DOI:
10.1039/C5RA23232E
(Paper)
RSC Adv., 2016,
6, 9066-9071
Modification of cellulose paper with polydopamine as a thin film microextraction phase for detection of nitrophenols in oil samples†
Received
4th November 2015
, Accepted 5th January 2016
First published on 8th January 2016
Abstract
Cellulose paper was modified with polydopamine by a simple, efficient, and environmentally friendly approach, and used as a novel extraction phase for thin film microextraction (TFME). The untreated and polydopamine-treated cellulose papers were characterized by Fourier transform infrared spectroscopy (FT-IR), energy dispersive X-ray spectroscopy (EDS) and scanning electron microscopy (SEM). In this work, the modified cellulose paper was evaluated as a sorbent for the extraction of phenolic compounds, in particular 4-nitrophenol and 2,4-dinitrophenol, from oil samples. And various extraction parameters were optimized by selecting the desorption condition, sodium hydroxide concentration and extraction time. High-performance liquid chromatography (HPLC) combined with ultraviolet (UV) and visible-light detection was used for the quantification of the extracted compounds. Under optimum conditions, calibration curves in the range of 5–1000 μg L−1 with a good linearity (r ≥ 0.9982) and low limits of detection (LODs) were achieved. The limits of detection were 1.54 μg L−1 for 4-nitrophenol and 2.16 μg L−1 for 2,4-dinitrophenol. Enrichment factors (EFs) were in the range of 146–179 and relative standard deviations (RSDs) were less than 4.31% for the target analytes. The method was successfully used to investigate cooking oils. Relative recoveries were found to range from 84.37% to 100.85%. The proposed method provides a simple, efficient and environmentally friendly approach for the rapid and convenient detection of nitrophenols in oil samples.
1. Introduction
Phenolic compounds can be released into the environment directly and indirectly. In recent years, phenols have been of wide concern.1,2 Phenolic compounds are almost ubiquitous contaminants and appear to be harmful to the environment, as they appear widely in discharged wastewater from oil refineries, drugs, and paint and pesticide industries, and as the intermediates of various processes.3 Moreover, these pollutants are harmful to organisms in general, even at low concentrations,4 and they have the potential to harm the health of humans in particular. For example, they may cause headaches, nausea, dizziness, difficulty in swallowing, diarrhea, vomiting, shock, convulsions, and even death. Even more worrisome is that phenols can affect the central nervous system, liver, and kidneys.5–7 Nitrophenols, which constitute a type of phenolic compound, have been identified by the US EPA as a hazardous pollutant because of their very poor biodegradability and their highly toxic properties.8 Because of the potential harm of phenolic compounds to human health and the environment, it is urgent to remove them from water, food and the environment.
To extract phenolic compounds from samples, many techniques have been developed such as liquid–liquid extraction (LLE),9 liquid-phase microextraction (LPME),10 solid-phase extraction (SPE),11,12 solid-phase microextraction (SPME),13 cloud point extraction (CPE),14 stir bar microextraction (SBME)15 and ionic liquids extraction.16 Among these techniques, microextraction techniques are simple, miniaturized and consume less organic solvent. SPME,17 single-drop microextraction (SDME),18,19 dispersive liquid–liquid microextraction (DLLME),20,21 hollow fiber liquid-phase microextraction (HF-LPME),22 supramolecular solvent-based microextraction (SM-SBME)23–27 and solidified floating organic drop microextraction (SFODME)28,29 are some examples of microextraction techniques. SPME has become one of the most effective microextraction techniques because it is simple, inexpensive, fast to carry out and involves a low consumption of organic solvents. The principle of SPME is based on the interactions of analytes with the sample matrix and the extraction phase (coating) via adsorption and desorption (depending on the nature of the coating). The extraction selectivity and efficiency of SPME mainly depend on the properties and size of the coating used as well as on its interactions with the analytes. In addition, the initial rate of SPME extraction has been recently shown to be proportional to the surface area of the extraction phase. Extraction efficiency has been shown to increase with an increase of the amount of coating. However, increasing the thickness of the coating has been reported to result in a long time to reach equilibrium.30 In order to overcome this shortcoming, thin film microextraction (TFME), which makes use of the high ratio between the surface area and volume of a thin film, was introduced as a mode of SPME by the Pawliszyn research group in 2003.31 Thus, chemical modification of a cellulose surface with suitable organic reagents makes cellulose appropriate for extraction purposes. Extraction phases are often appropriate for aqueous samples but rarely for oil samples. It is therefore necessary to find a chemical modification method to make them applicable to oil samples. Dopamine has been found to be up to this task. Dopamine is widely known as a catecholamine neurotransmitter, and has excellent biocompatibility32 and self-polymerizing abilities.33,34 Hong et al. reported the formation of stable polydopamine (PDA) by non-covalent self-assembly and covalent polymerization.35 Drawing inspiration from the adhesive proteins of mussels, investigators have polymerized dopamine in alkaline aqueous media to generate polymer films that adhere onto the surfaces of practically all materials including polymers, ceramics, metals and metal oxides.36 Oxidants have been reported to induce polymerization of dopamine in neutral or acidic pH.33 In this work, dopamine was made to polymerize in alkaline aqueous solutions and hence to bind the surface of cellulose paper, and this modified cellulose paper was applied for TFME. TFME parameters such as desorption condition, sodium hydroxide concentration and extraction time were studied and optimized. Under the optimized conditions, cooking oils were investigated.
2. Experimental
2.1. Chemicals and materials
Dopamine hydrochloride (98%) was obtained from Aladdin Chemistry Co., Ltd. Tris-(hydroxymethyl)aminomethane (99%) was purchased from Shanghai Xinhua Chemical Factory (Shanghai, China). Cellulose paper was purchased from Xinhua Paper Industry Co., Ltd (Hangzhou, China). Ethanol, dichloromethane, n-hexane, methanol (HPLC grade) and acetonitrile (HPLC grade) were obtained from Sinopharm Chemical Reagent Co., Ltd. Acetone and hydrochloric acid (36–38%) were purchased from Haohua Chemical Reagent Co., Ltd. (Luoyang, China). Sodium hydroxide was provided by Tianjin Kemiou Chemical Reagent Co., Ltd. (Tianjin, China). 4-Nitrophenol and 2,4-dinitrophenol were purchased from Sinopharm Chemical Reagent Co., Ltd. Each standard stock solution was prepared by dissolving the required amounts in HPLC grade methanol at a concentration of 1.00 mg mL−1 and stored at 4 °C in darkness. Working standard solutions of 4-nitrophenol and 2,4-dinitrophenol were prepared daily from appropriate dilutions with HPLC grade methanol before use. All chemicals used were of analytical reagent grade, unless otherwise stated.
2.2. Instrumentation
FT-IR spectra were obtained using an FT-IR spectrophotometer (FT-IR NEXUS, Nicolet) to identify the functional groups. Energy dispersive X-ray spectroscopy (EDS) was performed on a GENESIS system (EDAX Inc.) attached to a JEM-2100 microscope. Microstructures and morphologies were investigated using scanning electronic microscopy (SEM, JEOL JSM-6390LV). A THZ-82(A) thermostatic water-bath shaker (Jintan Scientific Analytical Instrument Co. Ltd., China) was used in the reaction and TFME process. The 1200 series high-performance liquid chromatography system (Agilent Technologies Inc., USA) equipped with a multi-wavelength detector was used for detecting the phenolic compounds (4-nitrophenol,2,4-dinitrophenol). An Agilent ChemStation program was used to control the instrument, data acquisition and analysis (Agilent, USA). The analytical column employed was a Zorbax C18 column (4.6 mm × 250 mm, 5 μm particle size) (Agilent, USA). The components of the mobile phase were high-purity water with 2% acetic acid and methanol in the ratio of 42/58 (v/v). The flow rate was set at a constant 1.0 mL min−1. The detection was performed at 230 nm. The injection volume was 20 μL and the separation temperature was 25 °C.
2.3. Preparation of PDA-modified cellulose paper
For synthesis of the PDA-modified cellulose papers, we cut the cellulose filter papers into rectangular strips (1 cm × 4 cm) with a pair of scissors. The tapes were washed with acetone and dried at room temperature. The dopamine solution was first made by dissolving dopamine hydrochloride (300 mg) in 100 mL Tris–HCl buffer (10 mM, pH 8.5). Then the tapes were immersed into the dopamine solution with shaking (120 rpm) at 30 °C under an aerobic atmosphere for 24 h. After the reaction, the PDA-treated cellulose paper was thoroughly washed with deionized water and ethanol several times to remove the non-adhered PDA, and then dried under vacuum at 60 °C for 10 h before use.
2.4. TFME procedure
First, 1.00 mL of vegetable oil, a 200 μL mixture of 4-nitrophenol and 2,4-dinitrophenol (10 μg mL−1), 9 mL n-hexane and 5 μL sodium hydroxide (2 mol L−1) were added in a 10 mL conical flask in sequence and shaken well to produce final nitrophenol concentrations equivalent to 200 μg L−1. Then the tapes obtained from the procedure described in section 2.3 were cut into two parts (1 cm × 2 cm) and one of the tapes was put into the above conical flask. This conical flask and its contents were shaken in a thermostatic water-bath shaker at 150 rpm at 30 °C for 120 min. After extraction, the tape was removed from the conical flask and washed with n-hexane, and then dried at room temperature. Then, the tape was rolled into a 1.5 mL centrifuge tube containing 1.25 mL desorption solvent. After 10 min, the tape was removed from the tube and the solvent was filtered by a PTFE filter. Then the solvent was evaporated under a stream of nitrogen and the final residue reconstituted in 50 μL of methanol. Finally, a 20 μL portion was injected into the HPLC system for chromatographic analysis.
2.5. Oil samples
Five kinds of vegetable oil were purchased from local supermarkets and investigated under optimum adsorption and desorption conditions. One kind of vegetable oil was used to optimize the adsorption and desorption conditions while other kinds of the vegetable oil were used for recovery.
3. Results and discussion
3.1. Use of PDA-modified cellulose paper as an extraction phase
Cellulose is a linear homopolymer of linked glucopyranose units that aggregate to form a highly ordered structure due to its chemical constitution and spatial conformation.37 The water solubility of cellulose is low, but the presence of OH functional groups in its chemical structure enhances its polarity. It is therefore difficult to use cellulose as a reversed-phase sorbent for the extraction of nonpolar and semipolar compounds. In addition, water can react with OH functional groups in cellulose fibers, and hence penetrate into and destroy the fiber network. Cellulose paper is mainly made up of cellulose. In order to reduce the hydrophilic character of cellulose fibers and improve their adhesion properties, it is necessary to chemically modify their surface.38 But most currently available forms of cellulose fiber are only suitable for aqueous samples, so it is necessary to find a chemical modification to also make forms of these fibers usable for oil samples. In this work, cellulose paper was modified with polydopamine, which is able to bind nitrophenols in oil samples. One of the most obvious characteristics observed when the paper was treated with polydopamine was that the colour of the paper changed from white to black. Energy dispersive X-ray spectra (EDS) of plain cellulose paper and PDA-treated cellulose paper are shown in Fig. 1. Comparing these spectra revealed the presence of the element N only in the PDA-treated cellulose paper, which confirmed that polydopamine was successfully bound to the cellulose paper surface. In order to further verify that the cellulose paper was indeed modified by PDA, SEM images of cellulose papers Fig. 2(a–c) and PDA-treated cellulose papers Fig. 2(d–f) at different magnifications were acquired (Fig. 2). From Fig. 2, an obvious difference between the plain and PDA-treated cellulose papers could be seen. The tubes of cellulose fibers in the untreated cellulose paper were observed to be smooth at all magnifications (Fig. 2a–c), while they were rough and appeared to have a compact coating with several clusters of small particles in the highest-magnification SEM image in the PDA-treated paper (Fig. 2f). The presence of these small particles is mainly attributable to aggregation of adjacent polydopamine. In addition, FT-IR analysis was carried out to check for the presence of polydopamine. The FT-IR analysis (Fig. S1†) showed several weak peaks at 1430–1650 cm−1 in the PDA-treated cellulose paper. The weak peaks at 1430–1650 cm−1 resulted from the benzyl functional group.
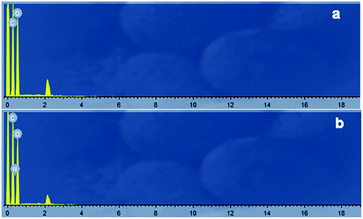 |
| Fig. 1 EDS of plain cellulose filter paper (a) and PDA-treated cellulose paper (b). | |
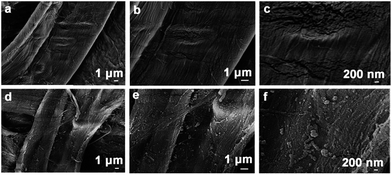 |
| Fig. 2 SEM of cellulose filter paper at (a) 10k×, (b) 20k× and (c) 50k× magnifications, PDA modified cellulose paper at (d) 10k×, (e) 20k× and (f) 50k× magnifications. | |
3.2. Optimization of TFME
The PDA-modified cellulose paper was used as an extraction phase, and TFME parameters such as desorption conditions, sodium hydroxide concentration and extraction time were studied and optimized. The type of desorption solvent has been shown to be an important factor influencing sample recovery, and organic solvents such as methanol, dichloromethane and acetonitrile have often been used in other investigations to elute adsorbed phenols.11 However, in the current work, these solvents showed poor eluting capability towards the studied phenols. The elution of phenols has also been performed with a mixture of ethanol and hydrochloric acid (HCl),39 so we in our current work tried methanol/HCl, dichloromethane/HCl and acetonitrile/HCl. The results indicated that acidification of these organic solvents by HCl significantly increased the recoveries of the two analytes. As shown in Fig. 3, we found that using acetonitrile/HCl as the eluent (especially 25 μL HCl in 2.5 mL acetonitrile) gave better elution performances for the two analytes. This phenomenon may be attributed to the presence of ion exchange between free chloride ions and the adsorbed phenol anions.39 So, a mixture of 25 μL HCl and 2.5 mL acetonitrile was chosen as the desorption solvent for the subsequent experiments.
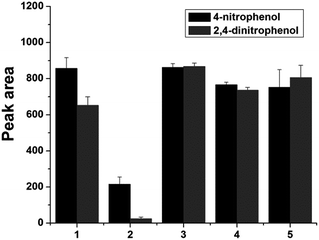 |
| Fig. 3 Effect of the type of desorption solvent on the extracted amounts of the phenolic compounds (1000 μL oil; concentration of phenolic compounds, 200 μg L−1; 9 mL n-hexane; 10 μL 2 mol L−1 NaOH; shaking rate, 150 rpm; extraction temperature, 30 °C; time of extraction, 150 min; volume of desorption, 1000 μL; time of desorption, 10 min). (1) 25 μL hydrochloric acid in 2.5 mL methanol, (2) 25 μL hydrochloric acid in 2.5 mL dichloromethane, (3) 25 μL hydrochloric acid in 2.5 mL acetonitrile, (4) 10 μL hydrochloric acid in 2.5 mL acetonitrile, (5) 50 μL hydrochloric acid in 2.5 mL acetonitrile. | |
In order to ensure that the two phenols were eluted from the PDA-modified cellulose paper completely and that no carryover occurred, different volumes of the solvent mixture, from 250 μL to 1500 μL, were examined. As can be seen in Fig. S2,† a volume of 1250 μL was sufficient for achieving a high extraction amount of the two analytes. However, when the volume of the desorption solvent was more than 1250 μL, the peak areas of the two analytes decreased obviously. Thus, a volume of 1250 μL was used in the rest of the study.
Effect of desorption time was also investigated in the range of 10–50 min. As shown in Fig. S3,† desorption effects were hardly influenced by increasing the desorption time, and a duration of 10 min was enough to elute the two phenols from the PDA-modified cellulose paper.
In general, aqueous NaOH solutions are used to separate phenols from oils. In this work, preliminary extraction experiments were carried out in the presence of small amounts of aqueous NaOH solutions. It was found that the peak areas of the two analytes were improved significantly with respect to those obtained in the absence of NaOH. Hence, the extraction was done by changing the volume of 2 mol L−1 NaOH from 0 to 70 μL, and Fig. 4 shows the effect of the volume of NaOH on the peak area. The highest analytical signals were observed in the case of 5 μL of NaOH. The presence of NaOH can promote the ionization of the weakly acidic phenols in oils. The phenol anions have a strong affinity for binding sites of the PDA-modified cellulose paper, which may further improve the enrichment capacities of the PDA-modified cellulose paper for the two analytes. However, increasing the volume of the NaOH aqueous solution to over 5 μL resulted in a rapid decrease of the peak areas of the two analytes. This rapid decrease may have occurred because the larger volume of aqueous NaOH could deposit tiny water droplets onto the bottom of the conical flasks. Many strongly polar phenol anions in such a case would easily enter into these alkaline water droplets, such as what occurs for reverse DLLME,40 leading to the much lower extraction efficiency. Therefore, a volume of 5 μL of 2 mol L−1 NaOH was used to achieve a high analytical sensitivity.
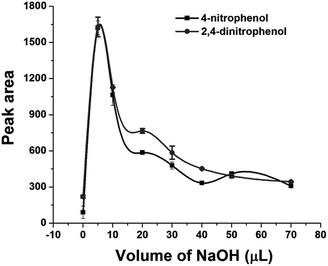 |
| Fig. 4 Effect of the volume of sodium hydroxide on the extracted amounts of the phenolic compounds (1000 μL oil; concentration of phenolic compounds, 200 μg L−1; 9 mL n-hexane; shaking rate, 150 rpm; extraction temperature, 30 °C; time of extraction, 150 min; desorption solvent, 25 μL hydrochloric acid in 2.5 mL acetonitrile; volume of desorption, 1250 μL; time of desorption, 10 min). | |
In addition, extraction time plays a very important role in the TFME process because the adsorbed amount of analyte always increases with extraction time until reaching an adsorption equilibrium.31 Different extraction times from 20 to 150 min were evaluated in this work (Fig. S4†). The peak area of each analyte greatly increased with the increase of extraction time and reached a maximum adsorption amount at 120 min. Therefore, a duration of 120 min was chosen as the optimum time for the extraction of the nitrophenols.
3.3. Analytical characteristics
In order to investigate the oil samples, the analytical characteristics of the proposed method were determined in terms of linearity, precision (expressed as the relative standard deviation), enrichment factor and limit of detection. All of the obtained results are summarized in Table 1. Calibration curves for 4-nitrophenol and 2,4-dinitrophenol were obtained in the concentration range of 5–1000 μg L−1. A good linearity with a correlation coefficient (r) greater than 0.9982 was obtained for each of the analytes. Precision was investigated by performing intraday and inter-day precision studies. The intra-day RSDs of the method varied from 3.07% to 3.37% and the inter-day RSDs obtained were in the range of 4.18–4.31% (n = 8). Enrichment factors were obtained by comparing the peak areas of the target analytes after pre-concentration with the peak areas of the target analytes for calibration curves without direct pre-concentration. The enrichment factor for each analyte was obtained by applying eight replicate extractions of oil samples spiked with 200 μg L−1 of the analyte (Table 1). Based on a signal-to-noise (S/N) ratio of 3, a limit of detection (LOD) of 1.54 μg L−1 was achieved for 4-nitrophenol and an LOD of 2.16 μg L−1 was achieved for 2,4-dinitrophenol. Compared with previous investigations for the detection of phenolic compounds,41,42 this work exhibited an acceptable analytical performance.
Table 1 Analytical performance of the method
Compound |
Linear range (μg L−1) |
Correlation coefficient (r) |
Precisiona (%) |
EFsb |
LODs (μg L−1) |
Intra-day |
Inter-day |
Relative standard deviation (n = 8). The mean values of the enrichment factors (n = 8). |
4-Nitrophenol |
5–1000 |
0.9998 |
3.37 |
4.18 |
157.41 |
1.54 |
2,4-Dinitrophenol |
5–1000 |
0.9982 |
3.07 |
4.31 |
170.97 |
2.16 |
3.4. Analysis of oil samples
The TFME-HPLC method proposed in this work was applied to quantitatively measure the concentration of phenolic compounds in the oil samples. The proposed extraction method was combined with HPLC-UV, and the procedure used for the extraction of the oil samples was the same as that used to analyze the standard solution. The oil samples spiked with concentrations of 80 μg L−1 and 200 μg L−1 of each target analyte were investigated, and the analytical results are summarized in Table 2. Relative recoveries obtained for oil samples varied from 84.37% to 100.85%. Fig. 5 shows the liquid chromatogram of the phenolic compounds in a vegetable oil before and after spiking at 80 μg L−1 and 200 μg L−1.
Table 2 Relative recoveries for oil sample analysis
Sample |
Relative recoverya (%) |
4-Nitrophenol |
2,4-Dinitrophenol |
Mean values ± standard deviation (n = 3). |
1# |
80 μg L−1 |
94.85 ± 8.00 |
84.37 ± 7.25 |
200 μg L−1 |
98.65 ± 6.29 |
90.88 ± 5.62 |
2# |
80 μg L−1 |
89.17 ± 3.51 |
85.07 ± 3.39 |
200 μg L−1 |
97.69 ± 3.11 |
98.66 ± 1.03 |
3# |
80 μg L−1 |
87.93 ± 2.86 |
100.47 ± 3.89 |
200 μg L−1 |
94.29 ± 0.85 |
100.85 ± 3.90 |
4# |
80 μg L−1 |
87.33 ± 3.99 |
99.37 ± 13.18 |
200 μg L−1 |
99.72 ± 1.28 |
93.07 ± 4.63 |
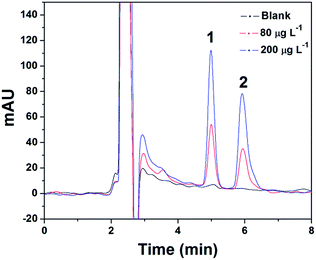 |
| Fig. 5 The chromatograms of a vegetable oil sample and spiked sample from the bottom up: blank, 80 μg L−1 and 200 μg L−1. (1) 4-Nitrophenol; (2) 2,4-dinitrophenol. | |
4. Conclusions
In this study, cellulose paper was successfully modified with polydopamine by using a simple, efficient and environmentally friendly approach for the first time. The plain and PDA-treated cellulose papers were characterized by EDS, FT-IR and SEM. The results demonstrated that the papers were indeed successfully modified by polydopamine. The PDA-modified cellulose paper was then introduced as a novel extracting phase for TFME. The proposed extraction method was combined with HPLC-UV to detect the phenolic compounds 4-nitrophenol and 2,4-dinitrophenol in oil samples, which showed that the PDA-modified cellulose paper has potentially great application in the pre-concentration of phenolic compounds from vegetable oil systems.
Acknowledgements
The financial support that was provided by the National Natural Science Foundation of China (No. 21107022) and that provided by the Key Scientific Research Project of Higher Education of Henan Province of China (No. 15A610007) are gratefully acknowledged.
Notes and references
- H. Tahermansouri, Z. Dehghan and F. Kiani, RSC Adv., 2015, 5, 44263–44273 RSC.
- P. Xu, G. M. Zeng, D. L. Huang, L. Liu, C. Lai, M. Chen, C. Zhang, X. X. He, M. Y. Lai and Y. B. He, RSC Adv., 2014, 4, 40828–40836 RSC.
- D. M. Nevskaia, E. Castillejos-Lopez, V. Munoz and A. Guerrero-Ruiz, Environ. Sci. Technol., 2004, 38, 5786–5796 CrossRef CAS PubMed.
- B. H. Hameed and A. A. Rahman, J. Hazard. Mater., 2008, 160, 576–581 CrossRef CAS PubMed.
- G. Favaro, D. de Leo, P. Pastore, F. Magno and A. Ballardin, J. Chromatogr. A, 2008, 1177, 36–42 CrossRef CAS PubMed.
- C. J. Liao, C. P. Chen, M. K. Wang, P. N. Chiang and C. W. Pai, Environ. Toxicol., 2006, 21, 71–79 CrossRef CAS PubMed.
- F. E. O. Suliman, S. S. Al-Kindi, S. M. Z. Al-Kindy and H. A. J. Al-Lawati, J. Chromatogr. A, 2006, 1101, 179–184 CrossRef CAS PubMed.
- Toxic Substance Control Act, US Environmental Protection Agency, Washington, DC, 1979 Search PubMed.
- Y. Wang, D. Wei, H. Yang, Y. Yang, W. Xing, Y. Li and A. Deng, Talanta, 2009, 77, 1783–1789 CrossRef CAS PubMed.
- F. J. López-Jiménez, S. Rubio and D. Pérez-Bendito, Food Chem., 2010, 121, 763–769 CrossRef.
- M. Raoov, S. Mohamad, M. R. bin Abas and H. Surikumaran, Talanta, 2014, 130, 155–163 CrossRef CAS PubMed.
- Z. Zhang, H. Zhang, Y. Hu and S. Yao, Anal. Chim. Acta, 2010, 661, 173–180 CrossRef CAS PubMed.
- J. Regueiro, E. Becerril, C. Garcia-Jares and M. Llompart, J. Chromatogr. A, 2009, 1216, 4693–4702 CrossRef CAS PubMed.
- W. Liu, W. Zhao, J. Chen and M. Yang, Anal. Chim. Acta, 2007, 605, 41–45 CrossRef CAS PubMed.
- C. Yu, Q. Liu, L. Lan and B. Hu, J. Chromatogr. A, 2008, 1188, 124–131 CrossRef CAS PubMed.
- Y. Fan, M. Chen, C. Shentu, F. El-Sepai, K. Wang, Y. Zhu and M. Ye, Anal. Chim. Acta, 2009, 650, 65–69 CrossRef CAS PubMed.
- M. M. Tian, D. X. Chen, Y. L. Sun, Y. W. Yang and Q. Jia, RSC Adv., 2013, 3, 22111–22119 RSC.
- A. Jain and K. K. Verma, Anal. Chim. Acta, 2011, 706, 37–65 CrossRef CAS PubMed.
- L. Pelit, İ. Bağatıra, F. O. Pelit and F. Nil Ertaş, RSC Adv., 2014, 4, 32189–32196 RSC.
- F. Aydin, E. Yilmaz and M. Soylak, RSC Adv., 2015, 5, 40422–40428 RSC.
- M. Shamsipur and B. Hashemi, RSC Adv., 2015, 5, 20339–20345 RSC.
- H. Jiang, B. Hu, B. Chen and W. Zu, Spectrochim. Acta, Part B, 2008, 63, 770–776 CrossRef.
- S. Jafarvand and F. Shemirani, J. Sep. Sci., 2011, 34, 455–461 CrossRef CAS PubMed.
- A. Ballesteros-Gomez, S. Rubio and D. Perez-Bendito, J. Chromatogr. A, 2009, 1216, 530–539 CrossRef CAS PubMed.
- S. Jafarvand and F. Shemirani, Anal. Methods, 2011, 3, 1552–1559 RSC.
- A. Moral, M. D. Sicilia and S. Rubio, J. Chromatogr. A, 2009, 1216, 3740–3745 CrossRef CAS PubMed.
- F. Rezaei, Y. Yaminia, M. Moradi and B. Daraei, Anal. Chim. Acta, 2013, 804, 135–142 CrossRef CAS PubMed.
- M. R. Moghadam, S. Dadfarnia and A. M. H. Shabani, J. Hazard. Mater., 2011, 186, 169–174 CrossRef CAS PubMed.
- S. Dadfarnia and A. M. H. Shabani, Anal. Chim. Acta, 2010, 658, 107–119 CrossRef CAS PubMed.
- J. Pawliszyn, Handbook of Solid Phase Microextraction, Chemical Industry Press of China, Beijing, China, 2009 Search PubMed.
- I. Bruheim, X. Liu and J. Pawliszyn, Anal. Chem., 2003, 75, 1002–1010 CrossRef CAS PubMed.
- S. Hong, K. Y. Kim, H. J. Wook, S. Y. Park, K. D. Lee, D. Y. Lee and H. Lee, Nanomedicine, 2011, 6, 793–801 CrossRef CAS PubMed.
- H. Lee, S. M. Dellatore, W. M. Miller and P. B. Messersmith, Science, 2007, 318, 426–430 CrossRef CAS PubMed.
- H. Lee, J. Rho and P. B. Messersmith, Adv. Mater., 2009, 21, 431–434 CrossRef CAS PubMed.
- S. Hong, Y. S. Na, S. Choi, I. T. Song, W. Y. Kim and H. Lee, Adv. Funct. Mater., 2012, 22, 4711–4717 CrossRef CAS.
- Q. Wei, F. Zhang, J. Li, B. Li and C. Zhao, Polym. Chem., 2010, 1, 1430–1433 RSC.
- Y. Gu and J. Huang, J. Mater. Chem., 2009, 19, 3764–3770 RSC.
- M. Abdelmouleh, S. Boufi, A. Ben Salah, M. N. Belgacem and A. Gandini, Langmuir, 2002, 18, 3203–3208 CrossRef CAS.
- F. Ferri, L. Bertin, A. Scoma, L. Marchetti and F. Fava, Chem. Eng. J., 2011, 166, 994–1001 CrossRef CAS.
- A. Spietelun, Ł. Marcinkowski, M. de la Guardia and J. Namieśnik, Talanta, 2014, 119, 34–45 CrossRef CAS PubMed.
- J. N. Sun, J. Chen and Y. P. Shi, Talanta, 2014, 125, 329–335 CrossRef CAS PubMed.
- Q. Z. Feng, L. X. Zhao and J. M. Lin, Anal. Chim. Acta, 2009, 650, 70–76 CrossRef CAS PubMed.
Footnote |
† Electronic supplementary information (ESI) available. See DOI: 10.1039/c5ra23232e |
|
This journal is © The Royal Society of Chemistry 2016 |
Click here to see how this site uses Cookies. View our privacy policy here.