DOI:
10.1039/C5RA26225A
(Paper)
RSC Adv., 2016,
6, 13399-13406
A novel microfluidic device that integrates nucleic acid extraction, amplification, and detection to identify an EGFR mutation in lung cancer tissues†
Received
8th December 2015
, Accepted 20th January 2016
First published on 25th January 2016
Abstract
The ability to detect gene mutations is critical for the development of individual therapeutic strategies to improve lung cancer patient survival rate. We developed an easy-to-use, easy-to-take, low-cost, pump-free, loop-mediated isothermal amplification (LAMP)-based, glass powder-assisted point-of-care testing (POCT) microfluidic device that can extract DNA from samples in a minute and can detect gene mutations with high sensitivity and high specificity in 40 min. Incorporation of the fluorescent dye calcein allows the presence of the mutation to be detected by the naked eye. Using this device, we detected the L858R mutation in the epidermal growth factor receptor (EGFRL858R) in lung adenocarcinoma NCI-H1975 cells, but not in A549 cells that do not carry the mutation. Moreover, the EGFRL858R mutation was detected in five clinical tissue specimens from lung adenocarcinoma patients using this device.
Introduction
Lung cancer has been widely recognized as a first incidence and mortality malignancy. Although targeted therapy technology has improved, the cure and 5 year survival rates are still very low. Studies have shown that early diagnosis and individualized treatment significantly improve the cure rate. The development of lung cancer is a multi-stage process including inactivation of tumor suppressor genes and activation of proto-oncogenes by multiple gene mutations.1,2 Studies have found that different lung cancer patients exhibited different therapeutic effects and adverse reactions (including fatality) to treatment with standard doses of the same drug due to their different genetic mutations.3,4 Therefore, individualized treatments are necessary.
Currently, individualized lung cancer treatments are based on detection of differential gene expression in patients.5–7 Mutations in epidermal growth factor receptor (EGFR) have been found in more than 30% of lung cancer patients, and mutations in EGFR have been identified as important driving factors for the development of cancer. Numerous clinical studies have shown that the presence of EGFR mutations can predict drug sensitivity in patients. Screening patients for EGFR mutations could allow for individualized targeted drug treatment.
EGFR is a cell proliferation and signaling receptor that is widely distributed in mammalian epithelial cells, the surface of fibroblasts, glial cells, and keratinocytes. The loss of EGFR or other key factors associated with signaling pathways significantly alters cell proliferation, invasion and metastasis of tumor cells, tumor angiogenesis, and apoptosis.8 EGFR mutations primarily occur in exons 18–21 with mutations in exons 19 and 21 comprising 90% of the mutations.9,10 Typically, to detect EGFR mutations, samples are obtained, DNA is extracted, and mutations are detected and analyzed by DNA sequencing, amplification-refractory mutation system (ARMS), denaturing high performance liquid chromatography (DHPLC), or by microarray.11–16 These methods do not meet the requirements for diagnostic tests in economically underdeveloped areas because the sensitivity limit is high and prone to false positive results, because the equipment is expensive, and because the methods are costly and time-consuming. The World Health Organization (WHO) developed guidelines for diagnostic tests appropriate for the developing world; these guidelines are summarized in the acronym ASSURED, which stands for affordable, sensitive, specific, user-friendly, rapid and robust, equipment-free, and deliverable to end users.17 A rapid clinical detection device, which can achieve point-of-care testing (POCT) for individualized lung cancer treatment, is needed.
Isothermal nucleic acid amplification technology including loop-mediated isothermal amplification (LAMP), helicase-dependent amplification, and rolling circle amplification have potential for POCT for EGFR mutations. LAMP is a relatively new gene amplification technique that was initially described in 2000. This technology utilizes Bst DNA polymerase with chain replacement activity and multiple specific primers sets that target genes in six regions.18,19 In LAMP, DNA amplification equals detection and no further analysis is required. In LAMP, the amplification reaction is performed at a constant temperature of 60–65 °C that requires only a water bath or incubator, and the results are determined by visualizing white turbidity or green fluorescence with the naked eye.20,21 The high specificity, high sensitivity, and fast and high output of LAMP make it suitable for rapid diagnosis at the grass-roots level. LAMP has been successfully used to detect SARS, avian flu, HIV, and other diseases.22–26 In this study, we further developed this method to detect the EGFRL858R point mutation. Despite the high sensitivity of this technique, there are significant problems with false positives because once a reaction tube is opened, it aerosolizes and pollutes the environment where the experiments are performed. Thus, there is an urgent need for development of a device that integrates nucleic acid extraction, isothermal amplification, and real-time detection of single nucleotide polymorphisms.
Microfluidic (lab-on-a-chip) technology permits the accommodation and control of microliter–picoliter amounts of samples on a device measuring a few square centimeters or smaller. Consequently, it miniaturizes basic conventional biological or chemical laboratory operations, such as sample preparation, reaction, separation, and detection.27 LAMP incorporates these advantages with some modifications. In our design, specific primers are required, enzyme and reaction buffers must be assembled in advance to improve the rate of nucleic acid synthesis, and chemically modified glass powder must be introduced prior to POCT gene amplification for nucleic acid adsorption onto the glass powder.28 Not only does microfluidic technology eliminate the need for centrifugation, electrophoresis, signal detection, and other methods that require large equipment, but it also allows for the reaction to occur in a controlled and confined space that is free from environmental contaminants. We developed an integrated POCT microfluidic device to detect the EGFRL858R point mutation in NCI-HI1975 cells; the cell line A549 was used as the negative control.29,30 Moreover, the EGFRL858R mutation was detected in five clinical tissue specimens from lung adenocarcinoma patients using this device.
Materials and methods
Cell culture and DNA extraction
The human non-small cell line NCI-HI1975 containing the EGFRL858R point mutation was used as the positive control and the human non-small cell line A549 without the EGFR mutation was used as the negative control. Cells were cultured at 37 °C with 5% CO2 in RMPI 1640 (Gibco, USA) supplemented with 10% fetal bovine serum and 100 U penicillin–streptomycin. Every 2–3 days, cells were detached from the surface of the culture flasks using 0.25% trypsin–EDTA, and logarithmic phase cells were used in the experiments. Genomic DNA was extracted using DNAiso Reagent (Takara, Dalian, China) and stored at −20 °C.
Sequencing
DNAs from the NCI-HI1975 and A549 cell lines were PCR amplified using a PCR amplification kit (TransGen, Beijing, China). Primers for the amplification of EGFRL858R were designed using Primer 5 software. Reaction mixtures contained 2 μl of template DNA, 25 μl of 2× EasyTaq®PCR Supermix, 1 μl of each primer (100 μM), and 21 μl of ddH2O in a total volume of 50 μl. Thermal cycling conditions were as follows: initial denaturation at 94 °C for 5 min, 30 cycles of 94 °C for 30 s, 55 °C for 30 s, and 72 °C for 20 s, and a final extension at 72 °C for 5 min. PCR products were purified using a TIAN quick Midi Purification Kit (TIANGEN, Beijing, China) and were sequenced using an ABI PRISM 3730 Genetic Analyzer® and ABI PRISM SeqScape Software Version 5.2.0®.
Primer design
To design primers for EGFR, the sequence of EGFR (NM_005228) and Primer Explorer V4 were utilized. The L858R mutation is a CTG to CGG mutation. Backward inner loop (BIP) primers were designed as the site-specific primers. The mutation was placed on the 5′ end of the BIP primer and was followed by a mismatch. The sequences of the four LAMP primers (Invitrogen, Life Technologies) and peptide nucleic acid (Search Co, Ltd, Beijing, China):
F3, GCAGGGTCTTCTCTGTTTCA;
B3, TCAGGAAAATGCTGGCTGAC;
FIP, TTCACCAGTACGTTCCTGGCTG–AACTACTTGGAGGACCGTCG;
BIP, GTGCCAAACTGCTGGGTGCGGA–CCACCTCCTTACTTTGCCTC;
PNA, GCCAGCCCAA.
The LAMP reaction
LAMP was performed using a Loopamp DNA amplification kit (Loopamp, Beijing, China) according to the manufacturer's instructions. The reaction contained 12.5 μl of reaction mix (RM: 40 mM Tris-HCL, 20 mM KCl, 16 mM MgSO4, 20 mM (NH)4SO4, 0.2%, Tween 20, 16 mM betaine, 2.8 mM dNTPs, 2 mM PNA), 1.0 μl of Bst DNA polymerase, 1 μl of calcein reagent, 2 μl of template DNA, 40 pmol of forward inner primer (FIP), 40 pmol of backward inner primer (BIP), 5 pmol of forward outer primer F3, 5 pmol of backward outer primer B3 in a total volume of 25 μl. LAMP reactions were assembled on ice and performed at 65 °C without denaturation at 94 °C. After 40 min, reactions were loaded onto 2% agarose gels, electrophoresed, and visualized with ultraviolet (UV) light.
Design of the microfluidic device
We designed a double-layered microfluidic device (Fig. 1A) that consisted of three parallel units enabling the positive control reaction, the negative control reaction, and the test reaction could be assayed on one chip. Each unit consisted of a balloon room (radius r = 8 mm), an amplification reagent storage zone, and a detection zone connected by channels. The amplification reagent storage zone included three linear wells: an isothermal amplification buffer chamber (r = 6 mm; containing dNTPs, MgSO4, Triton X-100, calcein, betaine, Tris–HCL, KCl and (NH)4SO4), a primer chamber (r = 3 mm), and a Bst DNA polymerase chamber (r = 2 mm). Modified-glass powder was placed into the detection chamber (r = 7.5 mm). A barrier was designed between the detection chamber and the inlet and outlet chambers, and the DNA was adsorbed onto the glass powder in the detection chamber. Microcolumns within the chambers prevented collapse upon pinching. The balloon and detection chambers each had nine microcolumns, and the reagent storage zone had a series of three groups of microcolumns, which had five, three, and one, respectively (Fig. 1C).
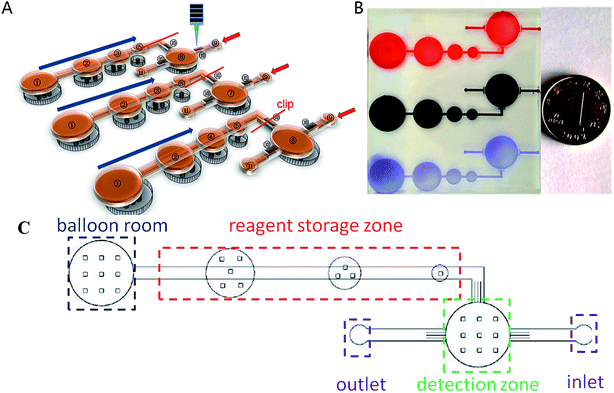 |
| Fig. 1 Design of the microfluidic device. (A) A schematic of the double-layered chip. (B) A photograph of the double-layered microfluidic device. (C) A vertical view of the microfluidic chip. | |
The microfluidic device (Fig. 1B) was fabricated using a 10
:
1 (w/w) mixture of polydimethylsiloxane (PDMS) and a curing agent (Sylgard184, Dow Corning, USA). The chip pattern was designed using FreeHand. The height of the wells in the upper and lower layer were 200 μm. The two layers were irreversibly bonded by exposure to oxygen plasma for ∼1 min. The isothermal amplification buffer, primers and probe, Bst DNA polymerase, and glass powder were quickly placed into the wells prior to bonding. The channel between the storage and detection chambers was clamped to prevent mixing. The entire process was performed in a clean room (cleanliness level > 1000) and the microfluidic devices were stored at −20 °C.
Glass powder preparation
Glass powder with an average diameter of 60 μm was cleaned in piranha solution (6
:
4 v/v 98% H2SO4
:
H2O2) for 45 min. Then, the glass powder was silanized in 2.5% (3-aminopropyl)triethoxysilane (in 95% ethanol) for 30 min after washing with ethanol several times. A carboxyl group was attached to the glass surface and the washed glass beads were immersed in 10% succinic anhydride (in N,N-dimethylformamide) for 6 h with gentle shaking.
DNA adsorption onto glass powder
Media was removed from cell cultures and samples were digested with 1 ml of DNAiso. Paraffin-embedded tissue sections were cut into chips (10–15 μm). The mixture of cell debris and DNAiso was slowly injected into each chip through the inlet. After 10 min, 95% ethanol and saturated sodium chloride (1
:
1 v/v) was injected into each chip to remove the DNAiso, and then a puff of air was injected to remove remaining ethanol and sodium chloride reagent. Then, 10 μl of SYBR Green was injected. The glass powder was visualized with UV light to check whether any DNA remained in the reaction chamber. All injections (liquid or air) were conducted with gentle force to avoid the generation of bubbles.
Processing the LAMP reaction through the microfluidic device
After the DNA sample was adsorbed onto the glass powder, the air chambers were squeezed to push the reaction mixture into the reaction chamber. The clip around the detection chamber was removed and the balloon room was squeezed to allow the stored reagents to flow into the detection chamber. To avoid generating bubbles, the reaction chamber was situated slightly lower than the air chamber during air chambers squeezing. Additionally, to prevent reflux of the liquid from the detection chamber and avoid introducing bubbles, the inlet and outlet were sealed with oils and the balloon room was clipped. The microfluidic device was incubated at 65 °C and the color of the reaction was analyzed to determine whether the mutation was present. A green color indicated the presence of the EGFR mutation and a yellow color indicated the absence of the mutation. The process of extruding the storage reagent into the reaction chamber has been captured in a movie (ESI†).
Clinical tissue specimens
Formalin-fixed paraffin-embedded tissue sections (5 mm) from lung adenocarcinoma patients were supplied by the Second Affiliated Hospital of Dalian Medical University (Dalian, China). All of the patients provided written informed consent.
Detection of mutations in clinical samples using the integrated microfluidic chip
Samples from lung adenocarcinoma patients at the Second Affiliated Hospital of Dalian Medical University were collected from April 2014 through February 2015. Samples were genetically tested for the EGFRL858R mutation, were lysed, and injected into reaction chambers. Products were examined with UV light.
Results and discussion
Validation of cell lines containing the EGFR mutation
DNA sequencing was used to determine whether NCI-HI1975 and A549 cells carried the L858R mutation. There was a clear point mutation (CTG → CGG) in NCI-HI1975 cells, but not in A549 cells (Fig. 2A and B). The LAMP assay was performed using NCI-HI1975 as the positive control cell line and A549 as the negative control cell line.
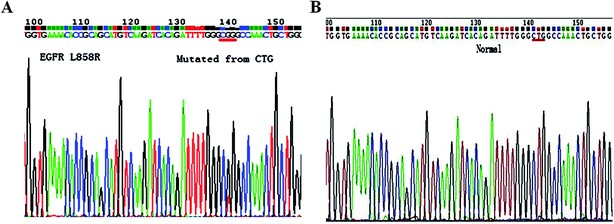 |
| Fig. 2 EGFR DNA sequences in NCI-H1975 (A) and A549 (B) cells. | |
LAMP primer verification
LAMP is highly specific and sensitive because it uses specific primers corresponding to six different regions on the target DNA and because the mutation is at the 5′ end (the 3′-end in the amplification direction) of the BIP. Thus, LAMP requires optimization of primer sets and reaction conditions. In our preliminary tests, twenty primer sets were designed for the allele-specific (AS) LAMP assay and were evaluated for their specificity and speed of amplification. Ultimately, we found two acceptable sets of primers and selected one set for further studies. The locations of the primers are shown in Fig. 3A.
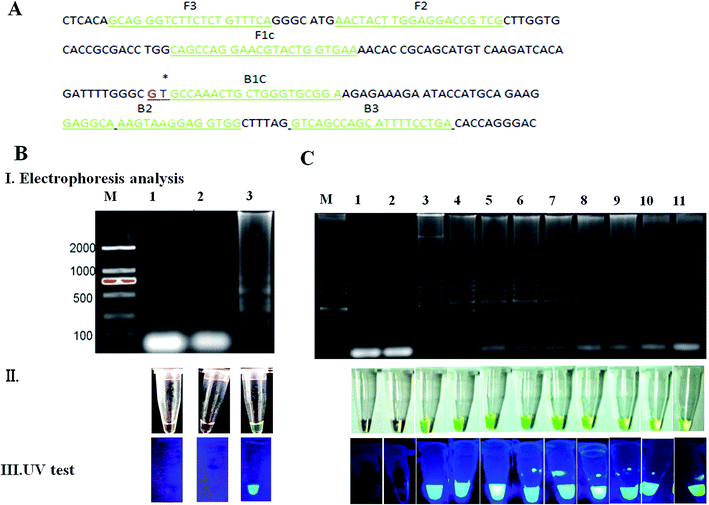 |
| Fig. 3 (A) The EGFR primer sets are indicated in green on the EGFR DNA sequence. The asterisk indicates the other mismatch. (B) Verification of the specificity of the primers. The upper panel shows the results of agarose gel electrophoresis of the LAMP reactions. (M) = 100bp DNA ladder, (1) = no DNA control, (2) = negative control DNA from A549 cells, and (3) = positive control DNA from NCI-H1975 cells. The middle panel shows the green color detectable with the naked eye for each of the reactions. The lower panel shows the fluorescence detectable with UV light for each of the reactions. (C) Sensitivity of LAMP for the detection of the EGFRL858R mutation. The upper panel shows the results of agarose gel electrophoresis of the LAMP reactions. (M) = 100bp DNA ladder, (1) = no DNA control, (2) = negative control DNA from A549 cells, and lanes (3–11) contain various amounts of positive control DNA from NCI-H1975 cells. Lane (3) = 100 ng μl−1, (4) = 10 ng μl−1, (5) = 1 ng μl−1, (6) = 100 pg μl−1, (7) = 10 pg μl−1, (8) = 1 pg μl−1, (9) = 100 fg μl−1, (10) = 10 fg μl−1, (11) = 1 fg μl−1. The middle panel shows the green color detectable with the naked eye for each of the reactions. The lower panel shows the fluorescence detectable with UV light for each of the reactions. | |
To verify the specificity of the primers, we compared a control reaction with no DNA (Fig. 3B upper panel lane (1)), a negative control reaction containing DNA from A549 cells (Fig. 3B upper panel lane (2)), and a positive control reaction containing DNA from NCI-H1975 cells (Fig. 3B upper panel lane (3)) by LAMP and agarose gel electrophoresis. Multiple bands were produced from the LAMP reaction containing the positive control, but not from the LAMP reactions with no DNA and with negative control DNA. The LAMP products were also detected macroscopically with the naked eye and with UV light. Macroscopically with the naked eye, a positive test produced a green color, and under UV light, a positive test exhibited green fluorescence (Fig. 3B middle and lower panels). These results demonstrate that the primers for the EGFRL858R mutation are specific.
To determine the detection limit of LAMP, we serially diluted the positive control DNA and used the dilutions in LAMP reactions. DNA dilutions ranged from 100 ng μl−1 to 1 fg μl−1. Products were visualized by agarose gel, macroscopically by eye, and by UV light in all the dilution reactions including the lowest 1 fg μl−1 reaction, but not in the negative control and no DNA control reactions (Fig. 3C). These results demonstrate that this LAMP reaction is not only highly specific, it is also highly sensitive and requires only minimal quantities of DNA.
Microfluidic usage
When the microfluidic is assembled, it is convenient to use because all of the LAMP reaction buffers are already in the chip. Consequently, one need only to inject the mixtures of samples and DNAiso and then squeeze the balloon-chambers. The most difficult step is the bonding of the two layers when assembling the chip. To facilitate this step, we recommended that the upper chambers be larger than the lower chambers (∼0.5 mm wider radius) to prevent the spread of buffer in the lower chambers.
Mixtures of cell debris and DNAiso were injected into the chips through the inlet. Then, 10 minutes later, saturated sodium chloride and ethanol (1
:
1, v/v) were injected to remove the DNAiso, and then a puff of air was injected to remove any remaining ethanol and sodium chloride reagent. Following the puff of air, the clips were removed and the balloon was pressed. Subsequently, all of the reaction buffer was extruded into the reaction chamber. To avoid generating bubbles, the reaction chamber was situated slightly lower than the air chamber during air chamber squeezing. In the event of any suck-back, the channel was re-clamped (clip site as in Fig. 1A), the entry and exit were sealed with oil, and the reaction was then started (Fig. 1). All injections (liquid or air) were conducted with gentle force to avoid the generation of bubbles.
The LAMP assay was performed on an integrated microfluidic device
We integrated the LAMP assay onto a microfluidic device. Lysis buffer, a A549 cell suspension, and a NCI-H1975 cell suspension were injected into the reaction chamber of a microfluidic device via the inlet. Products were visualized macroscopically by eye and by UV light. The reaction with the NCI-H1975 cell suspension turned green and fluoresced, whereas the lysis buffer and the A549 cell suspension reactions remained yellow and did not fluoresce (Fig. 4). This result demonstrates that our integrated microfluidic device detected the EGFRL858R mutation associated with lung cancer. Unlike conventional LAMP assays, this microfluidic-based method required only one syringe and an optional UV box since the results can also be visualized by the naked eye. In addition, this microfluidic device had a closed reaction space to eliminate environmental contamination, and reaction reagents were pre-added to the amplification reagent storage zone. Clamps prevented reagents in different chambers from mixing. All of these features meet the requirements of POCT.
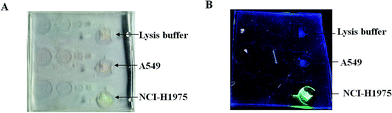 |
| Fig. 4 LAMP was performed on lysis buffer, A549 cell lysate, and NCI-H1975 cell lysate in the microfluidic device and reactions were visualized with the naked eye (A) and with UV light (B). | |
Detection of the EGFR mutation in clinical samples by integrated microfluidic chip
Tissue samples were obtained from 96 patients with surgically resected lung cancer at the Second Affiliated Hospital of Dalian Medical University from April 2014 to February 2015. Lung cancer was diagnosed by hematoxylin and eosin (HE) staining (Fig. 5A), and EGFR mutations were identified by direct DNA sequencing or ARMS-PCR. EGFR mutations were found in 41 of the 96 non-small cell lung cancer (NSCLC) patient samples (42.7%) and five of the patient samples contained the EGFRL858R mutation.
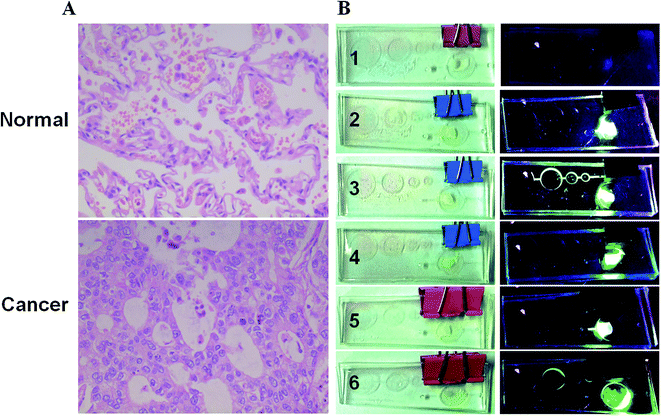 |
| Fig. 5 (A) HE staining of normal and cancerous lung tissue samples. (B) A tissue sample (#1) was identified as negative for the EGFRL858R mutation and five tissue samples (#2–6) were identified as positive for the EGFRL858R mutation using the integrated microfluidic device. Devices were visualized with the naked eye (images on the left) and with UV light (images on the right). | |
After tissue mixtures were injected into reaction chambers of the microfluidic devices and incubated for 25 min, tissues with the EGFRL858R mutation turned green and tissues with no or other mutations remained yellow (Fig. 5B).
Compared with the technical platforms of conventional LAMP testing, our microfluidic device has a number of advantages, such as being easy-to-use and easy-to-take, having greater sensitivity, and being less susceptible to contamination. This last advantage is particularly noteworthy given that there is broad agreement that contamination avoidance is critical for the LAMP reaction. These advantages can be attributed to the unique characteristics of the chip as well as to the design of the balloon chamber mode. As demonstrated, we chose a balloon room in which the reagent mixture is squeezed all at once. On the whole, microfluidics may be the most suitable technology, so far, for achieving the LAMP reaction successfully and for transferring all of the reagent buffer to the reaction site in a single action.
Conclusion
With the rapid development of mutation detection methods, many methods have been reported that detect EGFR mutations. These methods are often PCR and sequencing based.31,32 Some are more accurate and more sensitive than sequencing, but all are time-consuming, complex, and unsuitable for routine clinical examinations in poor, underdeveloped areas. We demonstrated that we could perform isothermal DNA amplification in a simple microfluidic chip containing glass powder to detect a single nucleotide polymorphism (SNP), EGFRL858R. This integrated device is ideal for POCT and does not require expensive equipment, such as centrifugal machines. Further, this is a fast, easy, inexpensive, highly sensitive, and accurate gene mutation detection device that has potential for multiple applications. Future studies will improve on the microfluidics and the site-specific LAMP primers to detect gene mutations. We are also improving the isothermal nucleic acid amplification methodology.33 This device integrates LAMP, glass powder, ARMS, and microfluidics to create an isothermal amplification method that is truly POCT.
Acknowledgements
The authors would like to thank Master Zhenlong Liang for his technical assistance and for supplying the glass powder. This work was supported in part by grants from the National Natural Science Foundation of China (#91129733 and #81330060), National High Technology Research and Development Program (863 Research Projects) of China (#2015AA020409) and the Opening Project of Zhejiang Provincial Top Key Discipline of Clinical Medicine (No. LKFyc05).
References
- J. G. Paez, P. A. Jänne, J. C. Lee, S. Tracy, H. Greulich, S. Gabriel, P. Herman, F. J. Kaye, N. Lindeman, T. J. Boggon, K. Naoki, H. Sasaki, Y. Fujii, M. J. Eck, W. R. Sellers, B. E. Johnson and M. Meyerson, EGFR Mutations in Lung Cancer: Correlation with Clinical Response to Gefitinib Therapy, Science, 2004, 304(5676), 1497–1500 CrossRef CAS PubMed
. - A. P. Feinberg, B. Vogelstein, M. J. Droller, S. B. Baylin and B. D. Nelkin, Mutation affecting the 12th amino acid of the c-Ha-ras oncogene product occurs infrequently in human cancer, Science, 1983, 220(4602), 1175–1177 CAS
. - L. Benesova, M. Pesek, B. Belsanova, P. Sekerka and M. Minarik, Denaturing capillary electrophoresis for automated detection of L858R mutation in exon 21 of the epidermal growth factor receptor gene in prediction of the outcome of lung cancer therapy, J. Sep. Sci., 2010, 33(15), 2349–2355 CrossRef CAS PubMed
. - T. J. Lynch, D. W. Bell, R. Sordella, S. Gurubhagavatula, R. A. Okimoto, B. W. Brannigan, P. L. Harris, S. M. Haserlat, J. G. Supko, F. G. Haluska, D. N. Louis, D. C. Christiani, J. Settleman and D. A. Haber, Activating mutations in the epidermal growth factor receptor underlying responsiveness of non-small-cell lung cancer to gefitinib, N. Engl. J. Med., 2004, 350(21), 2129–2139 CrossRef CAS PubMed
. - J. Ribeiro Gomes and M. R. Cruz, Combination of afatinib with cetuximab in patients with EGFR-mutant non-small-cell lung cancer resistant to EGFR inhibitors, OncoTargets Ther., 2015, 8, 1137–1142 CrossRef PubMed
. - D. Ercan, H. G. Choi, C. H. Yun, M. Capelletti, T. Xie, M. J. Eck, N. S. Gray and P. A. Jänne, EGFR Mutations and Resistance to Irreversible Pyrimidine-Based EGFR Inhibitors, Clin. Cancer Res., 2015, 21(17), 3913–3923, DOI:10.1158/1078-0432.CCR-14-2789
. - E. Sugiyama, S. Umemura, S. Nomura, K. Kirita, S. Matsumoto, K. Yoh, S. Niho, H. Ohmatsu, M. Tsuboi, Y. Ohe and K. Goto, Impact of single nucleotide polymorphisms on severe hepatotoxicity induced by EGFR tyrosine kinase inhibitors in patients with non-small cell lung cancer harboring EGFR mutations, Lung Cancer, 2015, 90(2), 307–313, DOI:10.1016/j.lungcan.2015.08.004
. - D. Veale, T. Ashcroft, C. Marsh, G. J. Gibson and A. L. Harris, Epidermal growth factor receptors in non-small cell lung cancer, Br. J. Cancer, 1987, 55(5), 513–516 CrossRef CAS PubMed
. - D. de Biase, M. Visani, U. Malapelle, F. Simonato, V. Cesari, C. Bellevicine, A. Pession, G. Troncone, A. Fassina and G. Tallini, Next-generation sequencing of lung cancer EGFR exons 18–21 allows effective molecular diagnosis of small routine samples (cytology and biopsy), PLoS One, 2013, 8(12), e83607 Search PubMed
. - M. Locatelli-Sanchez, S. Couraud, D. Arpin, R. Riou, P. P. Bringuier and P. J. Souquet, Routine EGFR molecular analysis in non-small-cell lung cancer patients is feasible: exons 18–21 sequencing results of 753 patients and subsequent clinical outcomes, Lung, 2013, 191(5), 491–499 CrossRef CAS PubMed
. - Y. Shi, J. Li, S. Zhang, M. Wang, S. Yang, N. Li, G. Wu, W. Liu, G. Liao, K. Cai, L. Chen, M. Zheng, P. Yu, X. Wang, Y. Liu, Q. Guo, L. Nie, J. Liu and X. Han, Molecular Epidemiology of EGFR Mutations in Asian Patients with Advanced Non-Small-Cell
Lung Cancer of Adenocarcinoma Histology – Mainland China Subset Analysis of the PIONEER study, PLoS One, 2015, 10(11), e0143515 Search PubMed
. - F. Oshita, S. Matsukuma, M. Yoshihara, Y. Sakuma, N. Ohgane, Y. Kameda, H. Saito, K. Yamada, E. Tsuchiya and Y. Miyagi, Novel heteroduplex method using small cytology specimens with a remarkably high success rate for analysing EGFR gene mutations with a significant correlation to gefitinib efficacy in non-small-cell lung cancer, Br. J. Cancer, 2006, 95(8), 1070–1075 CrossRef CAS PubMed
. - G. Ellison, E. Donald, G. McWalter, L. Knight, L. Fletcher, J. Sherwood, M. Cantarini, M. Orr and G. Speake, A comparison of ARMS and DNA sequencing for mutation analysis in clinical biopsy samples, J. Exp. Clin. Cancer Res., 2010, 6(29), 132 CrossRef PubMed
. - Z. Li, L. Jiang, H. Bai, Z. Wang, J. Zhao, J. Duan, X. Yang, T. An and J. Wang, Prevalence and clinical significance of BRAF V600E in Chinese patients with lung adenocarcinoma, Thorac. Cancer, 2015, 6(3), 269–274 CrossRef CAS PubMed
. - L. Xue, J. J. Fei, Y. Song, R. H. Xu and Y. J. Bai, Visual DNA microarray for detection of epidermal growth factor receptor (EGFR) gene mutations, Scand. J. Clin. Lab. Invest., 2014, 74(8), 693–699 CrossRef CAS PubMed
. - J. Liu, J. Shen, C. Yang, P. He, Y. Guan, W. Liang and J. He, High incidence of EGFR mutations in pneumonic-type non-small cell lung cancer, Medicine, 2015 Feb, 94(8), e540 Search PubMed
. - P. Yager, G. J. Domingo and J. Gerdes, Point-of-Care Diagnostics for Global Health, Annu. Rev. Biomed. Eng., 2008, 10, 107–144 CrossRef CAS PubMed
. - T. Notomi, H. Okayama, H. Masubuchi, T. Yonekawa, K. Watanabe, N. Amino and T. Hase, Loop-mediated isothermal amplification of DNA, Nucleic Acids Res., 2000, 28(12), E63 CrossRef CAS PubMed
. - T. Notomi, Y. Mori, N. Tomita and H. Kanda, Loop-mediated isothermal amplification (LAMP): principle, features, and future prospects, J. Microbiol., 2015, 53(1), 1–5 CrossRef CAS PubMed
. - Y. Mori, K. Nagamine, N. Tomita and T. Notomi, Detection of loop-mediated isothermal amplification reaction by turbidity derived from magnesium pyrophosphate formation, Biochem. Biophys. Res. Commun., 2001, 289(1), 150–154 CrossRef CAS PubMed
. - N. Tomita, Y. Mori, H. Kanda and T. Notomi, Loop-mediated isothermal amplification (LAMP) of gene sequences and simple visual detection of products, Nat. Protoc., 2008, 3(5), 877–882 CrossRef CAS PubMed
. - L. L. Poon, C. S. Leung, M. Tashiro, K. H. Chan, B. W. Wong, K. Y. Yuen, Y. Guan and J. S. Peiris, Rapid detection of the severe acute respiratory syndrome (SARS) coronavirus by a loop-mediated isothermal amplification assay, Clin. Chem., 2004, 50(6), 1050–1052 CAS
. - M. Nakauchi, I. Takayama, H. Takahashi, M. Tashiro and T. Kageyama, Development of a reverse transcription loop-mediated isothermal amplification assay for the rapid diagnosis of avian influenza A (H7N9) virus infection, J. Virol. Methods, 2014, 204, 101–104 CrossRef CAS PubMed
. - D. L. Rudolph, V. Sullivan, S. M. Owen and K. A. Curtis, Detection of Acute HIV-1 Infection by RT-LAMP, PLoS One, 2015, 10(5), e0126609 Search PubMed
. - I. Nishino, J. Fu, K. Tanji, T. Yamada, S. Shimojo, T. Koori, M. Mora, J. E. Riggs, S. J. Oh, Y. Koga, C. M. Sue, A. Yamamoto, N. Murakami, S. Shanske, E. Byrne, E. Bonilla, I. Nonaka, S. DiMauro and M. Hirano, Primary LAMP-2 deficiency causes X-linked vacuolar cardiomyopathy and myopathy (Danon disease), Nature, 2000, 406(6798), 906–910 CrossRef CAS PubMed
. - A. Badolo, K. Okado, W. M. Guelbeogo, H. Aonuma, H. Bando, S. Fukumoto, N. Sagnon and H. Kanuka, Development of an allele-specific, loop-mediated, isothermal amplification method (AS-LAMP) to detect the L1014F kdr-w mutation in Anopheles gambiae s. l., Malar. J., 2012, 11, 227 CrossRef CAS PubMed
. - Z. Xu, Y. Gao, Y. Hao, E. Li, Y. Wang, J. Zhang, W. Wang, Z. Gao and Q. Wang, Application of a microfluidic chip-based 3D co-culture to test drug sensitivity for individualized treatment of lung cancer, Biomaterials, 2013, 34, 4109–4117 CrossRef CAS PubMed
. - R. Cao, D. Duan, L. Jiang, Z. Lu, F. Bao, K. Zheng and J. Li, Polysaccharide-coated beads platform for biomolecule analysis: evolution of SiO2-based suspension arrays, Carbohydr. Polym., 2011, 83, 818–823 CrossRef CAS
. - H. Li, G. Schmid-Bindert, D. Wang, Y. Zhao, X. Yang, B. Su and C. Zhou, Blocking the PI3K/AKT and MEK/ERK signaling pathways can overcome gefitinib-resistance in non-small cell lung cancer cell lines, Adv. Med. Sci., 2011, 56(2), 275–284 CrossRef CAS PubMed
. - S. Simonetti, M. A. Molina, C. Queralt, I. de Aguirre, C. Mayo, J. Bertran-Alamillo, J. J. Sanchez, J. L. Gonzalez-Larriba, U. Jimenez, D. Isla, T. Moran, S. Viteri, C. Camps, R. Garcia-Campelo, B. Massuti, S. Benlloch, S. Ramon y Cajal, M. Taron and R. Rosell, Detection of EGFR mutations with mutation-specific antibodies in stage IV non-small-cell lung cancer, J. Transl. Med., 2010, 8, 135 CrossRef PubMed
. - Q. Pan, W. Pao and M. Ladanyi, Rapid Polymerase Chain Reaction-Based Detection of Epidermal Growth Factor Receptor Gene Mutations in Lung Adenocarcinomas, J. Mol. Diagn., 2005, 7(3), 396–403 CrossRef CAS PubMed
. - H. Asano, S. Toyooka, M. Tokumo, K. Ichimura, K. Aoe, S. Ito, K. Tsukuda, M. Ouchida, M. Aoe, H. Katayama, A. Hiraki, K. Sugi, K. Kiura, H. Date and N. Shimizu, Detection of EGFR gene mutation in lung cancer by mutant-enriched polymerase chain reaction assay, Clin. Cancer Res., 2006, 12(1), 43–48 CrossRef CAS PubMed
. - K. Hoshi, H. Takakura, Y. Mitani, K. Tatsumi, N. Momiyama, Y. Ichikawa, S. Togo, T. Miyagi, Y. Kawai, Y. Kogo, T. Kikuchi, C. Kato, T. Arakawa, S. Uno, P. E. Cizdziel, A. Lezhava, N. Ogawa, Y. Hayashizaki and H. Shimada, Rapid detection of epidermal growth factor receptor mutations in lung cancer by the Smart-Amplification Process, Clin. Cancer Res., 2007, 13(17), 4974–4983 CrossRef CAS PubMed
.
Footnotes |
† Electronic supplementary information (ESI) available. See DOI: 10.1039/c5ra26225a |
‡ These authors contributed equally to this work. |
|
This journal is © The Royal Society of Chemistry 2016 |
Click here to see how this site uses Cookies. View our privacy policy here.