DOI:
10.1039/C6RA03367A
(Communication)
RSC Adv., 2016,
6, 25620-25623
A novel red to transmissive electrochromic polymer based on phenanthrocarbazole†
Received
5th February 2016
, Accepted 1st March 2016
First published on 2nd March 2016
Abstract
A novel red to transmissive electrochromic polymer is described. A monomer which consists of EDOT and phenanthrocarbazole units was designed. Upon electrochemical polymerization of this material, a red colored polymer film was formed, which upon oxidation switches to a highly transmissive state. A high optical contrast (51%) and fast switching times (0.65 s and 0.52 s) were achieved, making this polymer an important candidate for electrochromic device and simple display applications. In addition, to best of our knowledge, this is the first example of a phenanthrocarbazole containing electrochromic polymer.
π-Conjugated organic polymers are now an important class of semiconductors and their electronic and optoelectronic properties enabled their use in various types applications including field-effect transistors,1,2 OLEDs,3 organic photovoltaics,4 sensors5 and electrochromic devices.6 Electrochromism is widely defined as the reversible color change upon applied potential, and various materials that showed electrochromic behavior have been synthesized and characterized over the past few decades.6–12 There is a plethora of applications that utilizes electrochromic materials, such as smart windows, simple color displays and camouflage materials.13,14 One of the major breakthroughs in the area of electrochromic material research was the completion of RGB additive color space with the discovery of green to transmissive polymeric materials.15–20 Another significant achievement in the field was the discovery of the yellow to transmissive polymers, which was the major obstacle towards the completion of subtractive color space (CMYK).21 Even though these major goals in the electrochromic research area were fulfilled over the past decade, towards high quality display applications, electrochromic materials with better optical contrasts and faster switching times are needed. There are a few reports on the red to transmissive polymers that were recently published literature.22–24 Even though high optical contrast values were reported, these materials were produced by oxidative polymerization utilizing either two or three monomers, which might constitute a reproducibility issue. Additionally the switching time of the materials were in the order of couple seconds, which hinders their use in certain device applications. This paper describes our initial findings towards realization of a well-defined, novel, high optical contrast and fast switching red to transmissive polymer. Our design principle was based on two significant observations based on previous reports on electrochromic materials. (1) Donor–acceptor type polymers tend to result in two absorption bands and in fact, this approach was used for the discovery of the green to transmissive electrochromic polymer.20 For red to transmissive materials, a single absorption band in the visible region is needed. (2) Research in the field clearly demonstrated that most of the EDOT containing conjugated polymers shows superior electrochromic characteristics. Hence, it was envisioned that if EDOT was combined with a highly conjugated “donor” material, the corresponding polymer should yield a single absorption band in the visible region that is red-shifted compared to PEDOT. Phenanthrocarbazole was chosen as the donor unit. Materials utilizing this structure have been successfully used in solar cell25 and OFET applications26 but this is the first example of an electrochromic polymer that contains phenanthrocarbazole. Electrochemical polymerization was determined as the method for the synthesis of the polymer. This method has the advantage of having the polymer film directly on the conductive surface and is better suited for screening of potential high performance electrochromic materials.
The structure of the monomer (DEP) and the synthetic pathway is shown below (Fig. 1). The synthesis started with alkylation27 of N-heterocyclic perylene derivative 1.28,29 The alkylated derivative 2 was then brominated with NBS to get compounds 3. Stannylated EDOT (4)30 and 3 was coupled in the presence of a Pd catalyst and the monomer DEP was synthesized successfully. All new materials were fully characterized with 1H-NMR, 13C-NMR and HRMS.
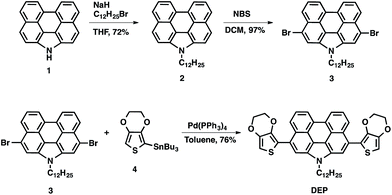 |
| Fig. 1 The synthetic pathway for the monomer DEP. | |
Next, the potentiodynamic polymerization of DEP was performed from a 10 mM solution of DEP in 0.1 M TBAPF6/MeCN supporting electrolyte–solvent couple (Fig. 2). An oxidation peak was observed at 0.69 V which was attributed to non-polymerizable radical cation formation most probably on the perylene unit. The monomer oxidation centered on EDOT unit was observed at 1.07 V and formation of a highly electroactive polymer was observed in the following cycles. The polymer oxidation and reduction potentials (10th cycle) were observed as 0.68 V and 0.35 V respectively. FT-IR, SEM and AFM analyses of the polymer are given in ESI.† Polymer film that were grown potentiodynamically on ITO was then subjected to spectroelectrochemistry studies. The UV-vis-NIR spectra were recorded as a function of applied potential (−0.3 V to 1.1 V with 0.1 V increments). The results are shown below (Fig. 3).
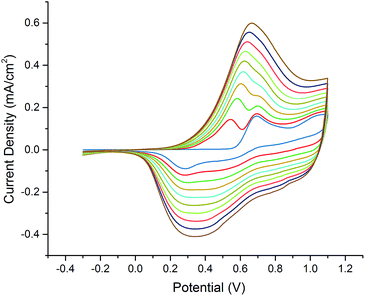 |
| Fig. 2 Repeated scan polymerization of DEP (WE: ITO, CE: Pt wire, RE: Ag wire, 0.1 M TBAPF6/MeCN, 100 mV s−1, 10 cycles). | |
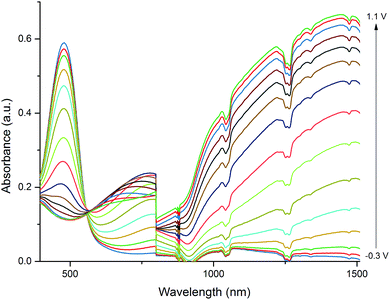 |
| Fig. 3 Spectroelectrochemistry studies of PDEP (0.3 V to 1.1 V with 0.1 V increments). | |
In its neutral state the PDEP show a narrow absorption band centered at 480 nm. Upon applied potential the strong absorption gradually depletes and a transmissive state is achieved. The polaron band (centered at 770 nm) increases upon applied positive potential. As the potential keeps increasing however, depletion of this band was observed. This is a crucial phenomenon resulted in higher transparency in the oxidized state (Fig. 4).
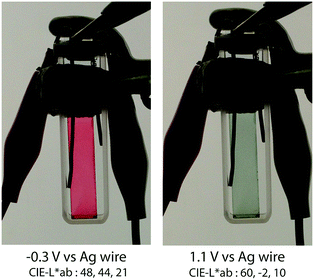 |
| Fig. 4 Photographs of the PDEP at its neutral and oxidized states. | |
Next, the kinetic studies for the PDEP were performed in order to probe changes in transmittance with time, while the polymer was repeatedly stepped between the neutral and oxidized states. The polymer film was deposited on ITO glass slide by repeated scanning (15 cycles) in TBAPF6/MeCN. The film thickness was determined to be 147 nm from AFM measurements (ESI†). The results can be seen from Fig. 5. The optical contrast of the PDEP was found to be 51% at 480 nm (λmax) with a fast switching time of 0.65 seconds for oxidation and 0.52 seconds for reduction (Fig. 6). The optical contrast is comparable with the other red to transmissive polymers (60%, 57%, 56%) in the literature, however the switching time was found to be considerably faster (0.65 s versus, 3.6 s, 2.3 s, 1.6 s).22–24 The coloration efficiency of the material was calculated to be 172 cm2 C−1, a comparable value to the high performance electrochromic material PEDOT (183 cm2 C−1).31
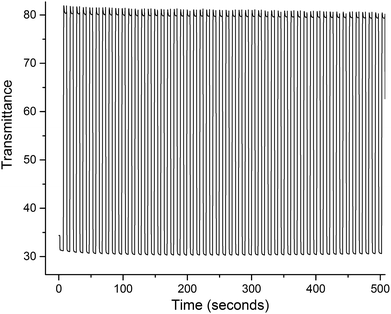 |
| Fig. 5 Electrochromic switching and optical absorbance change monitored at 480 nm (50 cycles). | |
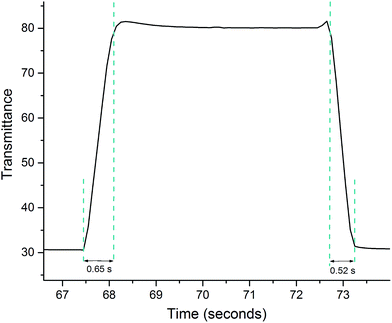 |
| Fig. 6 Electrochromic switching time and optical absorbance change monitored at 480 nm (1 cycle). | |
The stability of the PDEP film was also investigated. The polymer film was coated (TBAPF6/MeCN) on Pt electrode (0.12 cm2) was cycled 200 times (TBAPF6/PC) with 100 mV s−1 scan rate (Fig. 7). The polymer retained 92% of its activity after 200 cycles showing that PDEP has good stability.
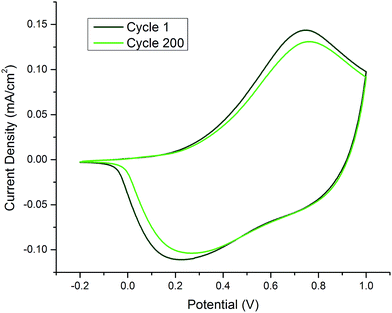 |
| Fig. 7 Cyclic voltammetry stability test of PDEP (WE: Pt, RE: Ag wire, 0.1 M TBAPF6/PC, 100 mV s−1). | |
Conclusions
A novel EDOT and phenanthrocarbazole containing polymer was synthesized electrochemically and electrochromic properties were investigated. To best of our knowledge, this is the first example of a phenanthrocarbazole-containing electrochromic material. The polymer showed red color in its neutral state and becomes highly transmissive upon oxidation with a high optical contrast of 51%. The polymer revealed very fast switching times (the fastest among red-transmissive polymers) of 0.65 s (oxidation) and 0.52 s (reduction). This study shows that PDEP is an important candidate for simple electrochromic display applications. In addition, results revealed that phenanthrocarbazole is a promising unit towards realization of novel, high-performance electrochromic materials. Synthesis of a solution processable derivative of PDEP and other novel phenanthrocarbazole containing polymers are currently pursued in our group.
Experimental
1-Dodecyl-1H-phenanthro[1,10,9,8-c,d,e,f,g]carbazole (2)
To a mixture of compound 1 (300 mg, 1.13 mmol) in dry THF (30 mL), was added NaH (40.8 mg, 1.70 mmol) and 1-bromododecane (425 mg, 1.71 mmol) respectively. The mixture was refluxed at 65 °C for 23 hours under argon atmosphere. After gradually cooling to 0 °C, the mixture was stirred for 30 min. Water was carefully (2 mL) added drop wise to quench any remaining NaH, and the mixture was then poured into water. The mixture was then extracted with diethyl ether (3 × 100 mL). The combined organic layers were washed with brine, dried (Na2SO4), and concentrated in vacuo. The crude product was purified by column chromatography on silica gel (6
:
1 petroleum ether/DCM) to yield a yellow wax (353 mg, 72%). 1H-NMR: (400 MHz, CDCl3) δ 8.65 (d, J = 7.5 Hz, 2H), 8.12 (d, J = 8.0 Hz, 2H), 7.91 (d, J = 8.8 Hz, 2H), 7.84–7.76 (m, 4H), 4.67 (t, J = 6.9 Hz, 2H), 2.08 (quin., J = 7.1 Hz, 2H), 1.46–1.11 (m, 18H), 0.88 (t, J = 6.9 Hz, 3H); 13C-NMR: (400 MHz, CDCl3) δ 132.0, 130.48, 128.88, 125.08, 125.00, 124.58, 123.70, 120.79, 117.53, 113.46, 45.94, 32.04, 31.34, 29.71, 29.67, 29.59, 29.45, 29.42, 27.29, 22.82, 14.26.
3,10-Dibromo-1-dodecyl-1H-phenanthro[1,10,9,8-c,d,e,f,g]carbazole (3)
To a solution of compound 2 (351 mg, 0.810 mmol) in dry DCM (21 mL) was added NBS (297 mg, 1.67 mmol) portion wise and the mixture was stirred at room temperature for 30 min under argon atmosphere. The solvent was evaporated under reduced pressure, and the resulting residue was purified by column chromatography on silica gel (3
:
1 petroleum ether/DCM) to yield a yellow solid (465 mg, 97%): 1H-NMR (400 MHz, CDCl3) δ 8.24 (d, J = 7.6 Hz, 2H), 8.09 (d, J = 8.2 Hz, 2H), 7.67 (t, J = 7.8 Hz, 2H), 7.65 (s, 2H), 4.12 (t, J = 7.1 Hz, 2H), 1.81 (quin., J = 7.1 Hz, 2H), 1.42–1.05 (m, 18H), 0.86 (t, J = 6.9 Hz, 3H); 13C-NMR (400 MHz, CDCl3) δ 130.93, 129.33, 127.41, 125.21, 124.75, 123.43, 121.44, 117.84, 116.58, 115.69, 45.77, 32.03, 31.10, 29.70, 29.65, 29.56, 29.44, 29.29, 27.15, 22.81, 14.26. HRMS calculated for C32H33Br2N: 591.0959. Found: 591.0961.
3,10-Bis(2,3-dihydrothieno[3,4-b][1,4]dioxin-5-yl)-1-dodecyl-1H-phenanthro[1,10,9,8-c,d,e,f,g]carbazole (DEP)
Compound 3 (100 mg, 0.170 mmol), and compound 4 (313 mg, 0.730 mmol) were dissolved in dry toluene (30 mL) and the mixture was purged with argon for 30 min. Then, tetrakis (triphenylphosphine)palladium(0) (35 mg, 0.030 mmol) was added at room temperature under argon atmosphere. The mixture was heated to 115 °C and stirred in the dark for 24 hours. The solvent was evaporated under reduced pressure, and the crude oily residue was purified by column chromatography on silica gel (1
:
1 petroleum ether/DCM) to yield a yellow-orange solid (91 mg, 76%): 1H-NMR (400 MHz, CDCl3) δ 8.71 (d, J = 7.5 Hz, 2H), 8.24 (d, J = 8.2 Hz, 2H), 7.91 (s, 2H), 7.85 (t, J = 7.9 Hz, 2H), 6.54 (s, 2H), 4.71 (t, J = 7.0 Hz, 2H), 4.46–4.19 (m, 8H), 2.11 (quin., J = 7.1 Hz 2H), 1.49–1.13 (m, 18H), 0.85 (t, J = 6.9 Hz, 3H); 13C-NMR (400 MHz, CDCl3) δ 141.78, 138.19, 132.04, 130.65, 127.77, 126.75, 124.79, 124.76, 124.69, 121.22, 117.61, 117.19, 116.07, 98.80, 64.95, 64.85, 45.94, 32.03, 31.33, 29.73, 29.70, 29.64, 29.45, 29.42, 27.30, 22.81, 14.27. HRMS calculated for C44H43NO4S2: 713.2643. Found: 713.2634.
Notes and references
- C. L. Wang, H. L. Dong, W. P. Hu, Y. Q. Liu and D. B. Zhu, Chem. Rev., 2012, 112, 2208 CrossRef CAS PubMed.
- G. Kim, S. J. Kang, G. K. Dutta, Y. K. Han, T. J. Shin, Y. Y. Noh and C. Yang, J. Am. Chem. Soc., 2014, 136, 9477 CrossRef CAS PubMed.
- M. S. AlSalhi, J. Alam, L. A. Dass and M. Raja, Int. J. Mol. Sci., 2011, 12, 2036 CrossRef CAS PubMed.
- L. Y. Lu, T. Y. Zheng, Q. H. Wu, A. M. Schneider, D. L. Zhao and L. P. Yu, Chem. Rev., 2015, 115, 12666 CrossRef CAS PubMed.
- D. T. McQuade, A. E. Pullen and T. M. Swager, Chem. Rev., 2000, 100, 2537 CrossRef CAS PubMed.
- G. Gunbas and L. Toppare, Chem. Commun., 2012, 48, 1083 RSC.
- S. H. Hsiao and Y. T. Chiu, RSC Adv., 2015, 5, 90941 RSC.
- Z. G. Shi, W. T. Neo, T. T. Lin, H. Zhou and J. W. Xu, RSC Adv., 2015, 5, 96328 RSC.
- T. Soganci, M. Ak, E. Giziroglu and H. C. Soyleyici, RSC Adv., 2016, 6, 2810 RSC.
- S. L. Ming, S. J. Zhen, K. W. Lin, L. Zhao, J. K. Xu and B. Y. Lu, ACS Appl. Mater. Interfaces, 2015, 7, 11089 CAS.
- C. M. Cho, Q. Ye, W. T. Neo, T. T. Lin, X. H. Lu and J. W. Xu, Polym. Chem., 2015, 6, 7570 RSC.
- C. M. Cho, Q. Ye, W. T. Neo, T. T. Lin, J. Song, X. H. Lu and J. W. Xu, J. Mater. Sci., 2015, 50, 5856 CrossRef CAS.
- R. J. Mortimer, Electrochromic materials and devices, Wiley-VCH, Weinheim, 2015 Search PubMed.
- P. R. Somani and S. Radhakrishnan, Mater. Chem. Phys., 2003, 77, 117 CrossRef CAS.
- A. Durmus, G. E. Gunbas, P. Camurlu and L. Toppare, Chem. Commun., 2007, 31, 3246 RSC.
- A. Durmus, G. E. Gunbas and L. Toppare, Chem. Mater., 2007, 19, 6247 CrossRef CAS.
- P. M. Beaujuge, S. Ellinger and J. R. Reynolds, Adv. Mater., 2008, 20, 2772 CrossRef CAS PubMed.
- A. Cihaner and F. Algi, Adv. Funct. Mater., 2008, 18, 3583 CrossRef CAS.
- G. E. Gunbas, A. Durmus and L. Toppare, Adv. Funct. Mater., 2008, 18, 2026 CrossRef CAS.
- G. E. Gunbas, A. Durmus and L. Toppare, Adv. Mater., 2008, 20, 691 CrossRef CAS.
- C. M. Amb, J. A. Kerszulis, E. J. Thompson, A. L. Dyer and J. R. Reynolds, Polym. Chem., 2011, 2, 812 RSC.
- A. L. Dyer, M. R. Craig, J. E. Babiarz, K. Kiyak and J. R. Reynolds, Macromolecules, 2010, 43, 4460 CrossRef CAS.
- X. M. Chen, Z. P. Xu, S. Mi, J. M. Zheng and C. Y. Xu, New J. Chem., 2015, 39, 5389 RSC.
- Z. P. Xu, X. M. Chen, S. Mi, J. M. Zheng and C. Y. Xu, Org. Electron., 2015, 26, 129 CrossRef CAS.
- Z. Q. Deng, L. Chen and Y. W. Chen, J. Polym. Sci., Part A: Polym. Chem., 2013, 51, 4885 CrossRef CAS.
- H. J. Chen, Y. L. Guo, X. N. Sun, D. Gao, Y. Q. Liu and G. Yu, J. Polym. Sci., Part A: Polym. Chem., 2013, 51, 2208 CrossRef CAS.
- Y. Li, L. X. Hao, H. B. Fu, W. Pisula, X. L. Feng and Z. H. Wang, Chem. Commun., 2011, 47, 10088 RSC.
- J. J. Looker, J. Org. Chem., 1972, 37, 3379 CrossRef CAS.
- W. Jiang, H. L. Qian, Y. Li and Z. Wang, J. Org. Chem., 2008, 73, 7369 CrossRef CAS PubMed.
- L. Chen, B. H. Zhang, Y. X. Cheng, Z. Y. Xie, L. X. Wang, X. B. Jing and F. S. Wang, Adv. Funct. Mater., 2010, 20, 3143 CrossRef CAS.
- C. L. Gaupp, D. M. Welsh, R. D. Rauh and J. R. Reynolds, Chem. Mater., 2002, 14, 3964 CrossRef CAS.
Footnote |
† Electronic supplementary information (ESI) available: FT-IR, SEM and AFM data of the polymer. See DOI: 10.1039/c6ra03367a |
|
This journal is © The Royal Society of Chemistry 2016 |
Click here to see how this site uses Cookies. View our privacy policy here.