DOI:
10.1039/C6RA00318D
(Communication)
RSC Adv., 2016,
6, 15180-15183
Phosphonoselenoic acid esters from the reaction between phosphoroselenoyl chlorides and Grignard reagents: synthetic and stereochemical aspects†
Received
6th January 2016
, Accepted 26th January 2016
First published on 28th January 2016
Abstract
Substitution reactions between a phosphoroselenoyl chloride and Grignard reagents proceeded smoothly at the phosphorus atom to furnish phosphonoselenoic acid esters. The resulting mixture of diastereoisomers could be readily distinguished on the basis of 31P NMR, and separated by recrystallization or column chromatography to afford diastereoisomerically enriched products. For one of these diastereoisomers, the absolute configuration was determined by carrying out a single-crystal X-ray diffraction analysis.
Due to the importance of binaphthyl-substituted phosphoric acids,1 esters,2 and amides,3 as well as their reduced trivalent phosphorus analogues that serve as organocatalysts and optically active ligands, many such derivatives with a wide range of substituents have been reported. In contrast, substantially less progress has been achieved for the corresponding phosphonic acids and their binaphthyl-containing derivatives. In 1993, Fuji and co-workers reported a phosphonic acid ester with a binaphthyl group and a methoxycarbonylmethyl group that functioned as an Horner–Wadsworth–Emmons reagent for the discrimination of enantiotopic carbonyl groups.4 Although the resulting chiral phosphonate5 and its analogues were used in the syntheses of natural products,6 only a few other derivatives are known, which is predominantly due to a lack of synthetic methods. Previously reported methods for the synthesis of phosphonic acid esters first involve a nucleophilic attack of a carbon compound on a semi-labile trivalent phosphorus compound containing a binaphthyl group, followed by oxidation of resulting phosphorus compounds.7 Even though the reaction of electrophilic pentavalent phosphorus compounds with carbon nucleophiles, such as the readily available Grignard reagents, should offer a straightforward synthetic alternative, reports on this type of reaction remain elusive, to the best of our knowledge. During the course of our studies on compounds with C
Se,8 P
S,9 and P
Se groups,10 we discovered a high propensity for P–Cl bonds in the presence of a P
Se group to cleave homolytically.10 Herein, we report substitution reactions of binaphthyl-containing phosphoryl chlorides and their sulfur and selenium isologues with Grignard reagents, as well as the discrimination of the resulting diastereoisomers using NMR spectroscopy. Moreover, the conversions of the resulting compounds to optically active compounds are described.
Initially, binaphthyl-containing phosphoryl chloride 1a was treated with methylmagnesium bromide (2a) in THF, but the desired product (3a) was neither formed at room temperature (Table 1, entry 1) nor under reflux conditions (entry 2). We then attempted to use phosphorothionyl (1b) and phosphoroselenoyl chlorides (1c),10 as we expected a higher reactivity for these reactants with 2a (entries 3 and 4). Interestingly, while chloride 1b did not react with 2a, the reaction of 1c with 2a afforded the desired product (5a) with a moderate yield (58%). The yield of 5a could be further improved by adjusting the solvent and the reaction temperature, and the reaction in toluene at 40 °C generated 5a with a 76% isolated yield (entry 5). To confirm the reactivity of 1a–1c towards aromatic Grignard reagents, 4-methylphenylmagnesium bromide (2b) was used under similar conditions as were used for the reaction with 2a (entries 6–9). Similar to the previous results, chloride 1c afforded the corresponding product (5b) selectively in high yield (entry 9).
Table 1 Reaction of phosphoryl or chalcogenophosphoryl chlorides with Grignard reagentsa
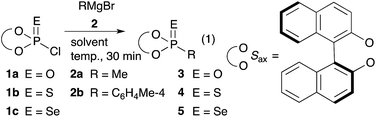
|
Entry |
Chloride, E |
RMgBr, R |
Solvent |
Temp. |
Product, E |
Yieldb,c (%), R |
Unless otherwise noted, chlorides 1 were treated with Grignard reagents (1.0 equivalents). Isolated yields. Percentages of the intensity of the signals corresponding to the desired products relative to all the signals observed in the 31P NMR spectra are shown in parentheses. |
1 |
1a |
O |
2a |
Me |
THF |
rt |
3a |
O |
Me |
0 |
2 |
1a |
O |
2a |
Me |
THF |
Reflux |
3a |
O |
Me |
(13) |
3 |
1b |
S |
2a |
Me |
THF |
Reflux |
4a |
S |
Me |
0 |
4 |
1c |
Se |
2a |
Me |
THF |
Reflux |
5a |
Se |
Me |
58 |
5 |
1c |
Se |
2a |
Me |
Toluene |
40 °C |
5a |
Se |
Me |
76 |
6 |
1a |
O |
2b |
C6H4Me-4 |
Toluene |
40 °C |
3b |
O |
C6H4Me-4 |
(41) |
7 |
1a |
O |
2b |
C6H4Me-4 |
Toluene |
Reflux |
3b |
O |
C6H4Me-4 |
(35) |
8 |
1b |
S |
2b |
C6H4Me-4 |
Toluene |
40 °C |
4b |
S |
C6H4Me-4 |
0 |
9 |
1c |
Se |
2b |
C6H4Me-4 |
Toluene |
40 °C |
5b |
Se |
C6H4Me-4 |
82 |
In order to understand the differences between the reactivities of 1a–1c, DFT calculations were carried out at the B3LYP/6-31G(d,p)11 level of theory. A comparison of the energy levels of the σ* orbitals of the P–Cl and P = E (E = O, S, Se) bonds revealed the LUMO+1 and LUMO+2 of the P
Se and P–Cl bonds to lie at lower energy levels than those of the P
O and P
S bonds. (For details on these energy levels, orbital shapes and NPA charge distributions, see Table S4 and Fig. S1†).
To further demonstrate the general utility of 1c in the synthesis of phosphonoselenoic acid esters containing a binaphthyl group, a range of Grignard reagents were tested (Table 2). The reaction with primary alkyl Grignard reagents furnished the corresponding products in good to very good yields (entries 1–5). During these reactions, we observed the phosphorodiselenoic acid ester 6 as a minor by-product (entry 1), the formation of which was ascribed to the selenophilic attack of the Grignard reagents12 on 1c, followed by the selenation of the intermediate as shown in Scheme 1. The formation of 6 could be prevented by adding DMAP, and the efficiency of the substitution reaction at the phosphorus atom could thus be enhanced (entry 4). DMAP may coordinate to the phosphorus atom of 1c in the same manner as in the reaction of pyridine with chlorophosphonates,13 and this coordination may reduce the electrophilicity of the selenium atom of 1c. Alternatively, the nuclephilicity of the Grignard reagents may be enhanced by the coordination of DMAP to the magnesium atom.
Table 2 Reaction of phosphoroselenoyl chloride 1c with Grignard reagentsa
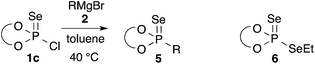
|
Entry |
RMgBr |
Product |
Yieldb (%) |
2 |
R |
5 |
R |
Unless otherwise noted, 1c was treated with Grignard reagents (1.0 equivalents). Isolated yields. Byproduct 6 was obtained in 5% yield. RMgCl was used. DMAP (2 equiv.) was used as an additive. |
1 |
2c |
Et |
5c |
Et |
72c |
2 |
2d |
CH2Ph |
5d |
CH2Ph |
83 |
3 |
2e |
CH2CH2Ph |
5e |
CH2CH2Ph |
79 |
4 |
2fd |
CH2SiMe3 |
5f |
CH2SiMe3 |
66e |
5 |
2g |
CH2Bu-t |
5g |
CH2Bu-t |
66 |
6 |
2h |
Cyclohexyl |
5h |
Cyclohexyl |
69 |
7 |
2id |
tert-Butyl |
5i |
tert-Butyl |
87 |
8 |
2j |
1-Adamantyl |
5j |
1-Adamantyl |
63 |
9 |
2k |
Allyl |
5k |
Allyl |
39 |
10 |
2l |
C6H4OMe-4 |
5l |
C6H4OMe-4 |
65e |
11 |
2m |
C6H4F-4 |
5m |
C6H4F-4 |
69e |
12 |
2n |
C6H4CF3-4 |
5n |
C6H4CF3-4 |
83e |
13 |
2o |
C6H4OMe-2 |
5o |
C6H4OMe-2 |
45e |
14 |
2p |
2-Naphtyl |
5p |
2-Naphtyl |
82 |
15 |
2q |
Ethyl-1,3-dioxlane |
5q |
Ethyl-1,3-dioxlane |
64 |
 |
| Scheme 1 Plausible reaction pathway to 6. | |
Secondary, tertiary, and allyl Grignard reagents also participated in the addition reaction with 1c, and afforded the corresponding products (5h–5k) in moderate to good yields (entries 6–9). In the reaction with allyl Grignard, reaction product 5k was found to compete for the allyl Grignard reagent, leading to the formation of the corresponding compound with three allyl groups at the phosphorus atom. In the reactions with aromatic Grignard reagents, the use of DMAP facilitated the substitution reaction at the phosphorus atom on 1c to give the corresponding products in good yields (entries 10–13).
To determine the diastereoselectivity of the reaction of 1c with racemic Grignard reagents, 1c was treated with sec-butylmagnesium bromide (2r), sec-decylmagnesium bromide (2s), and 2-ethylhexylmagnesium bromide (2t) (Scheme 2).
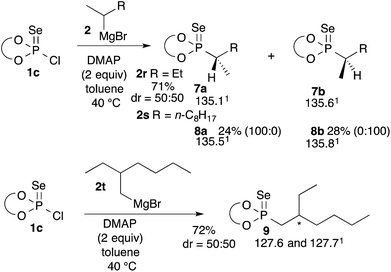 |
| Scheme 2 phosphonoselenoic acid esters 7–9. Values with superscript 1 correspond to chemical shifts (ppm) in the 31P NMR. | |
Although no selectivity was observed for these reactions, two diastereoisomers could be readily distinguished by 31P NMR in all cases. The separation of two diastereoisomers 7a and 7b, obtained from the reaction with 2r, was accomplished by recrystallization to afford diasteroisomerically enriched fractions (Scheme 3).
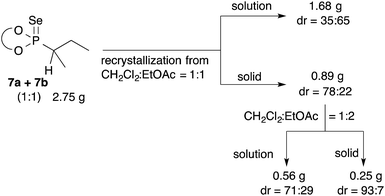 |
| Scheme 3 Separation of a diastereoisomer of 7 by recrystallization. | |
A single-crystal X-ray diffraction analysis of one of the diastereoisomers, corresponding to the 31P NMR signal at δ 135.1, was carried out, and revealed unequivocally an absolute S configuration for the carbon atom adjacent to the phosphorus atom (Fig. 1).
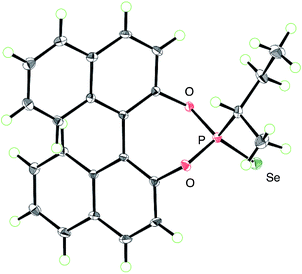 |
| Fig. 1 Molecular structure of Sax,S-7a with atomic displacement parameters set to 50% probability; only selected atoms are labeled. | |
During the purification of the products 8a and 8b, fractions involving one of the diastereoisomers were collected to afford 8a and 8b with high diastereoselectivity. The primary Grignard reagent 2s, containing a chiral center at the β-position relative to the magnesium atom, also engaged in addition reactions with 1c. Again, no diastereoselectivity was observed, but two diastereoisomers could be distinguished by 31P NMR.14 This observation is quite remarkable, considering that the discrimination of diastereoisomers is usually not an easy task when the chiral centers are located far from the chiral moieties.15
Finally the conversion of the resulting phosphonoselenoic acid esters was examined. The abstraction of the selenium atom from 5j and that from 7 were each accomplished by a treatment with PBu3 (Scheme 4). Optically active phosphonite 10
16 was successfully isolated, and was found to be stable under atmospheric conditions. In contrast, the initial product derived from 7 was found to be air sensitive and was hence immediately treated with BH3·THF to give 11, which is a rare example of a phosphonite–borane.9,17
 |
| Scheme 4 Conversion of phosphonoselenoic acid ester 5j or 7 to phosphonite 10 and phosphonite–borane 11. | |
Conclusions
In summary, we have demonstrated highly efficient substitution reactions of a binaphthyl-substituted phosphoroselenoyl chloride with Grignard reagents. The reactivity difference between selenoyl chloride and the corresponding phosphoryl and thionyl chlorides was rationalized on the basis of DFT calculations. The results showed the energy levels of the unoccupied molecular orbitals corresponding to the P–Cl and P
Se bonds to be lower than those of the phosphoryl and thionyl chlorides. Successful reactions of phosphoroselenoyl chlorides with a range of aliphatic, allylic and aromatic Grignard reagents demonstrated the broad synthetic utility of this reaction. The substitution reactions afforded mixtures of diastereoisomeric products, which could be readily distinguished by 31P NMR spectroscopy. The purification and separation of these isomer mixtures were accomplished by either recrystallization or column chromatography, and led to diastereoisomerically enriched products. Further studies on the applications of such phosphonic acids and their chalcogen isologues will be reported elsewhere in due course.
Acknowledgements
This work was partially supported by Grants-in-Aid for Scientific Research in Priority Areas ‘‘Stimuli-responsive Chemical Species for the Creation of Functional Molecules’’ from the MEXT.
Notes and references
- For recent reviews, see:
(a) T. Akiyama, Chem. Rev., 2007, 107, 5744 CrossRef CAS PubMed;
(b) M. Terada, Chem. Commun., 2008, 4097 RSC;
(c) D. Parmar, E. Sugiono, S. Raja and M. Rueping, Chem. Rev., 2014, 114, 9047 CrossRef CAS PubMed.
- For recent examples, see:
(a) H. Nakatsuji, Y. Sawamura, A. Sakakura and K. Ishihara, Angew. Chem., Int. Ed., 2014, 53, 6974 CrossRef CAS PubMed;
(b) M. Sohail, Y. Zhang, W. Liu, Q. Chen, L. Wang and Z. K. Zhao, RSC Adv., 2015, 5, 17014 RSC;
(c) Y. Senda, K. Nakajima and Y. Nishibayashi, Angew. Chem., Int. Ed., 2015, 54, 4060 CrossRef CAS PubMed;
(d) L. Pignataro, D. Fiorito, V. Vece, R. Ferraccioli and C. Gennari, Eur. J. Org. Chem., 2015, 6669 CrossRef CAS;
(e) J. Zhang, S.-X. Lin, D.-J. Cheng, X.-Y. Liu and B. Tan, J. Am. Chem. Soc., 2015, 137, 14039 CrossRef CAS PubMed.
- For recent examples, see:
(a) J. H. Kim, I. Coric, C. Palumbo and B. List, J. Am. Chem. Soc., 2015, 137, 1778 CrossRef CAS PubMed;
(b) C. Recsei and C. S. P. McErlean, Aust. J. Chem., 2015, 68, 555 CrossRef CAS;
(c) Z. Wang, F. K. Sheong, H. H. Y. Sung, I. D. Williams, Z. Lin and J. Sun, J. Am. Chem. Soc., 2015, 137, 5895 CrossRef CAS PubMed;
(d) S.-B. Yan, S. Zhang and W.-L. Duan, Org. Lett., 2015, 17, 2458 CrossRef CAS PubMed;
(e) M. Sai and H. Yamamoto, J. Am. Chem. Soc., 2015, 137, 7091 CrossRef CAS PubMed;
(f) G. C. Tsui, L. Luping and B. List, Angew. Chem., Int. Ed., 2015, 54, 7703 CrossRef CAS PubMed;
(g) J.-S. Lin, P. Yu, L. Huang, P. Zhang, B. Tan and X.-Y. Liu, Angew. Chem., Int. Ed., 2015, 54, 7847 CrossRef CAS PubMed;
(h) B.-M. Yang, P.-J. Cai, Y.-Q. Tu, Z.-X. Yu, Z.-M. Chen, S.-H. Wang, S.-H. Wang and F.-M. Zhang, J. Am. Chem. Soc., 2015, 137, 8344 CrossRef CAS PubMed;
(i) C.-Y. Liu and F.-S. Han, Chem. Commun., 2015, 51, 11844 RSC;
(j) J.-H. Tay, A. J. Arguelles and P. Nagorny, Org. Lett., 2015, 17, 3774 CrossRef CAS PubMed;
(k) L. Liu, M. Leutzsch, Y. Sheng, M. W. Alachraf, W. Thiel and B. List, J. Am. Chem. Soc., 2015, 137, 13268 CrossRef CAS PubMed;
(l) M. Hatano, Y. Goto, A. Izumiseki, M. Akakura and K. Ishihara, J. Am. Chem. Soc., 2015, 137, 13472 CrossRef CAS PubMed.
- K. Tanaka, Y. Ohta and K. Fuji, Tetrahedron Lett., 1993, 34, 4071 CrossRef CAS.
-
(a) K. Tanaka, K. Otsubo and K. Fuji, Tetrahedron Lett., 1996, 21, 3735 CrossRef;
(b) K. Tanaka, T. Watanabe, Y. Ohta and K. Fuji, Tetrahedron Lett., 1997, 38, 8943 CrossRef CAS;
(c) K. Tanaka, T. Watanabe, K. Shimamoto, P. Sahakitpichan and K. Fuji, Tetrahedron Lett., 1999, 40, 6599 CrossRef CAS;
(d) J. Yamazaki, T. Watanabe and K. Tanaka, Tetrahedron: Asymmetry, 2001, 12, 669 CrossRef CAS;
(e) A. V. Bedekar, T. Watanabe, K. Tanaka and K. Fuji, Tetrahedron: Asymmetry, 2002, 13, 721 CrossRef CAS;
(f) J. Yamazaki, A. V. Bedekar, T. Watanabe, K. Tanaka, J. Watanabe and K. Fuji, Tetrahedron: Asymmetry, 2002, 13, 729 CrossRef CAS.
- For recent examples, see:
(a) E. A. Crane, T. P. Zabawa, R. L. Farmer and K. A. Scheidt, Angew. Chem., Int. Ed., 2011, 50, 9112 CrossRef CAS PubMed;
(b) G. E. Keck, Y. B. Poudel, T. J. Cummins, A. Rudra and J. A. Covel, J. Am. Chem. Soc., 2011, 133, 744 CrossRef CAS PubMed;
(c) P. A. Wender and A. J. Schrier, J. Am. Chem. Soc., 2011, 133, 9228 CrossRef CAS PubMed;
(d) Y. Lu, S. K. Woo and M. J. Krische, J. Am. Chem. Soc., 2011, 133, 13876 CrossRef CAS PubMed;
(e) M. O'Brien and E. J. Thomas, Tetrahedron, 2011, 67, 10068 CrossRef;
(f) L. Wu, X. Zhang, Q.-Q. Chen and A.-K. Zhou, Org. Biomol. Chem., 2012, 10, 7859 RSC;
(g) J. Coetzee, G. R. Eastham, A. M. Z. Slawin and D. J. Cole-Hamilton, Org. Biomol. Chem., 2012, 10, 3677 RSC;
(h) H. Fuwa, K. Mizunuma, M. Sasaki, T. Suzuki and H. Kubo, Org. Biomol. Chem., 2013, 11, 3442 RSC.
- T. Murai, D. Nishi, S. Hayashi and W. Nakanishi, Bull. Chem. Soc. Jpn., 2014, 87, 677 CrossRef CAS.
- T. Murai, T. Hayashi, K. Yamada, Y. Maekawa and M. Minoura, Chem. Commun., 2014, 50, 12473 RSC.
- T. Murai, Y. Maekawa, M. Monzaki, T. Ando and T. Maruyama, Chem. Commun., 2013, 49, 9675 RSC.
- T. Murai, D. Matsuoka and K. Morishita, J. Am. Chem. Soc., 2006, 128, 4584 CrossRef CAS PubMed.
- M. J. Frischet al., Gaussian 09, revision D.01, Gaussian, Inc., Wallingford, CT, 2009 Search PubMed.
- T. Kimura, T. Murai and N. Mizuhata, Heteroat. Chem., 2005, 16, 185 CrossRef CAS.
- A. K. Guha, H. W. Lee and I. Lee, J. Org. Chem., 2000, 65, 12 CrossRef CAS PubMed.
- The oxygen isologues of the two diastereoisomers 7, which were prepared by the oxidation of 7, exhibited two signals for each diastereoisomer in their 31P NMR spectra, while those of 8 and 9 showed only one signal.
- For reviews, see:
(a) T. J. Wenzel, Discrimination of Chiral Compounds, John Wiley & Sons, Inc., New Jersey, 2007 Search PubMed;
(b) T. J. Wenzel and C. D. Chisholm, Prog. Nucl. Magn. Reson. Spectrosc., 2011, 59, 1 CrossRef CAS PubMed.
- For recent examples, see:
(a) M. Sakuma, A. Sakakura and K. Ishihara, Org. Lett., 2013, 15, 2383 CrossRef PubMed;
(b) A. Ficks, R. W. Harrington and L. J. Higham, Dalton Trans., 2013, 42, 6302 RSC;
(c) T. Amako, T. Kimoto, N. Tajima, M. Fujiki and Y. Imai, RSC Adv., 2013, 3, 6939 RSC;
(d) M. S. T. Morin and B. A. Arndtsen, Org. Lett., 2014, 16, 1056 CrossRef CAS PubMed;
(e) C. F. Czauderna, D. B. Cordes, A. M. Z. Slawin, C. Müller, J. I. van der Vlugt, D. Vogt and P. C. J. Kamer, Eur. J. Inorg. Chem., 2014, 1797 CrossRef CAS;
(f) E. L. Hazeland, A. M. Chapman, P. G. Pringle and H. A. Sparkes, Chem. Commun., 2015, 51, 10206 RSC.
- C. B. Caputo, S. J. Geier, E. Y. Ouyang, C. Kreitner and D. W. Stephan, Dalton Trans., 2012, 41, 237 RSC.
Footnote |
† Electronic supplementary information (ESI) available: Materials including experimental procedures, NMR spectra of all new products and X-ray diffraction data for Sax,S-7a (CCDC 1439654). For ESI and crystallographic data in CIF or other electronic formats see DOI: 10.1039/c6ra00318d |
|
This journal is © The Royal Society of Chemistry 2016 |
Click here to see how this site uses Cookies. View our privacy policy here.