang and C.-P. Zhang, Chem. Commun., 2016, 52, 7458–7461 RSC
.
-
(a) K. N. Hojczyk, P. Feng, C. Zhan and M.-Y. Ngai, Angew. Chem., Int. Ed., 2014, 53, 14559–14563 CrossRef CAS PubMed
;
(b) P. Feng, K. N. Lee, J. W. Lee, C. Zhan and M.-Y. Ngai, Chem. Sci., 2015, 7, 424–429 RSC
;
(c) K. Lee, J. Lee and M.-Y. Ngai, Synlett, 2016, 313–319 CAS
;
(d) K. N. Lee, Z. Lei, C. A. Morales-Rivera, P. Liu and M.-Y. Ngai, Org. Biomol. Chem., 2016 10.1039/c6ob00132g
.
|
This journal is © the Partner Organisations 2016 |
Click here to see how this site uses Cookies. View our privacy policy here.