DOI:
10.1039/C6RA01589A
(Paper)
RSC Adv., 2016,
6, 28034-28037
A highly selective and sensitive acylhydrazone-based turn-on optical sensor for Al3+†
Received
19th January 2016
, Accepted 10th March 2016
First published on 11th March 2016
Abstract
An acylhydrazone-based chemosensor M1 (2-hydroxy-((2-phenyl-2H-1,2,3-triazol-4-yl)methylene)benzohydrazide) was synthesized for the detection of Al3+. Unlike other receptors reported, M1 showed an excellent selectivity which could hardly be interfered by other ions like Cu2+ and Fe3+. The fluorescence intensity varied almost linearly vs. the concentration of Al3+, and the detection limit of Al3+ was estimated to be 1.2 × 10−8 M, illustrating that M1 can be used as a “turn-on” fluorescent chemosensor to selectively and sensitively detect Al3+ in ethanol.
1. Introduction
The development of selective and sensitive fluorescent sensors for the detection of metal ions has received more and more attention due to fundamental roles in medicine, living systems and the environment.1–9 Among those metals, aluminium is the third most prevalent element and the most abundant metal in the earth's crust.10–17 However, the unregulated amounts of aluminium in the human body may lead to the malfunction of central nervous system, Parkinson's disease and Alzheimer's disease.18–24 In 1989, the WHO listed aluminium ions as a source of food pollution and limited their concentration in drinking water to 200 mg L−1. For these reasons, the detection of aluminium ions is quite important.25–27
The majority of the reported Al3+ sensors have limitations such as requiring complicated synthesis and are insoluble in polar solutions.28,29 Besides, compared to transition metals, detection of Al3+ has always been problematic due to the lack of spectroscopic characteristics and poor coordination ability. As a result, many ions such as Fe3+, Cu2+, Co2+, Cd2+ may have a great deal of interference with those sensors.30–33 Therefore, design of high selective and sensitive chemosensors for Al3+ remains desirable.
Our research aims at development of new fluorescent chemosensor, which the compounds containing 2-phenyl-2H-1,2,3-triazole-4-yl structure units. Recently, we have reported this kinds of fluorescent chemosensors for mercury ion34 and cyanide anion.35 In the present manuscript, owing to the strong coordination power of the Schiff base (acylhydrazones) to metal ions and the possibility of a hydrogen bond between the amide (CONH)/OH groups and the targeted ions, a bi-function fluorescence chemosensor 2-phenyl-2H-1,2,3-triazole-4-yl based acylhydrazone compound M1 has been designed and synthesized. Fortunately, in the presence of Al3+, M1 displays a dramatic fluorescence enhancement in ethanol, while the interferences isn't been found in competition experiments. The detection limit was calculated to be 1.2 × 10−8 M, indicating that M1 is a highly sensitive and selective fluorescence probe for Al3+.
2. Experimental
2.1 Materials and instruments
All chemical reagents, unless otherwise specified, were purchased from Adamas and Acros Chemical Co. and used without further purification. Solutions of metal ions were prepared with metal nitrate salts. 1H NMR and 13C NMR spectra were recorded on a Varian 400 spectrometer. Absorption spectra were recorded using a Metash UV-6000PC UV-vis spectrometer. Emission spectra were recorded on a F-320 (Gangdong Technology) fluorescence spectrometer. All the measurements were taken at room temperature. Melting point was determined on a Beijing X-4 microscopic melting point apparatus.
2.2 Synthesis and characterization of M1
The synthetic route of M1 (2-hydroxy-((2-phenyl-2H-1,2,3-triazol-4-yl)methylene)benzohydrazide) was exhibited in Scheme 1. A mixture of 2-phenyl-2H-1,2,3-triazole-4-carbaldehyde (0.173 g, 1 mmol), 2-hydroxybenzohydrazide (0.164 g, 1.08 mmol), AcOH (2 mL) in ethanol was refluxed for 4 h. After the mixture was cooled to room temperature, the white precipitate was obtained, filtered-off and washed with ethanol. The product was then dried in vacuo. The yield: 92.5%. Mp = 275–277 °C; 1H NMR (400 MHz, DMSO-d6) δ 12.01 (s, 1H), 11.61 (s, 1H), 8.67 (s, 1H), 8.48 (s, 1H), 8.06 (d, J = 7.7 Hz, 2H), 7.85 (d, J = 6.9 Hz, 1H), 7.61 (t, J = 7.9 Hz, 2H), 7.47 (dd, J = 14.2, 6.8 Hz, 2H), 7.07–6.87 (m, 2H). 13C NMR (100 MHz, CF3COOD) δ 167.57, 159.74, 144.26, 140.59, 140.20, 139.91, 138.59, 133.27, 132.70, 132.15, 124.39, 122.56, 119.72, 114.36. IR (cm−1, KBr): 3424, 3240, 1632, 1543, 1490, 1308, 754. ESI-MS: m/z = 330.0952, calcd for C16H13N5O2 + Na+: 330.0967.
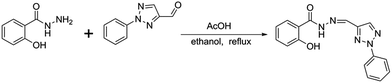 |
| Scheme 1 The synthetic procedure for M1. | |
3. Result and discussion
3.1 UV-vis experiments
The spectroscopic properties of the sensor M1 were investigated in ethanol. As shown in Fig. 1, the free chemosensor M1 alone exhibited a maximal absorption at 317 nm. Upon addition of Al3+ ions (10 equiv.), the absorbance at 317 nm decreased slightly while a new peak appears at 358 nm. According to the UV-vis absorption spectra of M1, we chose the peak (358 nm) as excitation wavelength in the following fluorescence emission measurements.
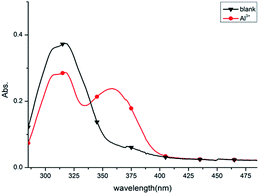 |
| Fig. 1 Changes in the absorption spectra of M1 (10.0 μM) in ethanol at room temperature as a function of added Al3+ (10.0 μM). | |
3.2 Fluorescence experiments
From the fluorescence spectra of M1 (Fig. 2), the free probe and other relevant metal ions did not show any significant fluorescence. In contrast, the addition of Al3+ resulted in a large enhancement of the emission intensity positioned around 442 nm (287 folds). Based on the use of a UV lamp, the solution of M1 showed a significant color change from dark blue to light blue in the presence of Al3+, which could easily be distinguished by the naked-eye (Fig. 3).
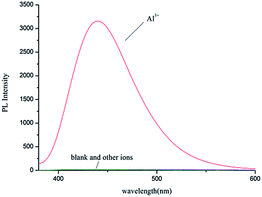 |
| Fig. 2 Fluorescence spectra of M1 (10.0 μM) and upon the addition of metal ions (10.0 equiv.) of Ag+, Ba2+, Ca2+, Cd2+, Co2+, Cr3+, Cu2+, Fe3+, Hg2+, Mg2+, Ni2+, Pb2+, Zn2+ and Al3+ in ethanol (λex = 358 nm). | |
 |
| Fig. 3 Photographs of the fluorescence of M1 (10.0 μM) in the presence of various metal ions (10.0 equiv.) under portable UV lamp. | |
To further investigate the practical applicability of M1 as a selective fluorescent sensor for Al3+, competitive metal ion selectivity experiments have been performed for the M1–Al3+ system in the presence of background metal ions. As exhibited in Fig. 4, M1 was treated with 10.0 equiv. of Al3+ in the presence of other metal ions of the same concentration. The results demonstrated that other competing metal ions, even Cu2+ and Fe3+, did not interfere with the binding of Al3+ with M1 and could not disrupt the highly fluorogenic detection of M1 for Al3+ in ethanol. Meanwhile, the influence of anions to detection of the Al3+ has also been observed. As shown in Fig. S6,† all of anions did not bring obvious change in the fluorescence emission spectra.
 |
| Fig. 4 Interference from other metal cations in a binary mixture: M1 (10.0 μM) + Al3+ (10.0 equiv.) + Mn+ (10.0 equiv.), where Mn+ = Ag+, Ba2+, Ca2+, Cd2+, Co2+, Cr3+, Cu2+, Fe3+, Hg2+, Mg2+, Ni2+, Pb2+, Zn2+ (λex = 358 nm, ethanol). | |
The quantitative nature of the sensing of Al3+ ion by M1 was elucidated by fluorescence titration of M1 (10 μM) in ethanol as described in Fig. 5. As the concentration of Al3+ increased, a gradual increase in the fluorescence intensity of chemosensor M1 at 442 nm was observed. From the fluorescence titration, the binding constant of M1 for Al3+ has been estimated. The slope of the line of best fit gives the Ka as 2.4 × 106 (Fig. 5b). Base on 3σ criteria, the LOD (limit of detection) of M1 for Al3+ was calculated to be 1.2 × 10−8 M.36
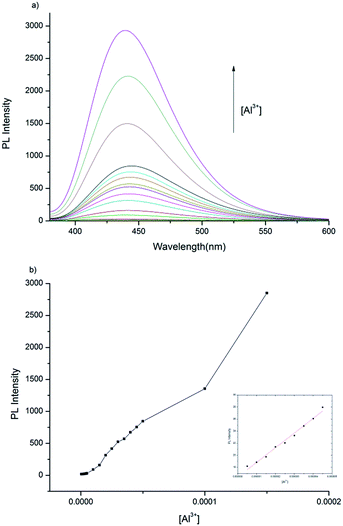 |
| Fig. 5 (a) Fluorescence spectra of M1 (10.0 μM) in ethanol upon the addition of Al(NO3)3 with an excitation of 358 nm. (b) Fluorescence intensity at 442 nm versus the number of equivalents of Al3+ added. Inset: enlarged figure in the concentration range of 5 × 10−7 to 5.00 × 10−6 M of Al3+. | |
Job's plot (Fig. S7†) using continuous variation with a total concentration of 10 μM M1 and Al3+ exhibited a maximum fluorescence (at 442 nm) when the molecular fraction of Al3+ and M1 was close to 1
:
1, suggesting a 1
:
1 stoichiometry for the binding of M1 and Al3+.
3.3 1H NMR titration experiments
In order to have better understanding of the complexation behavior of M1 with Al3+, 1H NMR spectra of M1 and M1–Al3+ complex were recorded in DMSO-d6. Fig. 6 illustrates that significant spectral changes were turned up with addition of 1 equiv. Al3+. Adding Al3+ to M1 caused the loss of the OH proton (11.61 ppm) with subsequent binding to electron deficient Al3+ which resulted in the disappearance of the OH peak.24,32 A new broad signal was developed at 8.67 ppm, which was assigned to CH
N of M1–Al3+. It is possibility that the interaction of the imine-nitrogen and Al3+ result in the peak of CH
N moving toward the downfield region. Furthermore, withdrawing electron density from the aromatic ring results the ortho-proton to the OH group moving toward the downfield (9.33 ppm). The ESI-MS spectra of the M1–Al3+ complex exhibits a molecular ion peak at m/z at 427.18 with a 100% intensity, which confirms the formula [AlNO3(M1)(CH3OH)] for the complex (Fig. S8†). Therefore, from Job's plot, 1H NMR titration experiments and the MS spectra of M1–Al3+ complex, the results suggest that M1 can chelate Al3+ through interactions with imine nitrogen and oxygen of phenolic hydroxyl group and forms 1
:
1 complex. Plausible mechanism of binding mode of M1 with Al3+ is shown in Scheme 2.
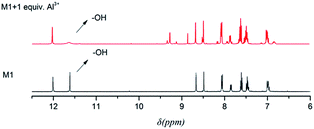 |
| Fig. 6 1H NMR spectra of M1 with Al(NO3)3·9H2O in DMSO-d6: M1 and M1 with 1 equiv. of Al3+. | |
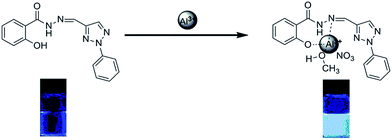 |
| Scheme 2 Plausible mechanisms for detection of Al3+ by M1. | |
4. Conclusion
In summary, an acylhydrazone-based chemosensor M1 was synthesized for fluorescent recognition of Al3+. The chemosensor was found to provide sensitive recognition of Al3+ through enhancement of fluorescence intensity at 442 nm. More importantly, because of its excellent selectivity and low detection limit, the chemosensor may find potential applications to detect nanomolar concentrations of Al3+ in the area of food safety and sewage treatment.
Acknowledgements
The authors are greatly appreciate the financial support from the National Natural Science Foundation of China (Project No. 21262034, 21364013).
References
- Y. Zhou, H. N. Kim and J. Yoon, Bioorg. Med. Chem. Lett., 2010, 20, 125 CrossRef CAS PubMed.
- W. Xu, D. Qi, J. You, F. Hu, J. Bian, C. Yang and J. Huang, J. Mol. Struct., 2015, 1091, 133 CrossRef CAS.
- T. Xu, H. Duan, X. Wang, X. Meng and J. Bu, Spectrochim. Acta, Part A, 2015, 138, 603 CrossRef CAS PubMed.
- H. Xu, X. Wang, C. Zhang, Y. Wu and Z. Liu, Inorg. Chem. Commun., 2013, 8 CrossRef CAS.
- L. Wang, D. Ye and D. Cao, Spectrochim. Acta, Part A, 2012, 90, 40 CrossRef PubMed.
- N. Vasimalai and S. A. John, J. Lumin., 2011, 131, 2636 CrossRef CAS.
- S. Suganya, S. Velmathi and D. Mubarak Ali, Dyes Pigm., 2014, 104, 116 CrossRef CAS.
- E. J. Song, G. J. Park, J. J. Lee, S. Lee, I. Noh, Y. Kim, S.-J. Kim, C. Kim and R. G. Harrison, Sens. Actuators, B, 2015, 213, 268 CrossRef CAS.
- P. Saluja, H. Sharma, N. Kaur, N. Singh and D. O. Jang, Tetrahedron, 2012, 68, 2289 CrossRef CAS.
- O. Alici and S. Erdemir, Sens. Actuators, B, 2015, 208, 159 CrossRef CAS.
- X. Cheng, R. Fang, Z. Yang, M. Wang, Q. Zhou, T. Li and Y. Li, J. Coord. Chem., 2014, 67, 737 CrossRef CAS.
- Y. W. Choi, G. J. Park, Y. J. Na, H. Y. Jo, S. A. Lee, G. R. You and C. Kim, Sens. Actuators, B, 2014, 194, 343 CrossRef CAS.
- S. Erdemir and S. Malkondu, J. Lumin., 2015, 158, 401 CrossRef CAS.
- S. Goswami, A. K. Das, K. Aich, A. Manna, H. K. Fun and C. K. Quah, Supramol. Chem., 2013, 26, 94 CrossRef.
- V. K. Gupta, A. K. Jain and G. Maheshwari, Talanta, 2007, 72, 1469 CrossRef CAS PubMed.
- S. A. Ingale and F. Seela, J. Org. Chem., 2012, 77, 9352 CrossRef CAS PubMed.
- S. Kim, J. Y. Noh, K. Y. Kim, J. H. Kim, H. K. Kang, S. W. Nam, S. H. Kim, S. Park, C. Kim and J. Kim, Inorg. Chem., 2012, 51, 3597 CrossRef CAS PubMed.
- C. Liu, Z. Yang and M. Yan, J. Coord. Chem., 2012, 65, 3845 CrossRef CAS.
- C. Lu, Y. Liu, P. Hung, C. Wan and A. Wu, Inorg. Chem. Commun., 2013, 273 CrossRef CAS.
- D. Maity and T. Govindaraju, Inorg. Chem., 2010, 49, 7229 CrossRef CAS PubMed.
- S. Malkondu, Tetrahedron, 2014, 70, 5580 CrossRef CAS.
- S. Malkondu and S. Erdemir, Tetrahedron, 2014, 70, 5494 CrossRef CAS.
- G. J. Park, Y. J. Na, H. Y. Jo, S. A. Lee, A. R. Kim, I. Noh and C. Kim, New J. Chem., 2014, 38, 2587 RSC.
- J. Qin, T. Li, B. Wang, Z. Yang and L. Fan, Synth. Met., 2014, 195, 141 CrossRef CAS.
- Y. Lu, Y. Liu, S. He, L. Zhao and X. Zeng, Org. Lett., 2011, 13(19), 5274 CrossRef CAS PubMed.
- K. Zhang, Z. Y. Yang, B. D. Wang, S. B. Sun, Y. D. Li, T. R. Li, Z. C. Liu and J. M. An, Spectrochim. Acta, Part A, 2014, 124, 59 CrossRef CAS PubMed.
- X. Zhou, P. Li, Z. Shi, X. Tang, C. Chen and W. Liu, Inorg. Chem., 2012, 51, 9226 CrossRef CAS PubMed.
- C. Li, J. Qin, G. Wang, B. Wang, A. Fu and Z. Yang, Inorg. Chim. Acta, 2015, 430, 91 CrossRef CAS.
- R. Azadbakht and J. Khanabadi, Inorg. Chem. Commun., 2013, 30, 21 CrossRef CAS.
- X. Jiang, B. Wang, Z. Yang, Y. Liu, T. Li and Z. Liu, Inorg. Chem. Commun., 2011, 14, 1224 CrossRef CAS.
- Y. J. Lee, C. Lim, H. Suh, E. J. Song and C. Kim, Sens. Actuators, B, 2014, 201, 535 CrossRef CAS.
- K. Tiwari, M. Mishra and V. P. Singh, RSC Adv., 2013, 3, 12124 RSC.
- X. Sun, Y. Wang and Y. Peng, Org. Lett., 2012, 14(13), 3420 CrossRef CAS PubMed.
- H. Lin, W. Shi, Y. Tian, F. Ma, L. Xu, J. Ma, Y. Hui and Z. Xie, J. Lumin., 2015, 157, 280 CrossRef CAS.
- Y. Chen, W. Shi, Y. Hui, X. Sun, L. Xu, L. Feng and Z. Xie, Talanta, 2015, 137, 38 CrossRef CAS PubMed.
- T. Volker, S. Debbie and M. David, Spectroscopy, 2003, 18(12), 112 Search PubMed.
Footnote |
† Electronic supplementary information (ESI) available: 1H & 13C NMR, IR and mass spectra of M1; UV-visible spectral changes of M1 with various ions; Job's plot of M1 and Al3+. See DOI: 10.1039/c6ra01589a |
|
This journal is © The Royal Society of Chemistry 2016 |
Click here to see how this site uses Cookies. View our privacy policy here.