DOI:
10.1039/C6RA03378D
(Paper)
RSC Adv., 2016,
6, 35764-35770
A concise approach to indoles via oxidative C–H amination of 2-alkenylanilines using dioxygen as the sole oxidant†
Received
5th February 2016
, Accepted 31st March 2016
First published on 1st April 2016
Abstract
A novel synthetic method to prepare indole derivatives from N-Ts-2-alkenylanilines has been achieved. This reaction is through an oxidative intramolecular C–H amination by using molecular oxygen as the sole oxidant. This protocol is operationally simple and environmental friendly, and provides a diverse range of substrate scope.
Introduction
Indoles are important and valuable heterocycles because of their wide application in chemistry, biology, and material sciences.1 Therefore the synthesis of indole derivatives has long been of great interest in organic synthesis.2 A great number of superior methods have been established, and a large portion of these methods employ transition metal catalysts.3 These protocols are efficient and have been widely applied in organic synthesis. However, to be more friendly to the environment and economic, developing a metal-free methodology to prepare indole derivatives is an attractive goal.4
The intramolecular amination reaction of alkenes is a direct approach to indole synthesis and these approaches are known in the literature.5 The different oxidants were utilized for the metal-free version (Scheme 1), for example, Muñiz reported a sulfonic acid-catalyzed synthesis of indoles using iodosobenzene as the oxidant.4a Later on, Youn reported a synthesis of indoles through oxidative C–H amination of 2-alkenylanilines by 2,3-dichloro-5,6-dicyano-1,4-benzoquinone (DDQ) at 120 °C.4b Recently, Ortgies and Zhao successively demonstrated the protocols involving the use N-fluorobenzenesulfonimide as the terminal oxidant catalyzed by selenium via the intramolecular amination of C(sp2)–H bonds.4c,d Our laboratory is engaged in developing transition-metal-free methods for the synthesis of heterocycles, especially through new C–N, C–O bond formation.6 Although we have achieved the synthesis of indoles by N-iodosuccinimide (NIS) mediated cascade C–N bond formation/aromatization of 2-alkenylanilines under temperate conditions,6a we became interested in the design of the novel method to replace these oxidants by more environmentally friendly and economical reagent. Since dioxygen is very cheap and produces no environmentally hazardous byproduct, and has been extensively applied in organic synthesis.7 Consequently we wish to communicate here our success in achieving the practical and novel synthesis of indole derivatives using molecular oxygen as the terminal oxidant.
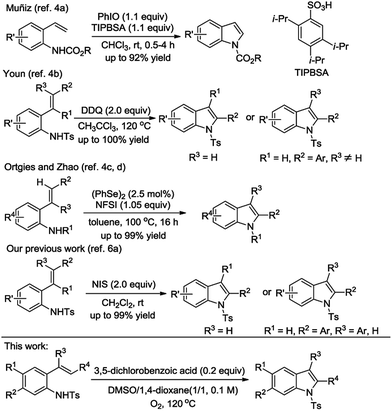 |
| Scheme 1 Metal-free indole synthesis from 2-alkenylanilines. | |
Results and discussion
We began our investigation with the anilide 1a as the model substrate to search for the optimal reaction conditions (Table 1). First, we evaluated the solvent effect on this reaction. Various solvents were tested, only dimethyl sulfoxide (DMSO) and 1,4-dioxane showed relatively high yield (Table 1, entries 1, 2).
Table 1 Optimization of reaction conditionsa
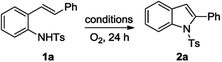
|
Entry |
Reagent (0.2 equiv.) |
Solvent |
Yieldb (%) |
Reaction conditions: 1a (0.1 mmol), additive (0.2 equiv.), solvent (1.0 mL), 120 °C under O2 (balloon). Isolated yield, ND = not detected. DMSO/dioxane (3 : 7). DMSO/1,4-dioxane (7 : 3). Using 1.0 equiv. additive. At 100 °C. At 150 °C. 0.05 M. 0.15 M. |
1 |
— |
1,4-Dioxane |
30 |
2 |
— |
DMSO |
22 |
3 |
— |
Toluene |
<10 |
4 |
— |
THF |
0 |
5 |
— |
ClCH2CH2Cl |
<10 |
6 |
— |
CCl4 |
<10 |
7 |
— |
HOCH2CH2OH |
15 |
8 |
— |
EtOH |
<10 |
9 |
— |
DMSO/1,4-dioxane |
32 |
10 |
K2CO3 |
DMSO/1,4-dioxane |
0 |
11 |
t-BuONa |
DMSO/1,4-dioxane |
0 |
12 |
CH3COOH |
DMSO/1,4-dioxane |
44 |
13 |
TsOH |
DMSO/1,4-dioxane |
50 |
14 |
Benzoic acid |
DMSO/1,4-dioxane |
58 |
15 |
3-Chlorobenzoic acid |
DMSO/1,4-dioxane |
61 |
16 |
3,5-Dichlorobenzoic acid |
DMSO/1,4-dioxane |
73 |
17 |
3,5-Difluorobenzoic acid |
DMSO/1,4-dioxane |
58 |
18c |
3,5-Dichlorobenzoic acid |
DMSO/1,4-dioxane |
61 |
19d |
3,5-Dichlorobenzoic acid |
DMSO/1,4-dioxane |
37 |
20e |
3,5-Dichlorobenzoic acid |
DMSO/1,4-dioxane |
46 |
21f |
3,5-Dichlorobenzoic acid |
DMSO/1,4-dioxane |
ND |
22g |
3,5-Dichlorobenzoic acid |
DMSO/1,4-dioxane |
20 |
23h |
3,5-Dichlorobenzoic acid |
DMSO/1,4-dioxane |
47 |
24i |
3,5-Dichlorobenzoic acid |
DMSO/1,4-dioxane |
29 |
Combination of these two solvents and gratifyingly provided a higher yield (Table 1, entry 9). Next, we then turned our attention to screen the additives. When the benzoic acid as additive for this reaction, the corresponding indole was obtained in good yield (Table 1, entry 14), however, poorer yields were obtained when we use acetic acid and p-toluenesulfonic acid (Table 1, entries 12, 13). Base such as potassium carbonate and sodium tert-butoxide proved to be ineffective for this transformation and resulted in no reaction (Table 1, entries 10, 11). Subsequently, we examined benzoic acid derivatives and delightfully find that the 3,5-dichlorobenzoic acid appeared preferable with regard to product yield (Table 1, entry 16). We attempted to increase the amount of 3,5-dichlorobenzoic acid to 1 equiv. and it led to decrease the yield to 46% (Table 1, entry 20). We changed the ratio of DMSO and 1,4-dioxane, and the yield is decreasing (Table 1, entries 18, 19). Alteration of key operating parameters was also examined, e.g., neither lower nor raise the temperature could increase the yield (Table 1, entries 21, 22). Last but not the least, we examined the concentration of this reaction and it turned out the 0.1 M is appropriate (Table 1, entries 23, 24). After all above experiments, the optimal conditions for the synthesis of indole derivatives using molecular oxygen as the sole oxidant were established [0.2 equiv. 3,5-dichlorobenzoic acid, DMSO/1,4-dioxane (1/1, 0.1 M), O2, 120 °C].
With optimized conditions in hand, a brief survey of the effect of N-protecting groups including sulfonyl (entries 1–6), acyl (entry 7) reconfirmed the effectiveness of p-toluenesulfonyl as the preferred group for this reaction (Table 2). These observations proved that the pKa of the N–H units plays a significant role in the reaction.
Table 2 Effects of protecting groupsa
To probe the flexibility of this methodology, we proceeded to explore the substituent effect at the alkene moiety (Table 3, entries 1–14). We tested the substrates with various ortho-, meta- and para-substitution on the phenyl ring at the alkene moiety. The results reveal that there is a certain influence on the substitution pattern of the substituent on the phenyl ring of the substrates. The electron-donating groups at different positions furnished the corresponding products in moderate to good yields. For example, substrates with a methyl or methoxyl group at ortho-, meta- and para-substitution on the phenyl ring provided the desired products with 45–72% yields (Table 3, entries 2–7). Both tert-butylbenzene alkene and cyclopropyl alkene only can obtain about 50% yields (Table 3, entries 8, 9). The alkene without any substitutions is also suitable under the reaction conditions (Table 3, entry 11). It is noted that the naphthyl alkene formed the desired product in best yield (76% yield, Table 3, entry 10). While the electron-withdrawing groups at different positions obtained the corresponding products in lower yields. Such as substrates with F, Cl substituents on the phenyl ring provided the desired products in 44% and 36% yields respectively (Table 3, entries 12, 13). When the substrates with NO2 on the phenyl ring, the target products cannot be formed (Table 3, entry 14). This result was probably caused by the strong electron-withdrawing characteristic of the nitro group.
Table 3 Scope of 2-alkenylanilinesa
Subsequently we also investigated the effect of the substitution pattern of aniline. As shown in Table 3 (entries 15–23), the reaction exhibited poor tolerance to various substituents on the aromatic ring. Substituents (R1) residing on the aromatic moiety of N-Ts-2-styrylanilines showed fair yields. When it is electron-donating group, the reaction took place smoothly to provide the desired indoles in better yields (Table 3, entries 15, 16). When it is electron-withdrawing group such as F, Cl and CF3, the lower yields were obtained (Table 3, entries 17–19). The substituents (R2) residing on the aromatic moiety of N-Ts-2-styrylanilines showed relatively lower yields (Table 3, entries 20, 21). Apparently, this reaction is affected by the position of the substituents on the aromatic ring of anilines. We then tested substrates with two substituents on the aromatic ring of the alkene and aniline. When both of them are electron-donating group, the reaction took place smoothly to provide a higher yield than electron-withdrawing group was involved (Table 3, entries 22, 23).
After successfully synthesized a series of indole derivatives by oxidative C–H amination of 2-alkenylanilines. To gain more insight about the mechanism of above reaction, several control experiments were conducted (Scheme 2). First, TEMPO as a radical scavenger was added into the reaction with optimized conditions. However, there is no desired product was obtained only recovered the starting material. The fact might suggest a radical initiation pathway. Furthermore, this oxidation was completely inhibited under nitrogen. A control study using hydrogen peroxide as the oxidant did not lead to the desired indole. This result indicates that the reaction is not mediated by hydrogen peroxide.
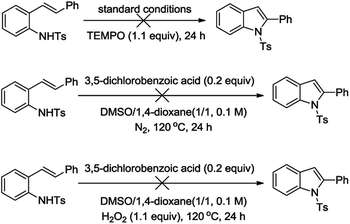 |
| Scheme 2 Control experiments. | |
Although the reaction mechanism has not been fully established at the present stage,8,9 a plausible mechanism for this reaction was outlined in Scheme 3. Under thermal condition, anilide was oxidized into nitrogen radical cation by singlet oxygen, then electrophilic addition of this radical cation to alkene to generate benzylic radical, which was oxidated to its corresponding benzylic cation. During this process, 3,5-dichlorobenzoate anion was formed and it can assist the following aromatization to provide the product.
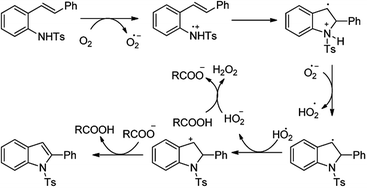 |
| Scheme 3 A proposed mechanistic pathway. | |
Conclusions
In conclusion, we have demonstrated a synthetic method to prepare the indole derivatives via an oxidative intramolecular C–H amination of 2-alkenylanilines by using molecular oxygen as sole oxidant. In comparison to other oxidants mediated reactions, this method was an environmentally friendly process. Furthermore, our discovery provides a possibility to design more new reactions in the field of aerobic oxidation of alkene.
Experimental
General information
All reactions were performed in standard glassware. Solvents were distilled prior to use. All commercially available reagents were used as purchased without further purification. 1H and 13C NMR spectra were obtained on 600 MHz (150 MHz for 13C NMR) spectrometer at 25 °C, using CDCl3 with TMS or residual solvent as standard unless otherwise noted. Chemical-shift values were given in ppm and referenced to the internal standard TMS (tetramethylsilane). The peak patterns are indicated as follows: s, singlet; d, doublet; t, triplet; q, quadruplet; m, multiplet; dd, doublet of doublets, and br s, broad singlet. The coupling constants (J) are reported in hertz (Hz). Melting points were determined with a micromelting point apparatus without corrections. High-resolution mass spectrometry (HRMS) was obtained on a Q-TOF microspectrometer. Flash column chromatography was performed over silica gel 200–300 mesh.
General procedure for the indole synthesis
To a solution of substrate (0.1 mmol) in DMSO/1,4-dixoane (1
:
1, 1.0 mL) was added 3,5-dichlorobenzoic acid (4 mg, 0.02 mmol) under oxygen (balloon). The resulting mixture was stirred at 120 °C for the reported time, and then the mixture was concentrated under reduced pressure. The residue was purified by column chromatography on silica gel to give the corresponding product.
N-Ts-2-phenylindole (2a)4b. White solid, mp: 145–148 °C (Lit. 142–144 °C); Rf = 0.59 (10% EtOAc/petroleum ether); 1H NMR (600 MHz, CDCl3) δ 2.27 (s, 3H), 6.54 (s, 1H), 7.03 (d, J = 7.8 Hz, 2H), 7.27 (d, J = 7.7 Hz, 3H), 7.35 (t, J = 7.7 Hz, 1H), 7.43–7.44 (m, 4H), 7.49–7.50 (m, 2H), 8.31 (d, J = 8.3 Hz, 1H). 13C NMR (150 MHz, CDCl3) δ 21.5, 113.6, 116.6, 120.7, 124.3, 124.8, 126.8, 127.5, 128.6, 129.2, 130.3, 130.5, 132.4, 134.6, 138.2, 142.1, 144.5; HRMS (ESI): m/z calcd for C21H17NO2S (M + Na)+ 370.0878, found 370.0891.
N-(Benzenesulfonyl)-2-phenylindole (2b)4b. White solid, mp: 91–93 °C (Lit. 98–101 °C); Rf = 0.42 (10% EtOAc/petroleum ether); 1H NMR (600 MHz, CDCl3) δ 6.53 (s, 1H), 7.23 (t, J = 7.8 Hz, 2H), 7.27 (d, J = 7.2 Hz, 1H), 7.34–7.49 (m, 10H), 8.32 (d, J = 8.4 Hz, 1H). 13C NMR (150 MHz, CDCl3) δ 112.7, 115.6, 119.7, 123.4, 123.8, 125.7, 126.5, 127.5, 127.7, 129.3, 129.5, 131.3, 132.5, 137.5, 139.2, 142.1; HRMS (ESI): m/z calcd for C20H15NO2S (M + Na)+ 356.0721, found 356.0729.
N-(4-Chlorophenylsulfonyl)-2-phenylindole (2c)4b. White solid, mp: 155–157 °C (Lit. 152–158 °C); Rf = 0.59 (10% EtOAc/petroleum ether); 1H NMR (600 MHz, CDCl3) δ 6.56 (s, 1H), 7.21 (d, J = 9.0 Hz, 2H), 7.27 (d, J = 8.4 Hz, 3H), 7.36 (t, J = 7.8 Hz, 1H), 7.42–7.49 (m, 6H), 8.29 (d, J = 8.4 Hz, 1H). 13C NMR (150 MHz, CDCl3) δ 113.1, 115.7, 119.9, 123.7, 124.0, 126.6, 127.2, 127.8, 127.9, 129.2, 129.6, 131.1, 134.7, 137.2, 139.2, 141.0; HRMS (ESI): m/z calcd for C20H14ClNO2S (M + Na)+ 390.0331, found 390.0333.
N-Ns-2-phenylindole (2d)4b. Light yellow solid, mp: 142–144 °C (Lit. 140–147 °C); Rf = 0.37 (10% EtOAc/petroleum ether); 1H NMR (600 MHz, CDCl3) δ 6.60 (s, 1H), 7.30 (t, J = 7.2 Hz, 1H), 7.39 (t, J = 8.4 Hz, 1H), 7.44–7.50 (m, 6H), 7.53 (d, J = 9.0 Hz, 1H), 8.08 (d, J = 9.0 Hz, 2H), 8.29 (d, J = 8.4 Hz, 1H). 13C NMR (150 MHz, CDCl3) δ 114.8, 116.7, 121.2, 123.8, 125.2, 125.4, 127.8, 128.1, 129.1, 130.2, 130.8, 131.7, 138.1, 141.9, 142.3, 150.4; HRMS (ESI): m/z calcd for C20H14N2O4S (M + Na)+ 401.0572, found 401.0581.
N-Ms-2-phenylindole (2f)4b. White solid, mp: 100–102 °C (Lit. 107–108 °C); Rf = 0.38 (10% EtOAc/petroleum ether); 1H NMR (600 MHz, CDCl3) δ 2.71 (s, 3H), 6.70 (s, 1H), 7.35 (t, J = 7.4 Hz, 1H), 7.38 (t, J = 7.6 Hz, 1H), 7.41–7.42 (m, 3H), 7.54–7.55 (m, 2H), 7.59 (d, J = 7.4 Hz, 1H), 8.12 (d, J = 8.2 Hz, 1H). 13C NMR (150 MHz, CDCl3) δ 38.4, 112.0, 114.8, 120.0, 123.5, 124.1, 126.7, 127.9, 129.1, 129.3, 131.0, 137.0, 141.0; HRMS (ESI): m/z calcd for C15H13NO2S (M + Na)+ 294.0565, found 294.0570.
N-Ts-2-o-tolylindole (2j)4b. White solid, mp: 90–92 °C (Lit. 82–89 °C); Rf = 0.54 (10% EtOAc/petroleum ether); 1H NMR (600 MHz, CDCl3) δ 2.21 (s, 3H), 2.31 (s, 3H), 6.46 (s, 1H), 7.08–7.10 (m, 3H), 7.20 (t, J = 7.4 Hz, 1H), 7.27 (t, J = 8.1 Hz, 2H), 7.35–7.37 (m, 4H), 7.49 (d, J = 7.6 Hz, 1H), 8.33 (d, J = 8.3 Hz, 1H). 13C NMR (150 MHz, CDCl3) δ 19.5, 20.5, 111.3, 114.7, 119.6, 122.8, 123.5, 123.6, 125.9, 128.1, 128.3, 128.6, 129.0, 129.8, 131.1, 134.6, 136.3, 138.3, 139.3, 143.6; HRMS (ESI): m/z calcd for C22H19NO2S (M + Na)+ 384.1034, found 384.1049.
N-Ts-2-m-tolylindole (2k)4b. White solid, mp: 144–147 °C (Lit. 140–144 °C); Rf = 0.43 (10% EtOAc/petroleum ether); 1H NMR (600 MHz, CDCl3) δ 2.28 (s, 3H), 2.41 (s, 3H), 6.52 (s, 1H), 7.03 (d, J = 7.7 Hz, 2H), 7.24–7.30 (m, 7H), 7.34 (t, J = 8.0 Hz, 1H), 7.43 (d, J = 7.6 Hz, 1H), 8.30 (d, J = 8.3 Hz, 1H). 13C NMR (151 MHz, CDCl3) δ 21.4, 21.6, 113.4, 116.6, 120.7, 124.3, 124.7, 126.8, 127.4, 129.1, 129.4, 130.5, 131.1, 132.3, 134.7, 137.0, 138.2, 142.3, 144.5; HRMS (ESI): m/z calcd for C22H19NO2S (M + Na)+ 384.1034, found 384.1016.
N-Ts-2-p-tolylindole (2l)4b. White solid, mp: 98–101 °C (Lit. 99–103 °C); Rf = 0.46 (10% EtOAc/petroleum ether); 1H NMR (600 MHz, CDCl3) δ 2.27 (s, 3H), 2.43 (s, 3H), 6.50 (s, 1H), 7.04 (d, J = 7.7 Hz, 2H), 7.23–7.28 (m, 5H), 7.33 (t, J = 7.7 Hz, 1H), 7.40 (t, J = 8.9 Hz, 3H), 8.30 (d, J = 8.3 Hz, 1H). 13C NMR (150 MHz, CDCl3) δ 21.4, 21.5, 113.3, 116.7, 120.6, 124.3, 124.6, 126.8, 128.3, 129.2, 129.5, 130.2, 130.7, 134.7, 138.2, 138.6, 142.3, 144.4; HRMS (ESI): m/z calcd for C22H19NO2S (M + Na)+ 384.1034, found 384.1024.
N-Ts-2-(2-methoxyphenyl)indole (2m)4b. White solid, mp: 72–75 °C (Lit. 77–80 °C); Rf = 0.50 (10% EtOAc/petroleum ether); 1H NMR (600 MHz, CDCl3) δ 2.29 (s, 3H), 3.77 (s, 3H), 6.53 (s, 1H), 6.95 (d, J = 8.2 Hz, 1H), 6.99 (t, J = 7.3 Hz, 1H), 7.07 (d, J = 7.9 Hz, 2H), 7.20–7.24 (m, 2H), 7.31 (t, J = 7.7 Hz, 1H), 7.39 (d, J = 7.8 Hz, 2H), 7.43 (t, J = 7.9 Hz, 1H), 7.47 (d, J = 7.7 Hz, 1H), 8.22 (d, J = 8.3 Hz, 1H). 13C NMR (150 MHz, CDCl3) δ 21.5, 55.5, 110.5, 112.4, 115.6, 119.6, 120.7, 121.9, 123.6, 124.4, 126.8, 129.2, 130.2, 130.6, 131.8, 135.8, 137.4, 138.0, 144.3, 158.6; HRMS (ESI): m/z calcd for C22H19NO3S (M + Na)+ 400.0983, found 400.0978.
N-Ts-2-(3-methoxyphenyl)indole (2n)4b. White solid, mp: 100–102 °C (Lit. 98–104 °C); Rf = 0.46 (10% EtOAc/petroleum ether); 1H NMR (600 MHz, CDCl3) δ 2.27 (s, 3H), 3.85 (s, 3H), 6.54 (s, 1H), 6.99 (dd, J = 8.2 Hz, 1H), 7.04 (d, J = 8.4 Hz, 3H), 7.09 (d, J = 7.4 Hz, 1H), 7.25–7.36 (m, 5H), 7.44 (d, J = 7.7 Hz, 1H), 8.31 (d, J = 8.4 Hz, 1H). 13C NMR (150 MHz, CDCl3) δ 21.2, 55.0, 113.3, 114.2, 115.6, 116.4, 120.4, 122.5, 124.0, 124.5, 126.5, 128.2, 128.9, 130.2, 133.3, 134.3, 138.0, 141.6, 144.2, 158.4; HRMS (ESI): m/z calcd for C22H19NO3S (M + Na)+ 400.0983, found 400.0982.
N-Ts-2-(4-methoxyphenyl)indole (2o)4b. White solid, mp: 131–133 °C (Lit. 126–128 °C); Rf = 0.42 (10% EtOAc/petroleum ether); 1H NMR (600 MHz, CDCl3) δ 2.27 (s, 3H), 3.88 (s, 3H), 6.47 (s, 1H), 6.94 (d, J = 7.74 Hz, 2H), 7.03 (d, J = 7.4 Hz, 2H), 7.25–7.26 (m, 3H), 7.32 (t, J = 7.3 Hz, 1H), 7.41 (d, J = 7.5 Hz, 3H), 8.30 (d, J = 8.1 Hz, 1H). 13C NMR (150 MHz, CDCl3) δ 20.5, 55.3, 111.9, 112.0, 115.7, 119.4, 123.2, 123.5, 123.7, 125.7, 128.1, 129.6, 130.6, 133.6, 137.1, 141.0, 143.4, 159.0; HRMS (ESI): m/z calcd for C22H19NO3S (M + Na)+ 400.0983, found 400.0981.
N-Ts-2-p-tert-butylindole (2p)6a. White solid, mp: 140–143 °C (Lit. 139–143 °C); Rf = 0.71 (10% EtOAc/petroleum ether); 1H NMR (600 MHz, CDCl3) δ 1.39 (s, 9H), 2.28 (s, 3H), 6.50 (s, 1H), 7.01 (d, J = 7.8 Hz, 2H), 7.23–7.27 (m, 3H), 7.33 (t, J = 7.8 Hz, 1H), 7.41–7.42 (m, 5H), 8.30 (d, J = 8.3 Hz, 1H). 13C NMR (150 MHz, CDCl3) δ 21.5, 31.4, 34.7, 113.3, 116.7, 120.6, 124.2, 124.4, 124.6, 126.8, 129.1, 129.4, 130.0, 130.6, 134.7, 138.3, 142.3, 144.4, 151.7; HRMS (ESI): m/z calcd for C25H25NO2S (M + Na)+ 426.1504, found 426.1503.
N-Ts-2-cyclopropylindole (2q)6b. Light yellow oil. Rf = 0.43 (5% EtOAc/petroleum ether); 1H NMR (600 MHz, CDCl3) δ 0.59 (d, J = 5.1 Hz, 2H), 0.95 (d, J = 8.0 Hz, 2H), 2.34 (s, 2H), 2.42–2.46 (m, 1H), 6.17 (s, 1H), 7.19–7.20 (m, 3H), 7.25 (t, J = 7.6 Hz, 1H), 7.38 (d, J = 7.6 Hz, 1H), 7.71 (d, J = 7.8 Hz, 2H), 8.21 (d, J = 8.3 Hz, 1H). 13C NMR (150 MHz, CDCl3) δ 7.33, 8.40, 20.5, 105.0, 113.5, 119.1, 122.3, 122.8, 125.6, 128.2, 128.6, 135.5, 136.3, 143.0, 143.5; HRMS (ESI): m/z calcd for C18H18NO2S (M + H)+ 312.1058, found 312.1049.
N-Ts-2-(1-naphthyl)indole (2r)4b. White solid, mp: 139–143 °C (Lit. 138–142 °C); Rf = 0.45 (10% EtOAc/petroleum ether); 1H NMR (600 MHz, CDCl3) δ 2.25 (s, 3H), 6.65 (s, 1H), 6.96 (d, J = 7.8 Hz, 2H), 7.25 (d, J = 8.1 Hz, 2H), 7.31 (t, J = 7.4 Hz, 2H), 7.40–7.46 (m, 3H), 7.51 (t, J = 7.7 Hz, 1H), 7.54 (d, J = 7.7 Hz, 1H), 7.64 (d, J = 8.3 Hz, 1H), 7.87 (d, J = 8.2 Hz, 1H), 7.95 (d, J = 8.2 Hz, 1H), 8.40 (d, J = 8.4 Hz, 1H). 13C NMR (150 MHz, CDCl3) δ 21.5, 113.7, 115.8, 120.8, 123.9, 124.4, 124.8, 125.8, 126.1, 126.2, 126.9, 128.0, 129.3, 129.4, 129.5, 129.8, 130.0, 133.0, 133.4, 135.3, 137.6, 138.8, 144.5; HRMS (ESI): m/z calcd for C25H19NO2S (M + Na)+ 420.1034, found 420.1035.
N-Ts-indole (2s)4b. White solid, mp: 57–60 °C (Lit. 68–71 °C); Rf = 0.45 (10% EtOAc/petroleum ether); 1H NMR (600 MHz, CDCl3) δ 2.33 (s, 3H), 6.65 (d, J = 2.9 Hz, 1H), 7.20–7.28 (m, 3H), 7.30 (t, J = 7.7 Hz, 1H), 7.52 (d, J = 7.6 Hz, 1H), 7.56 (d, J = 3.0 Hz, 1H), 7.76 (d, J = 7.9 Hz, 2H), 7.99 (d, J = 8.3 Hz, 1H). 13C NMR (150 MHz, CDCl3) δ 20.5, 108.0, 112.5, 120.3, 122.2, 123.5, 125.3, 125.8, 128.8, 129.7, 133.8, 134.3, 143.9; HRMS (ESI): m/z calcd for C15H13NO2S (M + Na)+ 294.0565, found 294.0598.
N-Ts-2-(4-fluorophenyl)indole (2t)6a. White solid, mp: 130–132 °C (Lit. 134–137 °C); Rf = 0.62 (10% EtOAc/petroleum ether); 1H NMR (600 MHz, CDCl3) δ 2.28 (s, 3H), 6.52 (s, 1H), 7.04 (d, J = 7.8 Hz, 2H), 7.10 (t, J = 8.3 Hz, 2H), 7.24–7.27 (m, 3H), 7.35 (t, J = 7.7 Hz, 1H), 7.43–7.46 (m, 3H), 8.31 (d, J = 8.4 Hz, 1H). 13C NMR (150 MHz, CDCl3) δ 21.5, 113.6, 114.5 (d, J = 21.7 Hz), 116.6, 120.7, 124.4, 124.9, 126.7, 128.4 (d, J = 3.2 Hz), 129.3, 130.4, 132.1 (d, J = 8.2 Hz), 134.7, 138.2, 140.9, 144.7, 163.9 (d, J = 247 Hz); HRMS (ESI): m/z calcd for C21H16FNO2S (M + Na)+ 388.0783, found 388.0776.
N-Ts-2-(4-chlorophenyl)indole (2u)4b. Light yellow solid, mp: 134–137 °C (Lit. 133–135 °C); Rf = 0.61 (10% EtOAc/petroleum ether); 1H NMR (600 MHz, CDCl3) δ 2.29 (s, 3H), 6.54 (s, 1H), 7.06 (d, J = 7.7 Hz, 2H), 7.26 (d, J = 5.1 Hz, 3H), 7.35–7.40 (m, 3H), 7.44 (d, J = 7.8 Hz, 3H), 8.30 (d, J = 8.3 Hz, 1H). 13C NMR (150 MHz, CDCl3) δ 20.5, 113.0, 115.7, 119.8, 123.4, 124.0, 125.7, 126.8, 128.2, 129.4, 129.8, 130.5, 133.5, 133.8, 137.3, 139.8, 143.7; HRMS (ESI): m/z calcd for C21H16ClNO2S (M + Na)+ 404.0488, found 404.0488.
N-Ts-5-methyl-2-phenylindole (2w)4b. White solid, mp: 100–103 °C (Lit. 114–115 °C); Rf = 0.50 (10% EtOAc/petroleum ether); 1H NMR (600 MHz, CDCl3) δ 2.28 (s, 3H), 2.41 (s, 1H), 6.47 (s, 1H), 7.04 (d, J = 7.8 Hz, 2H), 7.16 (d, J = 8.4 Hz, 1H), 7.22 (s, 1H), 7.25 (s, 2H), 7.40–7.42 (m, 3H), 7.48–7.50 (m, 2H), 8.17 (d, J = 8.4 Hz, 1H). 13C NMR (150 MHz, CDCl3) δ 21.3, 21.5, 113.6, 116.4, 120.6, 126.2, 126.8, 127.5, 128.6, 129.2, 130.3, 130.8, 132.5, 134.0, 134.6, 136.5, 142.3, 144.4; HRMS (ESI): m/z calcd for C22H19NO2S (M + Na)+ 384.1034, found 384.1029.
N-Ts-5-methoxy-2-phenylindole (2x)6a. White solid, mp: 114–117 °C (Lit. 123–125 °C); Rf = 0.48 (10% EtOAc/petroleum ether); 1H NMR (600 MHz, CDCl3) δ 2.20 (s, 3H), 3.74 (s, 3H), 6.39 (s, 1H), 6.80 (s, 1H), 6.88 (d, J = 9.1 Hz, 1H), 6.96 (d, J = 7.9 Hz, 2H), 7.16 (d, J = 7.8 Hz, 2H), 7.34–7.41 (m, 5H), 8.12 (d, J = 9.0 Hz, 1H). 13C NMR (150 MHz, CDCl3) δ 21.5, 55.6, 103.1, 113.4, 113.9, 117.7, 126.8, 127.5, 128.6, 129.1, 130.2, 131.7, 132.4, 132.8, 134.3, 143.1, 144.4, 157.0; HRMS (ESI): m/z calcd for C22H19NO3S (M + Na)+ 400.0983, found 400.0983.
N-Ts-5-fluoro-2-phenylindole (2y)6a. Light yellow solid, mp: 104–106 °C (Lit. 110–112 °C); Rf = 0.57 (10% EtOAc/petroleum ether); 1H NMR (600 MHz, CDCl3) δ 2.29 (s, 3H), 6.49 (s, 1H), 7.05 (d, J = 7.9 Hz, 2H), 7.08 (d, J = 9.0 Hz, 2H), 7.24 (d, J = 7.9 Hz, 2H), 7.42–7.44 (m, 3H), 7.49 (d, J = 7.0 Hz, 2H), 8.26 (dd, J = 4.4, 8.8 Hz, 1H). 13C NMR (150 MHz, CDCl3) δ 20.5, 105.2 (d, J = 23.9 Hz), 111.5 (d, J = 24.9 Hz), 112.3 (d, J = 3.4 Hz), 116.9 (d, J = 9.3 Hz), 125.7, 126.5, 127.9, 128.3, 129.2, 130.6, 131.0, 133.2, 133.5, 142.9, 143.7, 158.3; HRMS (ESI): m/z calcd for C21H16FNO2S (M + Na)+ 388.0783, found 388.0783.
N-Ts-5-chloro-2-phenylindole (2z)4b. White solid, mp: 138–140 °C (Lit. 136–137 °C); Rf = 0.61 (10% EtOAc/petroleum ether); 1H NMR (600 MHz, CDCl3) δ 2.30 (s, 3H), 6.47 (s, 1H), 7.05 (d, J = 7.9 Hz, 2H), 7.24 (d, J = 7.9 Hz, 2H), 7.30 (d, J = 8.8 Hz, 1H), 7.40–7.45 (m, 4H), 7.47 (d, J = 7.1 Hz, 2H), 8.23 (d, J = 8.8 Hz, 1H). 13C NMR (150 MHz, CDCl3) δ 21.5, 112.6, 117.7, 120.3, 124.9, 126.8, 127.6, 129.0, 129.3, 130.0, 130.4, 131.7, 131.9, 134.5, 136.6, 143.6, 144.8; HRMS (ESI): m/z calcd for C21H16ClNO2S (M + Na)+ 404.0488, found 404.0488.
N-Ts-2-phenyl-5-(trifluoromethyl)indole (2aa)4b. White solid, mp: 115–118 °C (Lit. 120–124 °C); Rf = 0.67 (20% EtOAc/petroleum ether); 1H NMR (600 MHz, CDCl3) δ 2.31 (s, 3H), 6.59 (s, 1H), 7.08 (d, J = 7.7 Hz, 2H), 7.26 (d, J = 6.4 Hz, 2H), 7.44–7.47 (m, 5H), 7.60 (d, J = 8.7 Hz, 1H), 7.74 (s, 1H), 8.42 (d, J = 8.7 Hz, 1H). 13C NMR (150 MHz, CDCl3) δ 21.6, 112.7, 116.7, 118.1 (q, J = 4.0 Hz), 121.4 (q, J = 3.3 Hz), 125.4, 126.6 (q, J = 32.3 Hz), 126.8, 127.6, 129.1, 129.5, 130.0, 130.5, 131.6, 134.7, 139.6, 143.7, 145.1; HRMS (ESI): m/z calcd for C22H16F3NO2S (M + Na)+ 438.0752, found 438.0757.
N-Ts-6-fluoro-2-phenylindole (2ab)6a. White solid, mp: 97–100 °C (Lit. 103–105 °C); Rf = 0.61 (10% EtOAc/petroleum ether); 1H NMR (600 MHz, CDCl3) δ 2.30 (s, 3H), 6.50 (s, 1H), 7.02 (t, J = 8.7 Hz, 1H), 7.07 (d, J = 7.9 Hz, 2H), 7.26–7.28 (m, 3H), 7.36 (t, J = 6.6 Hz, 1H), 7.42–7.47 (m, 4H), 8.06 (d, J = 10.3 Hz, 1H). 13C NMR (150 MHz, CDCl3) δ 21.6, 104.1 (d, J = 28.5 Hz), 112.6 (d, J = 23.7 Hz), 121.3 (d, J = 9.7 Hz), 126.7, 126.8, 127.5, 128.8, 129.3, 130.3, 132.1, 134.6, 138.5 (d, J = 12.4 Hz), 142.4, 144.8, 160.1, 161.7; HRMS (ESI): m/z calcd for C21H16FNO2S (M + Na)+ 388.0783, found 388.0783.
N-Ts-6-chloro-2-phenylindole (2ac)4b. White solid, mp: 137–140 °C (Lit. 140–144 °C); Rf = 0.57 (10% EtOAc/petroleum ether); 1H NMR (600 MHz, CDCl3) δ 2.30 (s, 3H), 6.49 (s, 1H), 7.06 (d, J = 7.9 Hz, 2H), 7.24–7.26 (m, 3H), 7.35 (d, J = 8.2 Hz, 1H), 7.41–7.46 (m, 5H), 8.34 (s, 1H). 13C NMR (150 MHz, CDCl3) δ 21.6, 112.9, 116.7, 121.3, 124.9, 126.8, 127.5, 128.9, 129.4, 130.4, 130.6, 131.9, 134.5, 138.6, 142.6, 144.9 (one carbon signal was missing due to peak overlap); HRMS (ESI): m/z calcd for C21H16ClNO2S (M + Na)+ 404.0488, found 404.0488.
N-Ts-5-methyl-2-p-tolylindole (2ad)6a. White solid, mp: 144–146 °C (Lit. 143–145 °C); Rf = 0.50 (10% EtOAc/petroleum ether). 1H NMR (600 MHz, CDCl3) δ 2.28 (s, 3H), 2.40 (s, 3H), 2.44 (s, 3H), 6.44 (s, 1H), 7.03 (d, J = 7.7 Hz, 2H), 7.15 (d, J = 8.5 Hz, 1H), 7.20 (s, 1H), 7.22–7.27 (m, 4H), 7.40 (d, J = 7.5 Hz, 2H), 8.16 (d, J = 8.4 Hz, 1H). 13C NMR (150 MHz, CDCl3) δ 21.3, 21.4, 21.5, 113.2, 116.4, 120.5, 126.0, 126.8, 128.2, 129.1, 129.7, 130.1, 130.9, 133.9, 134.6, 138.5, 144.3; HRMS (ESI): m/z calcd for C23H21NO2S (M + Na)+ 398.1191, found 398.1191.
N-Ts-5-trifluoromethyl-2-p-tolylindole (2ae)6a. White solid, mp: 148–150 °C; Rf = 0.65 (10% EtOAc/petroleum ether). 1H NMR (600 MHz, CDCl3) δ 2.31 (s, 3H), 2.45 (s, 3H), 6.56 (s, 1H), 7.07 (d, J = 7.7 Hz, 2H), 7.24–7.28 (m, 5H), 7.37 (d, J = 7.3 Hz, 2H), 7.58 (d, J = 8.8 Hz, 1H), 7.72 (s, 1H), 8.41 (d, J = 8.7 Hz, 1H). 13C NMR (150 MHz, CDCl3) δ 21.5, 21.6, 112.5, 116.7, 118.0 (d, J = 3.77 Hz), 121.2 (d, J = 3.4 Hz), 126.4, 126.6, 126.8, 128.3, 128.7, 129.4, 130.1, 130.4, 134.7, 139.2, 139.6, 143.9, 145.0; HRMS (ESI): m/z calcd for C23H18F3NO2S (M + Na)+ 452.0908, found 452.0917.
Acknowledgements
This project was partially sponsored by the Scientific Research Foundation for the Returned Overseas Chinese Scholars, State Education Ministry, the National Basic Research Project (No. 2014CB932201) and the National Natural Science Foundation of China (No. 21572154).
Notes and references
-
(a) R. J. Sundberg, Indoles, Academic Press, San Diego, 1996 Search PubMed;
(b) M. Somei and F. Yamada, Nat. Prod. Rep., 2004, 21, 278 RSC;
(c) A. J. Kochanowska-Karamyan and M. T. Hamann, Chem. Rev., 2010, 110, 4489 CrossRef CAS PubMed;
(d) J. E. Saxton, Nat. Prod. Rep., 1997, 14, 559 RSC;
(e) M. Bandini and A. Eichholzer, Angew. Chem., Int. Ed., 2009, 48, 9608 CrossRef CAS PubMed;
(f) N. Mushtaq, Z. S. Saify, F. Noor, S. Takween, S. Akhtar, M. Arif and K. M. Khan, Pak. J. Pharm. Sci., 2008, 21, 36 CAS.
- For selected reviews on indole synthesis, see:
(a) B. Robinson, Chem. Rev., 1963, 63, 373 CrossRef;
(b) G. R. Humphrey and J. T. Kuethe, Chem. Rev., 2006, 106, 2875 CrossRef CAS PubMed;
(c) M. Shiri, Chem. Rev., 2012, 112, 3508 CrossRef CAS PubMed;
(d) K. Kruger, A. Tillack and M. Beller, Adv. Synth. Catal., 2008, 350, 2153 CrossRef;
(e) M. Inmanand and C. J. Moody, Chem. Sci., 2013, 4, 29 RSC;
(f) I. Nakamura and Y. Yamamoto, Chem. Rev., 2004, 104, 2127 CrossRef CAS PubMed;
(g) D. F. Taber and P. K. Tirunahari, Tetrahedron, 2011, 67, 7195 CrossRef CAS PubMed.
- Pd-catalyzed indole synthesis reviews, see:
(a) S. Cacchi and G. Fabrizi, Chem. Rev., 2005, 105, 2873 CrossRef CAS PubMed;
(b) S. Cacchi and G. Fabrizi, Chem. Rev., 2011, 111, PR215 CrossRef PubMed; for selected recent examples of Rh-catalyzed indole synthesis, see:
(c) D. Shu, W. Song, X. Li and W. Tang, Angew. Chem., Int. Ed., 2013, 52, 3237 CrossRef CAS PubMed;
(d) C. Jones, Q. Nguyen and T. G. Driver, Angew. Chem., Int. Ed., 2014, 53, 785 CrossRef CAS PubMed;
(e) C. Wang and Y. Huang, Org. Lett., 2013, 15, 5294 CrossRef CAS PubMed;
(f) B. Liu, C. Song, C. Sun, S. Zhou and J. Zhu, J. Am. Chem. Soc., 2013, 135, 16625 CrossRef CAS PubMed;
(g) D. R. Stuart, P. Alsabeh, M. Kuhn and K. Fagnou, J. Am. Chem. Soc., 2010, 132, 18326 CrossRef CAS PubMed;
(h) N. Selander, B. T. Worrell, S. Chuprakov, S. Velaparthi and V. V. Fokin, J. Am. Chem. Soc., 2012, 134, 14670 CrossRef CAS PubMed.
- Recent metal-free examples:
(a) L. Fra, A. Millán, J. A. Souto and K. Muñiz, Angew. Chem., Int. Ed., 2014, 53, 7349 CrossRef CAS PubMed;
(b) H. J. Jang and S. W. Youn, Org. Lett., 2014, 16, 3720 CrossRef PubMed;
(c) S. Ortgies and A. Breder, Org. Lett., 2015, 17, 2748 CrossRef CAS PubMed;
(d) X. Zhang, R. Guo and X. Zhao, Org. Chem. Front., 2015, 2, 1334 RSC;
(e) V. T. Abaev, A. T. Plieva, P. N. Chalikidi, M. G. Uchuskin, I. V. Trushkov and A. V. Butin, Org. Lett., 2014, 16, 4150 CrossRef CAS PubMed;
(f) M. T. Hovey, C. T. Check, A. F. Sipher and K. A. Scheidt, Angew. Chem., Int. Ed., 2014, 53, 9603 CrossRef CAS PubMed;
(g) Z. He, H. Li and Z. Li, J. Org. Chem., 2010, 75, 4636 CrossRef CAS PubMed;
(h) Q. Q. Yang, C. Xiao, L. Q. Lu, J. An, F. Tan, B. J. Li and W. J. Xiao, Angew. Chem., Int. Ed., 2012, 51, 9137 CrossRef CAS PubMed;
(i) J. Barluenga, M. Trincado, E. Rubio and J. M. Gonzalez, Angew. Chem., Int. Ed., 2003, 42, 2406 CrossRef CAS PubMed;
(j) A. Correa, I. Tellitu, E. Domínguez and R. SanMartin, J. Org. Chem., 2006, 71, 8316 CrossRef CAS PubMed;
(k) X. Li, Y. Du, Z. Liang, X. Li, Y. Pan and K. Zhao, Org. Lett., 2009, 11, 2643 CrossRef CAS PubMed;
(l) W. Yu, Y. Du and K. Zhao, Org. Lett., 2009, 11, 2417 CrossRef CAS PubMed;
(m) S. L. Cui, J. Wang and Y. G. Wang, J. Am. Chem. Soc., 2008, 130, 13526 CrossRef CAS PubMed;
(n) D. McAusland, S. Seo, D. G. Pintori, J. Finlayson and M. F. Greaney, Org. Lett., 2011, 13, 3667 CrossRef CAS PubMed;
(o) D. Yue, T. Yao and R. C. Larock, J. Org. Chem., 2006, 71, 62 CrossRef CAS PubMed.
- For selected examples of indole synthesis through intramolecular amination reaction of alkenes
(a) T. W. Liwosz and S. R. Chemler, Chem.–Eur. J., 2013, 19, 12771 CrossRef CAS PubMed;
(b) S. Maity and N. Zheng, Angew. Chem., Int. Ed., 2012, 51, 9562 CrossRef CAS PubMed;
(c) M. Arthuis, R. Pontikis and J. C. Florent, Org. Lett., 2009, 11, 4608 CrossRef CAS PubMed;
(d) K. Okuma, T. Yasuda, I. Takeshita, K. Shioji and Y. Yokomori, Tetrahedron, 2007, 63, 8250 CrossRef CAS;
(e) K. Okuma, I. Takeshita, T. Yasuda and K. Shioji, Chem. Lett., 2006, 35, 1122 CrossRef CAS;
(f) R. Nallagonda, M. Rehan and P. Ghorai, Org. Lett., 2014, 16, 4786 CrossRef CAS PubMed;
(g) L. S. Hegedus, G. F. Allen, J. J. Bozell and E. L. Waterman, J. Am. Chem. Soc., 1978, 100, 5800 CrossRef CAS;
(h) P. J. Harrington, L. S. Hegedus and K. F. McDaniel, J. Am. Chem. Soc., 1987, 109, 4335 CrossRef CAS;
(i) D. Tsvelikhovsky and S. L. Buchwald, J. Am. Chem. Soc., 2010, 132, 14048 CrossRef CAS PubMed and also see ref. 4a and b.
-
(a) Y.-L. Li, J. Li, A.-L. Ma, Y.-N. Huang and J. Deng, J. Org. Chem., 2015, 8, 3841 CrossRef PubMed;
(b) Y.-L. Li, J. Li, S.-N. Yu, J.-B. Wang, Y.-M. Yu and J. Deng, Tetrahedron, 2015, 71, 8271 CrossRef CAS.
- For some reviews:
(a) S. S. Stahl, Science, 2005, 309, 1824 CrossRef CAS PubMed;
(b) S. S. Stahl, Angew. Chem., Int. Ed., 2004, 43, 3400 CrossRef CAS PubMed;
(c) B. M. Stoltz, Chem. Lett., 2004, 33, 362 CrossRef CAS;
(d) M. J. Schultz and M. S. Sigman, Tetrahedron, 2006, 62, 8227 CrossRef CAS;
(e) J. Muzart, Asian J. Chem., 2006, 1, 508 CrossRef CAS PubMed;
(f) M. S. Sigman and D. R. Jensen, Acc. Chem. Res., 2006, 39, 221 CrossRef CAS PubMed;
(g) E. M. Beccalli, G. Broggini, M. Martinelli and S. Sottocornola, Chem. Rev., 2007, 107, 5318 CrossRef CAS PubMed;
(h) J. Piera and J. E. Bäckvall, Angew. Chem., Int. Ed., 2008, 47, 3506 CrossRef CAS PubMed;
(i) K. M. Gligorich and M. S. Sigman, Chem. Commun., 2009, 3854 RSC;
(j) Z. Shi, C. Zhang, C. Tang and N. Jiao, Chem. Soc. Rev., 2012, 41, 3381 RSC;
(k) N. Gulzar, B. Schweitzer-Chaput and M. Klussmann, Catal. Sci. Technol., 2014, 4, 2778 RSC.
-
(a) A. A. Frimer, Chem. Rev., 1979, 79, 359 CrossRef CAS;
(b) K. Chen, P. Zhang, Y. Wang and H. Li, Green Chem., 2014, 16, 2344 RSC;
(c) S. Park, W. H. Jeon, W.-S. Yong and P. H. Lee, Org. Lett., 2015, 17, 5060 CrossRef CAS PubMed;
(d) R. Lin, F. Chen and N. Jiao, Org. Lett., 2012, 14, 4158 CrossRef CAS PubMed and ref. 5b.
- J. Liu, X. Xie and Y. Liu, Chem. Commun., 2013, 49, 11794 RSC.
Footnote |
† Electronic supplementary information (ESI) available: Copies of 1H and 13C spectra of all products. See DOI: 10.1039/c6ra03378d |
|
This journal is © The Royal Society of Chemistry 2016 |
Click here to see how this site uses Cookies. View our privacy policy here.