DOI:
10.1039/C5RA23146A
(Paper)
RSC Adv., 2016,
6, 1243-1252
Rapid and selective extraction of Pd(II) ions using the SCS type pincer ligand 1,3-bis(dimethylthiocarbamoyloxy)benzene, and its Pd(II) extraction mechanism†
Received
4th November 2015
, Accepted 7th December 2015
First published on 9th December 2015
Abstract
We report the salient metal complexation properties of the SCS pincer ligand 1,3-bis(dimethylthiocarbamoyloxy)benzene (1), and its Pd(II) extraction properties in liquid–liquid extraction. Rapid and selective extraction by 1 of >99% Pd(II) ions from an automotive catalyst leach liquor containing Rh, Pd, Pt, Zr, Ce, Ba, Al, La, and Y in Cl− media was observed. The extractability (E%) of 1 toward Pd was higher than that of the analogous compounds 1,4-bis(dimethylthiocarbamoyloxy)benzene (2), 1,4-bis(dimethylthiocarbamoyloxy)2,5-di-tert-butylbenzene (3), and 1,2-bis(dimethylthiocarbamoyloxy)benzene (4), and the commercial Pd(II) extractant di-n-hexyl sulfide (DHS). In o-dichlorobenzene diluent, 1 showed a high E%Pd (99.9–97.0%) in Cl− media (0.1–8.0 M) in 30 min, and excellent Pd(II) extraction and good phase separation were obtained with the various diluents studied. Pd(II) ions were stripped from the extractants using 0.1 M thiourea in 1.0 M HCl, enabling the reuse of 1. Loading capacity measurements revealed a Pd/extractant 1 ratio of 1. Palladation is promoted at the benzene ring and dimethylthiocarbamoyl moieties via formation of stable fused six-membered chelate rings. The extractant 1–Pd(II) complex was characterized using FT-IR spectroscopy, NMR spectroscopy, elemental analysis, single-crystal XRD study, and MALDI-TOF MS analysis. Extractant 1 displayed a high E%Pd (99%) even after five extraction cycles, indicating that it is very stable in acidic media and may be useful for the rapid and selective recovery of Pd(II) from catalyst solutions in platinum group metal refineries.
Introduction
Much of the total global production (2010) of platinum group metals (PGMs) (ca. 46% of Pt, ca. 57% of Pd, and ca. 77% of Rh) is consumed in the automobile catalytic converter industry.1 During the process of fuel burning inside an automotive engine, several gaseous components are released. These gaseous components include NOx, light hydrocarbons, and CO, which are all dangerous gases that are harmful both to human health and the environment.2 In order to reduce the emission levels of these species, precious metals such as Pt, Pd, and Rh (among other co-catalytic metals) are used for manufacturing catalytic converters for cars and other motor vehicles.3 After prolonged use, their catalytic ability is exhausted, and they become waste materials.3 The amounts of PGMs that can be recovered from a catalytic converter can range from 1–2 g for small cars to 12–15 g for large trucks.4 This is significantly higher than the PGM content of primary ores (on average < 0.01 g kg−1) with various possible combinations of PGMs.5 Recovery of PGMs from spent automotive catalysts, spent industrial catalysts, electronic scrap, etc. is very important for reuse.2
In a standard process, PGMs from spent automotive catalytic converters are dissolved in concentrated chloride media, forming chloro-complexes in solution.6 In general, Pd(II) is recovered using Aloxime®840, di-n-hexyl-sulfide, di-n-octyl-sulfide, di(2-ethylhexyl)thiophosphoric acid, Cyanex®471X, or Acorga®CLX-50.7 However, the commercial extractants for PGM recovery are not very effective, and mutual separations are not completed.7a,8 Their Pd(II) extraction is extremely slow, and deterioration and structural changes are possible with prolonged usage.7a,8a,9 For example, a general risk when dealing with sulfide-based extractants is the possibility of oxidation of the sulfur atom, especially by contact of the acidic leached catalyst solution with an oxidizing aqueous phase.10,11
In order to resolve the aforementioned problems, many researchers have investigated new types of sulfur-based extractants for Pd(II) recovery.12–14 Recently, we have developed many novel thiocarbamoyl- and phosphono-functionalized thiacalix[n]arenes as extractants for selective recovery of Pd from automotive catalyst residue.15 However, these newly developed extractants have drawbacks such as multistep synthesis procedures, the requirement of column chromatography for purification, problems with solubility, and co-extraction of minute amounts of other PGMs.12,15 Therefore, development of cheap extractants that show high stability in acidic media and can effectively and selectively separate specific PGMs is needed.
A pincer ligand is a type of chelating ligand that binds tightly to three adjacent coplanar sites with transition metals. Pincer complexes generally have a tridentate ligand composed of a cyclometalating σ carbon and donating groups, such as phosphorus-, nitrogen-, and sulfur-containing groups.16 This tridentate ligand is connected to the metal through at least one metal–carbon σ bond.16 Pincer type ligands are widely used in catalysis and coordination chemistry.17 Pincer organometallics with thioamide ligands are utilized in many applications, such as catalysts in organic cross-coupling reactions, tunable redox-potential complexes, luminescence materials, and chemical-sensor materials.18
Herein, we present the first report of the intriguing extraction properties of the thioamide-based SCS-pincer ligand, 1,3-bis(dimethylthiocarbamoyloxy)benzene (1), for selective Pd(II) extraction from a leach liquor of an automotive catalyst. This species was synthesized by Kurth et al.19 (1973) as an intermediate in the preparation of 1,3-benzenedithiol via a Newman–Kwart rearrangement. We have studied its solvent extraction properties and metal–pincer complex structure. In addition, we also prepared various similar thioamide derivatives; 1,4-bis(dimethylthiocarbamoyloxy)benzene (2), 1,4-bis(dimethylthiocarbamoyloxy)2,5-di-tert-butylbenzene (3), and 1,2-bis(dimethylthiocarbamoyloxy)benzene (4); for Pd(II) extraction, and compared their extraction capabilities with that of the pincer ligand (1). All extractants (1–4) can be easily synthesized with high yield in one step from commercially available reagents. Each could be isolated by recrystallization without column chromatography. Owing to the high yields and simple workup procedures, these extractants can be conveniently prepared at low cost on scales ranging from the gram to kilogram level. The extraction mechanism of 1 is quite different from those of Pd extractants reported in the literature. Compared with the other extractants prepared and commercial available extractants, the prepared extractant 1 displays low cost and rapid extraction of Pd(II) from a leach liquor of an automotive catalyst. We have observed many intriguing properties of 1 from the liquid–liquid extraction:
(1) 1 extracts Pd(II) in various diluents, and shows good extraction in HCl media (0.1–8.0 M) compared with the commercial extractant dihexyl sulfide (DHS).
(2) 1 exhibits rapid and efficient Pd(II) extraction even in very highly acidic media.
(3) 1 is very stable in acidic media, and can be used for the recovery of Pd(II) from catalyst solutions in PGM refineries.
(4) 1 exhibits a high loading capacity under the required Cl− media and a short equilibrium time.
(5) The loaded Pd(II) ions can be easily stripped from 1 in the organic phase.
(6) 1 has a high solubility in various diluents that possess high boiling point and flash points (aliphatic and aromatic diluents).
(7) In liquid–liquid extraction, the phase separation is clearly observed after extraction and/or back extraction.
(8) Extraction with 1 follows a different mechanism from that of the commercial extractants.
Furthermore, with o-dichlorobenzene as a diluent, a high extraction percentage and clear phase separation are achieved in a short time (30 min) over the entire range of Cl− media (0.1–8.0 M HCl), which compares favorably with use of chloroform as a diluent. Thus, o-dichlorobenzene can be conveniently used as an industrial diluent for the purpose of extraction. We have utilized the pincer type structure of 1 for liquid–liquid extraction of Pd(II) from a leach liquor of an automotive catalyst for the first time, and comprehensively studied the Pd(II) complexation behavior and extraction mechanism through single-crystal X-ray diffraction analyses and other spectroscopic studies. The present study opens new applications of pincer ligands as extractants for metal ions in aqueous solutions.
Experimental
Materials and methods
Stock solutions of each metal ion were prepared using PdCl2 (Kanto Chemical Co., Inc.), PtCl4 (Acros Organics), RhCl3·3H2O, AlCl3, YCl3·6H2O, ZrCl4, LaCl3·7H2O, BaCl2·2H2O (Wako Pure Chemical Industries, Ltd.), and CeCl3·7H2O (Nacalai Tesque, Inc.) in HCl solution. Resorcinol, catechol, hydroquinone, 2,5-di-tert-butylhydroquinone, N,N-dimethylthiocarbamoyl chloride, K2CO3, acetone, o-dichlorobenzene, chloroform, Na2SO4, and all other chemicals were of reagent grade, were purchased from commercial sources, and were used without further purification. Metal solutions at various concentrations were prepared by dissolving the required quantities of the respective metal salts in doubly-distilled water.
Fourier transform infrared (FT-IR) spectra were measured at 4000–600 cm−1 using a Nicolet iS5 spectrophotometer (Thermo Fisher Scientific) by the attenuated total reflection (ATR) method.
Nuclear magnetic resonance (NMR) data were recorded on Bruker DPX 300 and JEOL 600SSS ECA-600 instruments. Chemical shifts are quoted as parts per million (ppm) relative to the peak of tetramethylsilane (TMS). Matrix-assisted laser desorption ionization time-of-flight mass (MALDI-TOF MS) spectra were taken on Bruker autoflexspeed-AK1. Electronic absorption spectra were recorded on a Shimadzu UV 3600 double beam spectrophotometer using 1 cm matched quartz cells. Elemental analysis was performed on a Systems Engineering CE-440M CHN/O/S elemental analyzer.
Synthesis of extractants
Structural formulas of the synthesized thioamide-based extractants, 1–4 are shown in Fig. 1. 1,3-Bis(dimethylthiocarbamoyloxy)benzene (1), 1,4-bis(dimethylthiocarbamoyloxy)benzene (2), 1,2-bis(dimethylthiocarbamoyloxy)benzene (4) were synthesized according to literature methods.19,20 Extractant 3 was synthesized as follows: 2,5-di-tert-butylhydroquinone (2.22 g, 10.0 mmol), N,N-dimethylthiocarbamoyl chloride (4.94 g, 40.0 mmol) and K2CO3 (5.53 g, 40.0 mmol) were stirred in acetone (200 mL) and were refluxed for 2 days under a nitrogen atmosphere. After the suspension had cooled, the solvent was removed in vacuo. The resulting solid material was dissolved in chloroform, and excess K2CO3 was quenched with 2 M HCl. The organic phase was then separated, washed with water, 5% KOH, and saturated NaCl, and dried over anhydrous Na2SO4. A brown solid was obtained by evaporation of the solvent. Recrystallization from CHCl3 yielded 3 as a colorless crystal (yield: 2.77 g, 70%). FT-IR (ATR) ν/cm−1: 2960 (C–H), 1527 (C
S) and 1120 (C–N). 1H NMR (300 MHz, CDCl3, TMS): δ 6.99 (2H, s, Ar-H), 3.10 and 3.41 (12H, s, N(CH3)2), 1.28 (18H, s, C(CH3)3).
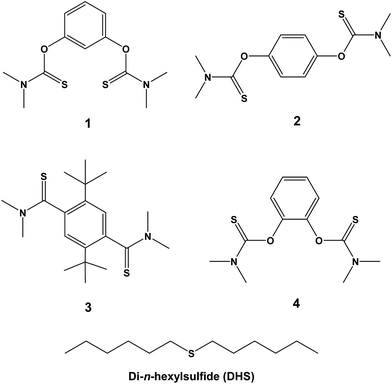 |
| Fig. 1 Structural formulas of 1,3-bis(dimethylthiocarbamoyloxy)benzene (1), 1,4-bis(dimethylthiocarbamoyloxy)benzene (2), 1,4-bis(dimethylthiocarbamoyloxy)2,5-di-tert-butylbenzene (3) 1,2-bis(dimethylthiocarbamoyloxy)benzene (4), and di-n-hexylsulfide (DHS). | |
Solvent extraction studies
Liquid–liquid extraction experiments were performed in duplicate at 20 ± 1 °C to ensure reproducibility. The values of extractability (E%) obtained were reproducible to within ±5%. The experiments were performed using diluent solutions of extractants (10 mL) with the desired concentration of metal ion solutions (10 mL) in glass bottles. The contents were shaken thoroughly using a mechanical shaker. The mixture was shaken at 300 strokes per min for the desired contact time. The aqueous and organic phases were separated using a separating funnel. The concentration of metal ions in the aqueous phase was measured by inductively coupled plasma atomic emission spectrometry (ICP-AES).
The Pd(II) concentration and E% were calculated using eqn (1) and (2):
|
E% = [M]org/[M]aq,init × 100
| (1) |
|
[M]org = ([M]aq,init − [M]aq)
| (2) |
where [M]
aq,init and [M]
aq are, respectively, the initial and final concentrations of the metal ions in aqueous solution. The volume ratio of the aqueous phase/organic phase (A/O) was 1
![[thin space (1/6-em)]](https://www.rsc.org/images/entities/char_2009.gif)
:
![[thin space (1/6-em)]](https://www.rsc.org/images/entities/char_2009.gif)
1 during the entire extraction experiments. All other specific conditions are explained in the discussion.
Liquid–liquid extraction of a simulated mixed solution containing nine metals (Pd, Pt, Rh, Y, Zr, Ba, Al, La, and Ce)
Liquid–liquid extraction experiments were carried out in which Pd was extracted from mixed solutions containing nine metals (Pd, Pt, Rh, Y, Zr, Ba, Al, La, and Ce) that simulate the leach liquor of automotive catalysts. A mixed solution containing 0.1 g L−1 each of Pd, Pt, Rh, Y, Zr, Ba, Al, La, and Ce ions in acidic media was prepared from the chloride salts of each metal. The diluted leach liquor of automotive catalyst contained 0.093 g L−1 Pd(II) ions and hence we have fixed 0.1 g L−1 of metal ion in the mixed metal ion solution in order to determine the Pd(II) selectivity of extractant 1 at very high concentrations of other metals. A 0.1 mM solution of extractant 1 in o-dichlorobenzene was prepared. Aliquots of 10 mL of the mixed solutions containing nine metals (1.0 M HCl media) were mixed with 10 mL of extractant 1 solution. Each mixture was shaken at 300 strokes per min for 30 min. After extraction, the concentrations of the nine metal ions in the aqueous phase were measured by ICP-AES.
Pd(II) ion loading capacity of extractant 1
A 0.1 mM extractant solution in o-dichlorobenzene was prepared. Pd(II) ion solutions with an initial concentration of 0.01–0.220 g L−1 were prepared in 1.0 M HCl media. Aliquots of 10 mL of different concentrations of Pd(II) ions were mixed with 10 mL of extractant 1. The mixture was shaken at 300 strokes per min for 30 min. After extraction, the concentrations of Pd(II) ions in aqueous phases were measured by ICP-AES.
Durability test of extractant 1 in hydrochloric acid media
o-Dichlorobenzene solutions of 0.1 M extractant 1 (40 mL) were stirred with 12.0 M HCl (40 mL) for 5 days. After extraction, the organic phase was evaporated and the acid treated extractant 1 was obtained. FT-IR spectra and 1H NMR of the acid treated extractant 1 were measured.
Liquid–liquid extraction of Pd(II) ions from leach liquors of automotive catalysts
Metal ions were leached from an automotive catalyst by treating the catalyst with a mixture of HCl (11.6 M) and H2O2 (1 vol%) at 65 °C for 3 h.21 The ratio of solid to liquid (pulp density) during the leaching was 500 g L−1. The resultant leached solution, which contained nine metal ions (Rh, Pd, Pt, Zr, Ce, Ba, Al, La, and Y), was diluted with distilled water to 5 times its original volume. The concentrations of metal ions in the diluted solution were determined by ICP-AES. A 1.0 mM solution of extractant 1 in o-dichlorobenzene (10 mL) was mixed with the diluted PGM solution (10 mL) in a 50 mL tube, and shaken at 300 strokes per min for 30 min. The concentrations of the metal ions remaining in the aqueous phase were determined by ICP-AES.
Stripping of Pd(II) ions from the organic phase
Extraction from the leach liquor of the automotive catalyst (10 mL) was carried out with a 10.0 mM solution of extractant 1 in o-dichlorobenzene (10 mL). Stripping of Pd(II) ions from the PGM-containing organic phase was then performed. After the extraction of the acidic leach liquors, the organic phase (10 mL) was mixed with 0.1 M thiourea in 1.0 M HCl (10 mL). The mixture was shaken at 300 strokes per min for 30 min (20 ± 1 °C), and the concentration of Pd(II) ions in the aqueous phase after stripping, [M]aq, was determined by ICP-AES. The stripping ability, S (%), was calculated using the following equation: |
S (%) = [Pd(II)]aq/[Pd(II)]org × 100
| (3) |
where [Pd(II)]aq is the concentration of Pd(II) ions in the aqueous solution after back extraction, and [Pd(II)]org is the concentration of metal ions in the organic phase before back extraction.
Recycling of extractant 1
The Pd(II) ion-stripped organic phase (10 mL) was shaken with water (30 mL) for 30 min at 300 strokes per min. The phases were then separated using a separating funnel. The water-washed extractant 1 was used for extraction–stripping cycles of the liquid–liquid extraction process carried out under the same conditions as those used for the acid leached automotive catalyst solution, above. Finally, the concentration of each metal ion in the aqueous phase, [M]aq, was determined by ICP-AES.
Synthesis of 1–Pd(Cl)·CHCl3 single crystal
The organic phase was evaporated to dryness, and a pale yellow solid was obtained with good yield (95%). This solid was dissolved in CHCl3 and allowed to stand at room temperature. After 5 days, single crystals of 1–Pd(Cl)·CHCl3 were formed. These crystals were analyzed by X-ray diffraction.
Structural determination for 1–Pd complex
Elemental analysis for 1 (C12H16O2N2S2), anal. calcd C, 50.68; H, 5.67; N, 9.85; found C, 50.75; H, 5.46; N, 9.91. For the 1–Pd complex (C12H15O2N2S2PdCl·CHCl3): anal. calcd C, 28.67; H, 2.96; N, 5.14, found C, 25.58; H, 2.78; N, 5.25.
MALDI-TOF MS spectra of 1 and 1–Pd are shown in Fig. S1 (in the ESI†). The MALDI-TOF MS analysis (m/z) for 1: calcd 307.37; found 307.37 [M − Na]+; for 1–Pd: calcd 389.78; found 389.30 [M − Pd]+.
X-ray crystallography measurement
The crystals in mother liquid were picked up with a pipette, and dropped in Paratone oil. The single crystals coated with oil were isolated on MicroMounts™, and the crystals were placed in a cold nitrogen steam at 100 K. X-ray diffraction data were collected on Rigaku R-AXIS RAPID imaging plate diffractometer equipped with graphite-monochromated Mo Kα radiation. The structure was solved by direct methods using SHELXS-97 program.
Crystal data. C12H15ClN2O2PdS2·CHCl3, M = 544.61, yellow, crystal dimensions 0.30 × 0.20 × 0.15 mm3, monoclinic, space group P21/c, crystal description prism, a = 10.1528(6), b = 27.9401(16), c = 7.5008(6) Å, α = 90.0000°, β = 115.004(15)°, γ = 90.0000°, V = 1928.3(3) Å3, Z = 4, Mo Kα radiation (λ = 0.71075 Å), ρcalcd = 1.876 g cm−3, T = 100 K, μ(Mo Kα) = 1.742 mm−1, 17
464 measured reflections, 4419 unique reflections (Rint = 0.1275), 3675 observed reflections (I > 2σ(I)), 217 parameters, R = 0.0592, wR = 0.1032, refined against |F|, GOF = 1.079. ESI.†
Results and discussion
Extraction of Pd(II) ions as a function of HCl concentration
The efficiency of Pd(II) ion extraction by extractants 1–4 depends on the acid concentration (Fig. 2). Extraction experiments were carried out for 1 mM Pd(II) in 0.1–8.0 M HCl solutions with 10 mM and 1 mM solutions of DHS and extractants 1–4, respectively, in CHCl3, for 12 h. Extractants 1, 2, and 3 showed an extractability (E%) of greater than 99% in 0.1 M HCl. However, DHS, a well-known Pd extractant, showed an E% of only 68% only. The Pd(II) extraction of 1 was 99.5–88.0% in 1–8 M HCl; i.e., it is not much affected by the HCl concentration. The E% of extractant 2 remained close to 100% up to an HCl concentration of 1.0 M, then fell gradually to 35% as the HCl concentration was further increased to 8.0 M. In contrast, the E% of extractant 3 showed a significant reduction to around 20% for an HCl concentration of 1.0 M, but remained steady at around this level at higher HCl concentrations. The E% of DHS showed a steady decrease with HCl concentration over its whole range. Extractant 4 showed negligible extraction over the entire range of HCl concentration studied. Since the E% for DHS and 3 is below 50–20% in 1–8 M HCl, these results indicate that DHS and extractants 3 and 4 and are not suitable for the extraction of Pd(II) at HCl concentrations of higher than 1.0 M. For almost all sulfur-based extractants, the E% of Pd(II) ions decreases with increasing Cl− concentration in the medium.6,13 However, in the case of extractant 1, the extractability is almost unaffected by HCl concentration. The E% of extractants 1–3 and DHS is high in comparison to that of 4. Hence, extractant 4 was not included in further studies.
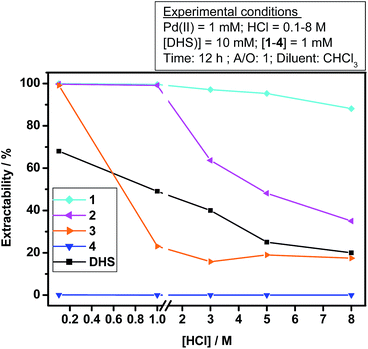 |
| Fig. 2 Extractability of Pd(II) ions by 1–4 and DHS, as function of HCl concentration. | |
Effect of contact time
The effect on the E% values of 1–3 and DHS of contact time in the 5–120 min range were determined (Fig. 3). CHCl3 solutions (10 mL) of 1–3 (1 mM) and DHS (2 mM) were mixed with 1 mM Pd(II) solutions in 0.1 M HCl (10 mL). After extraction, the concentration of Pd(II) ions in the aqueous phase was measured. The E% values of 1 and 2 reached saturation at 30 min and E% = 99.9% and 99.5%, respectively, whereas 3 showed an E% value of only 28% after 120 min. Extractants 1 and 2 showed more rapid Pd(II) extraction and higher E% than DHS and 3. Hence, further studies were limited to 1 and 2 with a contact time of 30 min.
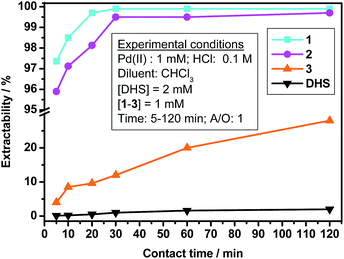 |
| Fig. 3 Effect of contact time on Pd(II) extraction by 1–3 and DHS. | |
Effect of diluents on the extraction of Pd(II) ions
We performed Pd(II) extractions from 1 mM Pd(II) solutions (10 mL) and 1 mM solutions of 1 and 2 (10 mL) in 11 different diluents. Extractants 1 and 2 are not soluble in kerosene, n-decane, ShellSol D70®, and cyclohexane. Furthermore, extractant 2 is not soluble in xylene. However, both are soluble in the other diluents studied. The Pd(II) extraction of 2 showed third phase (solid) formation with the use of o-dichlorobenzene, toluene, and chlorobenzene as diluents. Hence, 2 is not suitable for the extraction of Pd(II) in PGM refineries. Extractant 1 showed excellent Pd(II) extraction in the diluents studied, and good phase separation was obtained (Table 1). Among the studied diluents, CHCl3 and o-dichlorobenzene showed very high Pd(II) extractabilities with 1, and hence further studies were performed in CHCl3 and o-dichlorobenzene only.
Table 1 Effect of diluents on the extraction of Pd(II) ions by 1 and 2a
Solvents |
Boiling point (°C) |
Density (g cm−3) |
Flash point (°C) |
Dipole moment (D) |
Extractability% |
1 |
Remarks |
2 |
Remarks |
Conditions: Pd(II) = 1 mM in 0.1 M HCl; [E] = 1 mM; time = 30 min; A/O: 1; shaking speed = 300 strokes per min. |
o-Dichlorobenzene |
180.3 |
1.30 |
66 |
2.27 |
99.5 |
Clear separation |
97.5 |
3rd phase formation |
Chlorobenzene |
131.0 |
1.11 |
29 |
1.54 |
94.2 |
Clear separation |
95.3 |
3rd phase formation |
Toluene |
110.0 |
0.86 |
4.4 |
0.31 |
95.0 |
Clear separation |
97.3 |
3rd phase formation |
1,2-Dichloroethane |
83.5 |
1.25 |
13 |
1.80 |
97.0 |
Clear separation |
90.4 |
Clear separation |
Chloroform |
61.0 |
1.49 |
— |
1.04 |
99.8 |
Clear separation |
99.5 |
Clear separation |
Dichloromethane |
40.0 |
1.32 |
— |
1.60 |
98.2 |
Clear separation |
91.5 |
Clear separation |
Xylene (mixture) |
140.0 |
0.86 |
22 |
0.35 |
92.0 |
Clear separation |
— |
2 not soluble |
Cyclohexane |
81.0 |
0.77 |
−18 |
0 |
— |
1 not soluble |
— |
2 not soluble |
n-Decane |
214.0 |
0.74 |
71 |
0.07 |
— |
1 not soluble |
— |
2 not soluble |
Kerosene |
150–300 |
0.81 |
38–66 |
— |
— |
1 not soluble |
— |
2 not soluble |
ShellSol D70® |
193–245 |
0.79 |
74 |
— |
— |
1 not soluble |
— |
2 not soluble |
Extractability (E%) of extractant 1 in o-dichlorobenzene and CHCl3 with HCl concentrations of 0.1–0.8 M and a short contact time
In general, PGM ions have been extracted from very high Cl− content media in industrial processes. The efficiency of Pd(II) extraction dramatically decreases even for very small increases of acidity, and Pd(II) extraction is primarily dependent on the HCl concentration of the solution. For industrial extraction applications, the developed extractant must bind metal ions with a short equilibrium time, in a wide range of acid media. We performed Pd(II) extractions with 0.1 mM Pd(II) ion solutions (10 mL) and 1 mM solutions of 1 (10 mL) in o-dichlorobenzene and CHCl3 with 0.1–8.0 M HCl with a short contact time (30 min). As is evident from Fig. 4, with o-dichlorobenzene as the diluent, 1 displayed E% values of 99.9–97.0% in 0.1–8.0 M Cl− media. It is clear that 1 dissolved in o-dichlorobenzene, and that the E% of rapid Pd(II) extraction is not strongly dependent on the HCl concentration. When dissolved in CHCl3, 1 showed an E% of 99.9% at 0.1 M HCl, but with increasing HCl concentration, the E% gradually deceased to 48%. In CHCl3, a long contact time is required to reach extraction equilibrium; we found that equilibrium is reached after 12 h, where E% is 88.0% in 8 M HCl (cf. Fig. 2). In contrast, using o-dichlorobenzene as a diluent, a high E% is observed over the entire range of Cl− media (0.1–8.0 M HCl) within short time (30 min). o-Dichlorobenzene is suitable for refineries because its high boiling point (180.5 °C) and density (1.3 g cm−3) enable rapid separation from the aqueous phase.
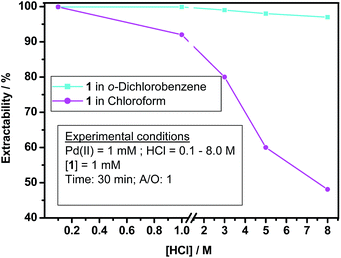 |
| Fig. 4 E% of 1 in o-dichlorobenzene and chloroform with a wide range of Cl− media. | |
Loading capacity of Pd(II) ions and acid durability of extractant 1
The Pd(II)-loading capacity of extractant 1 (1.0 mM) in the o-dichlorobenzene phase was investigated from an initial Pd(II) concentration ranging from 10 to 220 mg L−1 over a fixed contact time of 30 min in 1.0 M HCl. The Pd(II)-loading capacity of 1 is displayed in Fig. S2 in the ESI.† A Pd/extractant ratio of 1 for extractant 1 is evident when the 1.0 mM organic phase was saturated with 220 mg L−1 of Pd(II) at 1.0 M HCl. Extractant 1 has very high loading capacity even in 1.0 M HCl media. These results suggest that Pd(II) is extracted by the pincer ligand to form a 1
:
1 Pd–extractant complex. In contrast, DHS and many other reported Pd ion extractants form a 1
:
2 Pd–extractant complex.12a,13,22 Thus, extractant 1 is an economical extractant, which requires a concentration half that of DHS for Pd(II) ion extraction.
For PGM recovery from acid leach liquors of automotive catalysts, extractants should be stable in acid media and not deteriorate with continuous usage.23 In order to check the acid stability of extractant 1, we have carried out a durability test. A 0.1 M solution of extractant 1 (10 mL) in chloroform was stirred with of 12.0 M HCl (10 mL) for 5 days. The chloroform phase was then separated and washed with water, and the chloroform was evaporated. The FT-IR spectra and 1H NMR of the acid-treated compound were recorded in order to investigate possible deterioration or oxidation of the extractant. As shown in Fig. S3 in the ESI,† a good correspondence between the FT-IR spectra of the acid-treated 1 and native 1 is evident, with no new peaks apparent after the acid treatment. This indicates that 1 is highly stable and suitable for metal extraction in acidic media. Similarly, the 1H NMR spectra of the acid-treated extractant 1 showed only peaks corresponding to native 1 (figure not shown). Hence, extractant 1 is durable in HCl media.
Extraction of Pd(II), Pt(IV), Rh(III), and other metals from simulated mixed-metal solutions
In order to study the selectivity for Pd(II) extraction by 1, we conducted extractions from the simulated mixed-metal solution. Very high amounts of Pd(II) ions were extracted from the mixed-metal ion solutions by 1, as shown in Fig. 5. In this study, the Pd(II) extraction ability of 1 was found to be 99.9%. Extractant 1 showed a clear selectivity toward Pd(II) ions, with over extraction of Pt, Rh, Y, Zr, Ba, Al, La, and Ce ions found to be negligible.
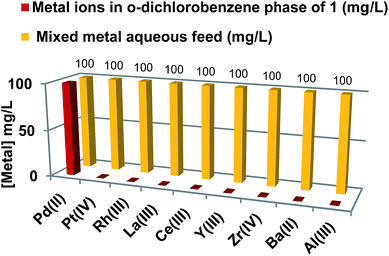 |
| Fig. 5 Extraction of Pd(II), Pt(IV), Rh(III) and other metals ions by 1 from mixed-metal solutions. | |
Recovery of Pd(II) from leached automotive catalyst solution
With the results in hand from our optimization experiments with standard solutions, we then moved on to study the liquid–liquid extraction of PGMs from leach liquors of automotive catalysts using extractant 1. The solutions were prepared by treating the automotive catalyst residue with HCl (11.6 M) and H2O2 (1.0 vol%).21 The resultant PGM solution contained nine metal ions, namely, Rh, Pd, Pt, Zr, Ce, Ba, Al, La, and Y, and was diluted with water to 5 times its original volume for the liquid–liquid extraction study. The metal concentrations of the leached solution (1.0 M HCl) before extraction are shown in Fig. 6a. We performed extractions with leached automotive catalyst solutions (10 mL) and 1 mM solutions of 1 in o-dichlorobenzene (10 mL) for 30 min. Selective extraction of 99.9% of Pd(II) ions by 1 from an automotive catalyst residue leached solution composed of Rh(III), Pd(II), Pt(IV), Zr(IV), Ce(III), Ba(II), Al(III), La(III), and Y(III) in 1.0 M Cl− media was achieved. None of the other metal ions in the PGM solutions were extracted. A schematic diagram of selective extraction of Pd(II) by 1 from automotive catalyst leach liquor is shown in Fig. 6b.
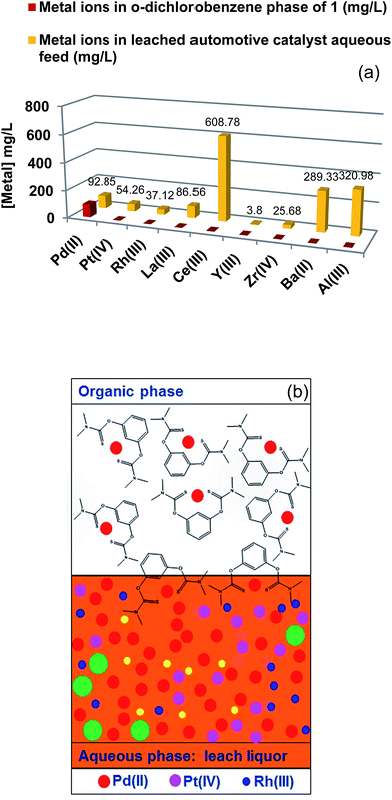 |
| Fig. 6 (a) Extraction of Pd(II) from a PGM solution containing nine metal ion (i.e., Al, Ba, Ce, La, Pd, Pt, Rh, Y, and Zr) by 1. (b) Schematic diagram of selective extraction of Pd(II) by 1 from automotive catalyst leach liquors. | |
Stripping of Pd(II) and reusability of 1
Our previous stripping studies showed that aqueous solutions 0.1 M thiourea and 1 M HCl stripped ca. 100% of the Pd(II) ions from an organic phase.15 Therefore back-extraction was attempted in the present study using a mixture of 0.1 M thiourea with 1 M HCl. The Pd(II) stripping experiment was conducted with the organic phase (10 mL) and 0.1 M thiourea with 1 M HCl solution (10 mL) for 30 min. The results of the Pd(II) ion stripping are shown in Fig. S4 in the ESI.† 1–Pd–Cl(org) complex was reacted with high concentration of protonated thiourea (0.1 M thiourea/1.0 M HCl) and forming the coordination bonds with Pd ions through sulfur atoms and releases the Pd ions to the stripping phase.14a,b,15
The stripping mechanism can be represented by following reaction.
|
1–Pd–Cl(org) + 2[CS(NH2)2H+](aq) → 1–H(org) + Pd[(CS(NH2)2)2]2+(aq)
| (4) |
A Pd(II) stripping ability of greater than 99% was achieved with the thiourea/HCl mixture as a stripping agent. After back-extraction, metal-free solutions of the organic phase containing extractant 1 were reused for the extraction of PGMs from the leached solution, under the same conditions as discussed earlier. Surprisingly, it was found that the organic phase was not capable of extracting Pd(II) ions from the solution. We hypothesized that this may be due to the presence of thiourea, and hence, the metal-free organic phase was subsequently washed with water in order to remove any thiourea present. As a result of the water washings, the reusability of the extractants/organic phase was confirmed over five cycles of extraction and stripping. The Pd(II) extraction ability of the recycled extractants 1 was found to be >99% after five extraction/stripping cycles. The high E%Pd (>99%) and S%Pd (>99%) for Pd(II) ions exhibited by 1 allows facile reuse of the extractant for >5 cycles.
Pd(II) extraction mechanism of extractants
The negligible extractability for Pd(II) ions shown by extractant 4 is due to presence of steric hindrance in the 1,2-positions of benzene substituted thiocarbamoyl groups, such that there is insufficient space to form a square planner Pd complex with the two thiocarbamoyl groups of 4.18a Extractant 3 displays very low Pd(II) ion extractability due to the steric hindrance in the 2,5-positions of the benzene substituted tertiary butyl groups, which inhibit the Pd(II) extraction capacity. In contrast, extractant 2 binds Pd(II) ions through complex formation between intermolecular thiocarbamoyl groups in a similar manner to DHS.
The solid sample used for the characterization of the extractant 1–Pd complex was isolated after Pd(II) extraction in CHCl3. Following the extraction, the aqueous and organic phases were separated. The organic phase was evaporated to dryness and a pale yellow solid was obtained. The FT-IR spectra of 1 and the 1–Pd solid complex were recorded, and are displayed in Fig. S5 in the ESI.† The FT-IR spectra of extractant 1 significantly changed after coordination with Pd(II) ions; the peak corresponding to N–C
S in 1 shifted from 1529 to 1556 cm−1 and the peak corresponding to C–H in 1 shifted from 2935 to 2961 cm−1. The shifts of these bands to higher frequency suggest that Pd(II) complexation occurs through the thiocarbamoyl group.18,23 The UV-visible spectra of 1 and 1–Pd are shown in Fig. S6 in the ESI.† The UV-visible spectrum of 1 in CHCl3 showed a single peak in the UV region at 253 nm, corresponding to the π–π* transition, whereas the 1–Pd complex showed a π–π* peak at 266, and another broad peak at 315–340 nm corresponding to metal-to-ligand charge-transfer (MLCT) and ligand-to-metal charge-transfer (LMCT) transitions. The complex is not light-emissive in solutions at room temperature, and hence no peak was obtained in the visible region. Thus, the UV-visible studies provide confirmation of the coordination of Pd(II) to 1.18b
Color changes before and after Pd(II) extraction and stripping were observed for extractants 1 and 2, as shown in Fig. S7 in the ESI.† Before the extraction process, the aqueous phase of 0.1 mM PdCl42− in 1.0 M HCl is yellow in color, and the 0.1 mM solutions of 1 and 2 in o-dichlorobenzene (organic phase) are colorless. After 30 min extraction, the yellow color completely disappeared in the aqueous phase due to extraction of Pd(II) to the organic phase. The same phenomenon was observed in all diluents used in the study. Generally, an organic phase becomes yellow or orange in color after Pd(II) extraction using a sulfur-based extractant, although no color change is observed for Pd extraction using the pincer ligand 1.18b However, the organic phase after extraction with 2 behaved differently to that of 1, i.e., the organic phase became orange in color, which confirms the typical palladium extraction mechanism of sulfur-based extractants. Extractant 1 clearly follows a very different Pd complexation mechanism from the typical process. After stripping of Pd(II) from organic phase of 1 with the 0.1 M thiourea/1 M HCl solution, the typical yellow color corresponding to PdCl42− is observed in the aqueous phase, which confirms the stripping of Pd(II). Similarly, after stripping of Pd(II) from the organic (orange) phase of 2 with 0.1 M thiourea/1 M HCl, the typical yellow color of PdCl42− is observed in the aqueous phase and the organic later becomes colorless. The color changes during stripping demonstrate the stripping mechanism and the facile reusability of the extractants. The clear mechanism of Pd extraction of 1 was further studied using NMR, MALDI-TOF MS, and single crystal XRD.
The 1H NMR spectra of 1 and 1–Pd are shown in Fig. 7. The spectrum of 1 shows δ (ppm) = 3.33 and 3.45 (2 × s, 6H), 6.84 (t, 1H, J = 2.4 Hz), 6.98 (dd, 2H, J = 2.4 and 8.4 Hz), 7.39 (t, 1H, J = 8.4 Hz). The spectrum of 1–Pd shows δ (ppm) = 3.45 and 3.55 (2 × s, 6H), 6.92 (d, 2H), 7.10 (t, 1H). In the 1H NMR spectrum of 1–Pd, the aromatic proton intensity is reduced by 1H. One of the N–CH3 signals of free 1 (3.33 and 3.45) is significantly de-shielded on Pd complexation (3.45 and 3.55). The plane of the (CH3)2NCS groups of free 1 is expected to be nearly perpendicular to the benzene ring, and hence one CH3 group is situated in the shielding region of the ring current.18a The 1H NMR clearly shows that Pd is directly bonded to C(5) during complexation and that the H attached to C(5) disappeared on Pd extraction. The results of 13C NMR analysis of 1 and 1–Pd are shown in Table S1 in the ESI.† The 13C NMR spectrum of 1–Pd showed that the shift in the peak assigned to the carbon (C(6)) atoms attached to the sulfur atoms, from 180 ppm to 187.0 ppm, and C(5) peak from 129.0 pm to 125.7 ppm after Pd(II) complexation, as compared with free 1.18a Thus, the 13C NMR spectrum also suggests that 1 extracts Pd ions through thiocarbamoyl groups. The elemental analysis and MS spectra (Fig. S1 in the ESI†) of 1–Pd show that one Pd atom is included in the complex. Based on these spectroscopic studies, it is confirmed that two thiocarbamoyl groups in the 1,3-positions of the benzene ring of 1 promote Pd complexation at the C(5) position of the S–C–S tridentate ligand, with formation of a stable fused six-membered chelate rings.
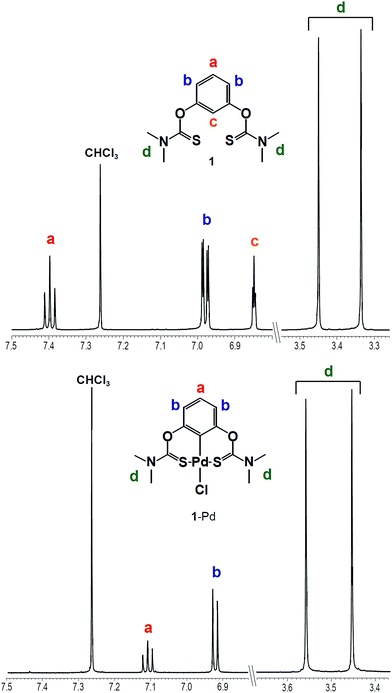 |
| Fig. 7 1H NMR spectra of 1 and 1–Pd. | |
Crystal structure of pincer ligand with Pd
The mechanism of Pd(II) extraction by 1 was further confirmed through a single-crystal X-ray diffraction study. The crystals of 1 were obtained as yellow block crystals in the monoclinic space group P21/c, while the asymmetrical unit comprised one pincer ligand 1 with a palladium(I) chloride (PdCl form) and one CHCl3 molecule (Fig. S8 in the ESI†). The overall composition is [{1}{Pd(I)+}{Cl−}(CHCl3)].
The crystal structure of 1 exhibits a distorted square planar structure, because Pd(I) acts as a stopper to inhibit the mobility of the two thiocarbamoyl moieties in the ligand. In fact, it is clear that the distorted structure of 1 is observed by two torsion angles of the thiocarbamoyl moieties as comparison with the aromatic ring of 1 (O1–O2–S2–Pd1 = 28.49(4)° and O2–O1–S1–Pd = 28.96(4)°, respectively). The environment of Pd+ is square planar coordination.
The Pd+ interacts with S1, S2, Cl1 and C5 belonging to the pincer ligand. The crystal structure of 1–Pd(Cl) is shown in Fig. 8. The bond distances and angles of the coordination environment are given Tables S2 and S3 of the ESI.† The crystal structure shows a distorted square plane with the formation of fused six-membered chelate rings. The geometry around the Pd center is a distorted square plane similar to that previously reported.18a The Pd–C and Pd–Cl bond lengths lie in the range of lengths found in related palladium complexes.24 The two thioamide groups in the 1,3-positions of the benzene ring promote palladation at the benzene ring C(5) position, since the reaction will result in production of an S–C–S tridentate ligand and the formation of stable fused six-membered chelate rings.
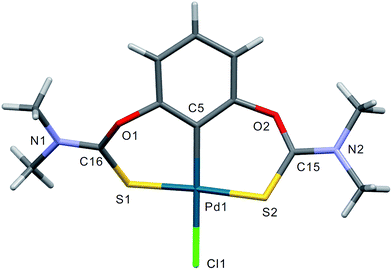 |
| Fig. 8 Crystal structure of 1–Pd(Cl). | |
Previously, Nonoyama and co-workers have reported18a a similar single crystal XRD structure of the palladium complex. They prepared palladium complexes with 1,3-bis(dimethylthiocarbamoyl)benzene in methanol/dichloromethane media, and reported formation of fused five-membered chelate rings. A description of halogen–halogen interaction and halogen–H interactions of the crystal is given in Fig. S9 of the ESI.† No examples of 1–PdCl complex having reported structure are found in the Cambridge Crystallographic Database (Sep 2015). The mechanism of Pd(II) extraction of SCS type pincer is given in Fig. 9.
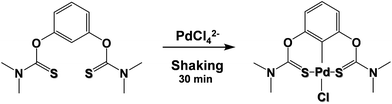 |
| Fig. 9 The mechanism of Pd(II) extraction by 1. | |
Conclusions
The extraction behavior using extractants 1–4 for Pd(II), Pt(IV), Rh(III), and other metal ions from HCl solutions was studied. Extractant 1 exhibited a good extraction performance as compared with extractants 2–4 and DHS. We have demonstrated that extractant 1 possesses a remarkably high efficiency and selectively for extraction of Pd(II) ions from solutions containing individual metal ions and from both a standard solution containing a mixture of metal ions and from leach liquors of automotive catalysts. The selective extraction of 99% of Pd(II) ions from the leach liquor of automotive catalysts, composed of Rh, Pd, Pt, Zr, Ce, Ba, Al, La, and Y, by extractant 1 in Cl− media was observed. In o-dichlorobenzene (1.0 mM), it selectively extracted 99.9–88% of Pd(II) (0.1 mM) in Cl− media (0.1–8.0 M), and additionally extracted Pd(II) ions in various diluents with clear phase separation. In contrast, extractant 2 showed third phase (solid) formation when o-dichlorobenzene, toluene, and chlorobenzene were used as diluents. The extractant 1–Pd complex was characterized, and its extraction mechanism was established. Extractant 1 coordinated Pd(II) ions via pincer type S–C–S tridentate ligands, with the formation of fused six-membered chelate rings. The S% towards Pd(II) ions from the organic phase was found to be 99% for extractant 1 when a mixture of 0.1 M thiourea solution with 1 M HCl solution was used as stripping agent. We also observed that the extractability of extractant 1 was high even after five extraction cycles (E%Pd ≥ 99%). The S%Pd also remained very high, and extractant 1 could be easily regenerated and reused. Extractant 1 is very stable in acid media can be used even in very highly acidic media for rapid and selective extraction of Pd(II) from acidic solutions of automotive catalysts and other secondary resources in PGM refineries. From these promising results, we can conclude that extractant 1 has great potential for applications in the extraction and separation of Pd(II) ions from leach liquors of automotive catalysts and other secondary resources in PGM refineries.
Acknowledgements
This work was supported by the “Program to Disseminate Tenure Tracking System,” MEXT, Japan.
References
- P. J. Loferski, Platinum-group metals, 2010 mineral year book, US geological survey, 2011 Search PubMed.
- A. Fornalczyk, J. Achiev. Mater. Manuf. Eng., 2012, 55, 864–869 Search PubMed.
- A. Fornalczyk and M. Saternus, Metalurgija, 2009, 48, 133–136 CAS.
- J. Tollefson, Nature, 2007, 450, 334–335 CrossRef CAS PubMed.
- C. Hagelüken, Platinum Met. Rev., 2012, 56, 29–35 CrossRef.
-
(a) P. Charlesworth, Platinum Met. Rev., 1981, 25, 106–112 CAS;
(b) F. L. Bernardis, R. A. Grant and D. C. Sherrington, React. Funct. Polym., 2005, 65, 205–217 CrossRef CAS.
-
(a) M. Aguilar and J. L. Cortina, Solvent extraction and liquid membranes: Fundamentals and applications in new materials, CRC Press, Florida, USA, 2008 Search PubMed;
(b) H. Narita and M. Tanaka, J. MMIJ, 2011, 127, 175–181 CrossRef CAS;
(c) C. Nowottny, W. Halwachs and K. Schiigerl, Sep. Purif. Technol., 1997, 12, 135–144 CrossRef CAS;
(d) M. Rovira, J. L. Cortina and A. M. Sastre, Solvent Extr. Ion Exch., 1999, 17, 333–349 CrossRef CAS;
(e) M. Regel-Rosocka, M. Wisniewski, A. Borowiak-Resterna, A. Cieszynska and A. M. Sastre, Sep. Purif. Technol., 2007, 53, 337–341 CrossRef CAS.
-
(a) K. Lillkung and J. Aromaa, Hydrometallurgical recovery of platinum group metals, School of Chemical Technology, Aalto University publication series Science + Technology, 2012 Search PubMed;
(b) G. Levitin and G. Schmuckler, React. Funct. Polym., 2003, 54, 149–154 CrossRef CAS;
(c) K. Ueda, S. Morisada, H. Kawakita and K. Ohto, Solvent Extr. Res. Dev., Jpn., 2014, 21, 9–19 CrossRef.
- H. Narita, M. Tanaka and S. Uneo, Effect of thiodiglycolamide addition to di-n-hexyl sulfide on the Pd(II) extraction rate. TMS 2012 141st annual meeting and exhibition, supplemental proceedings. Materials processing and interface, John Wiley & Sons, USA, 2012, vol. 1, pp. 173–177 Search PubMed.
-
(a) H. Narita, M. Tanaka, M. Morisaku and K. Tamura, Durability of two extractants for Pd(II) separation, thiodiglycolamide and di-n-hexyl sulfide: against a mixed solution of HNO3 and HCl, in Advanced processing of metals and materials (Sohn international symposium). New, improved and existing technologies: Non-ferrous materials extraction and processing, ed. F. Kongoli and R. G. Reddy, John Wiley & Sons, Inc., USA, 2006, vol. 4 Search PubMed;
(b) H. Renner, The selective extraction of palladium by the use of di-n-hexyl sulfide, Council for mineral technology, Report No. M217, 1985 Search PubMed.
-
(a) J. Traeger, J. König, A. Städtke and H.-J. Holdt, Hydrometallurgy, 2012, 127–128, 30–38 CrossRef CAS;
(b) J. Traeger, T. Klamroth, A. Kelling, S. Lubahn, E. Cleve, W. Mickler, M. Heydenreich, H. Müller and H.-J. Holdt, Eur. J. Inorg. Chem., 2012, 2341–2352 CrossRef CAS.
-
(a) N. E. El-Hefny and J. A. Daoud, J. Phys. Sci., 2013, 24, 35–47 CAS;
(b) F. Hamada, C. Li and Y. Kondo, US Pat. 8,557,020 B2, 2013;
(c) Y. Kondo, A. Shibayama, F. Hamada, M. Yamada, M. Akama and T. Imai, US Pat. 2010/0129277 A1, 2010;
(d) M. Yamada, A. Shibayama, Y. Kondo and F. Hamada, Int. J. Soc. Mater. Eng. Resour., 2007, 15, 13–15 CrossRef CAS.
-
(a) H. Narita, M. Tanaka, K. Morisaku and T. Abe, Chem. Lett., 2004, 33, 1144–1145 CrossRef CAS;
(b) H. Narita, M. Tanaka and K. Morisaku, Miner. Eng., 2008, 21, 483–488 CrossRef CAS;
(c) H. Narita, K. Morisaku, K. Tamura, M. Tanaka, H. Shiwaku, Y. Okamoto, S. Suzuki and T. Yaita, Ind. Eng. Chem. Res., 2014, 53, 3636–3640 CrossRef CAS.
-
(a) A. P. Paiva, G. I. Carvalho, M. I. Costa, A. M. Rosa da Costa and C. Nogueira, Solvent Extr. Ion Exch., 2014, 32, 78–94 CrossRef CAS;
(b) O. Ortet and A. P. Paiva, Hydrometallurgy, 2015, 151, 33–41 CrossRef CAS;
(c) S. Kagaya, E. Tanaka, N. Kawai, I. Masore, E. Sato, K. Hasegawa, M. Kishi and T. Kanbara, J. Inorg. Organomet. Polym. Mater., 2009, 19, 67–73 CrossRef CAS.
-
(a) M. Rajiv Gandhi, M. Yamada, Y. Kondo, R. Sato and F. Hamada, Ind. Eng. Chem. Res., 2014, 53, 2559–2565 CrossRef CAS;
(b) M. Yamada, M. Rajiv Gandhi, Y. Kondo, A. Shibayama and F. Hamada, Sep. Sci. Technol., 2015, 50, 1964–1973 CAS.
-
(a) D. Morales-Morales and C. Jensen, The chemistry of pincer compounds, Elsevier Science, Amsterdam, 2007 Search PubMed;
(b) K. Okamoto, J. Kuwabara and T. Kanbara, J. Organomet. Chem., 2011, 696, 1305–1309 CrossRef CAS;
(c) D. Morales-Morales, Rev. Soc. Quím. Méx., 2004, 48, 338–346 CAS.
-
(a) M. Albrecht and G. van Koten, Angew. Chem., Int. Ed., 2001, 40, 3750–3781 CrossRef CAS;
(b) J. T. Singleton, Tetrahedron, 2003, 59, 1837–1857 CrossRef CAS;
(c) J. Kuwabara and T. Kanbara, J. Photopolym. Sci. Technol., 2008, 21, 349–353 CrossRef CAS;
(d) K. Okamoto, T. Kanbara and T. Yamamoto, Chem. Lett., 2006, 35, 558–559 CrossRef CAS;
(e) J. Kuwabara, G. Munezawa, K. Okamoto and T. Kanbara, Dalton Trans., 2010, 39, 6255–6261 RSC;
(f) P. Steenwinkel, R. A. Gossage, T. Maunula, D. M. Grove and G. van Koten, Chem.–Eur. J., 1998, 4, 763–768 CrossRef CAS.
-
(a) S. Takahashi, M. Nonoyama and M. Kita, Transition Met. Chem., 1995, 20, 528–532 CrossRef CAS;
(b) M. Akaiwa, T. Kanbara, H. Fukumoto and T. Yamamoto, J. Organomet. Chem., 2005, 690, 4192–4196 CrossRef CAS;
(c) K. Okamoto, J. Kuwabara and T. Kanbara, Chem. Lett., 2015, 44, 102–110 CrossRef;
(d) T. Kanbara, K. Okada, T. Yamamoto, H. Ogawa and T. Inoue, J. Organomet. Chem., 2004, 689, 1860–1864 CrossRef CAS;
(e) Y. Ogawa, A. Taketoshi, J. Kuwabara, K. Okamoto, T. Fukuda and T. Kanbara, Chem. Lett., 2010, 39, 385–387 CrossRef CAS;
(f) J. Liu, Y. Deng, C. Lin and A. Lei, Chem. Sci., 2012, 3, 1211–1214 RSC;
(g) H. Wang, J. Liu, Y. Deng, T. Min, G. Yu, X. Wu, Z. Yang and A. Lei, Chem.–Eur. J., 2009, 15, 1499–1507 CrossRef CAS PubMed.
- H. J. Kurth, U. Kaatz and F. Korte, Chem. Ber., 1973, 106, 2419–2426 CrossRef CAS.
- M. S. Newman and H. A. Karnes, J. Org. Chem., 1966, 31, 3980–3984 CrossRef CAS.
- S. Harjanto, Y. Cao, A. Shibayama, I. Naitoh, T. Nanami, K. Kasahara, Y. Okumura, K. Liu and T. Fujita, Mater. Trans., 2006, 47, 129–135 CrossRef CAS.
- Y. Baba, T. Eguchi and K. Inoue, J. Chem. Eng. Jpn., 1986, 19, 361–366 CrossRef CAS.
- M. Rajiv Gandhi, M. Yamada, Y. Kondo, A. Shibayama and F. Hamada, Hydrometallurgy, 2015, 151, 133–140 CrossRef CAS.
-
(a) A. G. Orpen, L. Brammer, F. H. Allen, O. Kennard, D. G. Watson and R. Taylor, J. Chem. Soc., Dalton Trans., 1989, S1–S83 RSC;
(b) J. E. Kickham and S. J. Loeb, Inorg. Chem., 1994, 33, 4351–4359 CrossRef CAS.
Footnote |
† Electronic supplementary information (ESI) available: The FT-IR spectra of 1 and 1–Pd complex; UV-visible spectra of 1 and 1–Pd complex; XRD data; supplementary tables and figures are provided. CCDC 1422037. For ESI and crystallographic data in CIF or other electronic format see DOI: 10.1039/c5ra23146a |
|
This journal is © The Royal Society of Chemistry 2016 |
Click here to see how this site uses Cookies. View our privacy policy here.