DOI:
10.1039/C6RA04614B
(Paper)
RSC Adv., 2016,
6, 40527-40538
Palonosetron attenuates 1,2-dimethyl hydrazine induced preneoplastic colon damage through downregulating acetylcholinesterase expression and up-regulating synaptic acetylcholine concentration
Received
21st February 2016
, Accepted 14th April 2016
First published on 15th April 2016
Abstract
The present study was undertaken to evaluate the effect of palonosetron (PAL) against 1,2-dimethylhydrazine (DMH)-induced colon cancer. Wistar albino rats were randomly divided into four groups (n = 8). Group 1 served as normal control (1 mM EDTA + saline, 2 ml per kg per day, s.c.); group 2 toxic control; group 2, 3 and 4 received DMH (20 mg per kg per week, s.c.), for 6 weeks; groups 3 and 4 also received PAL (0.25 and 0.50 mg per kg per day, p.o) for 6 weeks. DMH treated rats showed altered heart rate variability (HRV) factors, increased incidence of aberrant crypt foci (ACF), distorted antioxidant markers (TBAR's, SOD, catalase, GSH) and increased levels inflammatory markers (COX). The colonic surface architecture, studied using scanning electron microscopy (SEM), revealed aberrant crypts (500×) and preneoplastic nodules (2000×). PAL treatment helped to minimize the ACF count, and restored oxidative and inflammatory markers favorably. To further validate our results, we directed our study to define the effect of PAL on acetylcholine (Ach) neurotransmission using a simple model organism, C. elegans. Increased cholinergic transmission by PAL (8 μM) was evident in the worms when studied through an aldicarb assay. However, PAL (2 μM, 4 μM and 8 μM) treatment negatively modulated nAchR, when evaluated using the levamisole assay. The increased synaptic Ach levels can be attributed to the decreased levels of acetylcholinesterase (AchE), which could be attributed to the decreased genomic levels of ace-1 and ace-2. The above findings were also supported by the fact, that we observed decreased AchE activity in PAL treated rats. In addition the downregulation in the expression of unc-38 (one of the necessary components of nAchR) sufficiently links with the decreased nAchR activity. Our findings emphasize the potential role of PAL in the suppression of colon carcinogenesis.
1. Introduction
The cholinergic anti-inflammatory pathway has been considered to be an additional endogenous mechanism to regulate the immune system and provide immediate modulatory input on the inflammatory cells. The physiological importance of the cholinergic anti-inflammatory pathway has recently immerged more strongly due to researchers indicating that stimulation of the vagus nerve could modulate systemic inflammation and the immune response.1 A plethora of evidences are available supporting the information that activation of the vagus nerve or mimicking the cholinergic anti-inflammatory pathway can be exploited in variable disorders.2,3 Recent studies have also demonstrated that activating the cholinergic anti-inflammatory pathway with a pharmacological agent or through vagus nerve stimulation, can confer anti-inflammatory effects both locally and systematically.2,3 In fact, extensive research on guanyl hydrazone (CNI-1493) (centrally acting pharmacological agent) was reported to anti-inflammatory activities in experimental model of cancer, pancreatitis and arthritis.4 Studies have also demonstrated that the peripheral anti-inflammatory effect of CNI-1493 are mediated through vagus nerve firing and subsequently modulated cholinergic anti-inflammatory pathway. These findings had led CNI-1493 to clinical trial for the management of Crohn's disease.5
The α-7 subunit of the nAchR has been accredited as the mediator of the cholinergic anti-inflammatory pathway through direct modulation or vagus nerve firing.6 The α-7nAchR signaling is also reported to reduce macrophagic cytokine production, inflammation and colitis. Henceforth, vagus nerve stimulation has immersed as an alternative therapy for the management of digestive disorders.7–9
Inflammation contributes to carcinogenesis that develops from the subthreshold neoplastic state caused by a chemical agent which further induces the somatic changes. The state of initiation, involves deoxyribonucleic acid (DNA) damage is followed by promotion which generates a microenvironment in favor of sustained growth, angiogenesis and metastasis.10 Tumor cells produce various cytokines and chemokines that attract leucocytes (neutrophils, dendritic cells, macrophages etc.) which are capable of producing array of cytokines mediators, serine and cysteine protease, membrane perforating agent, TNF-α, interleukins and interferon's.11
Considering the role of cholinergic anti-inflammatory pathway in variable inflammatory diseases, we hypothesize that the positive modulation of cholinergic neurotransmission may exert significant effect in the colon cancer through parasympathetic signaling/cholinergic anti-inflammatory pathway. Considering the same the present work was undertaken to enumerate the effect of palonosetron (PAL) (partial agonist of α-7nAchR) on preneoplastic colonic lesion in albino rats. The study enumerates the effect of PAL, besides initiating from rodent models and summing up to simple nematodes for elucidation of molecular events involved in the hypothesized mechanism.
2. Materials and methods
2.1. Drugs and chemicals
PAL (Ajanta pharma limited, Kandivli, Mumbai, India) was purchased from local market. 1,2-Dimethyl hydrazine (DMH) was procured from ACROS Organics, Thermo Fisher Scientific, New Jersey, US. All other chemicals were of analytical grade and procured from Hi-media Laboratories, Mumbai, India; else otherwise stated in the text.
2.2. Animals
Wistar strain of albino rats (100–120 g) were procured from the central animal house. Animals were housed under standard conditions of temperature (25 ± 1 °C) with 12 h light/dark cycle and free access to commercial pellet diet and water ad libitum. Animals were given weeks' time to get acclimatized with the laboratory conditions. The study was conducted as per guidelines issued from Department of Animal Welfare, Government of India and approved by the institutional animal ethics committee (approval no. SDCOP&VS/AH/CPCSEA/01/0031).
2.3. Experimental protocol
Animals were divided into 4 groups of 8 animals each. Group 1 served as normal control (1 mM EDTA + saline, 2 ml per kg per day, s.c.); group 2 toxic control (DMH – 20 mg per kg per week, s.c.); group 3 and 4 received, DMH (20 mg per kg per week, s.c.), for 6 weeks followed by PAL (0.25 and 0.50 mg per kg per day, p.o) for 6 weeks. The DMH (dissolved in 1 mM EDTA saline) was administered (s.c.) as weekly injection for six weeks with daily administration of PAL (p.o.).
The animals were recorded for the electrocardiogram (ECG) and HRV paradigms on 42nd day after the last dosing of PAL, using the protocol specified in forthcoming section. Subsequently animals were sacrificed on 43rd day under light ether anesthesia. The intestinal tissue was collected by securing the both ends with a surgical suture (to prevent drainage of the content). The intestinal content and the tissues were further scrutinized against different markers.
2.4. Hemodynamic changes
The animals were anaesthetized using ketamine hydrochloride (100 mg kg−1, i.m.) and diazepam (5 mg kg−1, i.m.) combination, and subsequently mounted on a wax tray. The platinum hook electrodes were placed on the skin of the dorsal and ventral thorax to record the ECG signal. The electrodes were connected to Bio-amplifier (ML-136) and channel power lab (ML-826) to convert analogue to digital signals (AD Instruments, Australia). The ECG signals were saved on the hard disk and analyzed offline using Lab Chart Pro-8 (AD Instruments, Australia).
HRV analysis was conducted on multiple segments of continuous ECG signals perceived in the preceding section. Firstly, all the raw signals were inspected manually to ensure that all the R waves are detected correctly. Subsequently, HR was calculated by plotting the number of R waves per unit time. Following the same, time and frequency domain parameters of HRV were also calculated using the Lab Chart Pro-8 (AD Instruments, Australia).12
2.5. Weight variation
Weight variations among all the groups were determined by following formula:
% Weight variation = [(final weight − initial weight)/final weight] × 100 |
2.6. Estimation of pH and total acidity
After the respective treatments, animals were euthanized with cervical dislocation and the intestinal tissue was collected. The content of the intestinal tissue was collected and evaluated for intestinal pH using a pen type pH meter (Hanna Instrument HI 98107). Total acidity was evaluated using the procedure described previously and expressed as mEq.13,14
2.7. Aberrant crypt foci (ACF)
ACF analysis was performed following the method described by Bird. Briefly colon tissues were longitudinally opened, rinsed with saline and fixed flat between two pieces of Whatman paper in buffered formalin (10%) for 24 h. Tissues were placed on a microscope slide and stained with 0.2% methylene blue in phosphate buffer saline for 10 min. The tissues were visualized through a light microscope at 40× magnification. ACF was characterized by nearby normal crypts through increase in size, increased distance from lamina and discernible pericryptal zone. ACF in total and number in crypts in each focus were counted for at least two focuses per slide. ACF were characterized by crypt multiplicity (1, 2, 3 or ≥4).15,16
2.8. Antioxidant markers
The distal part of the intestinal tissues (10% w/v) was homogenized in 0.15 M KCl and centrifuged at 10
000 rpm. The supernatants were scrutinized for biochemical parameters including thiobarbituric acid reactive substances (TBARS), superoxide dismutase (SOD), acetylcholine esterase (AchE), catalase and glutathione (GSH) using the methods established at our laboratory.17–22
2.9. Cyclooxygenase (COX) and lipoxygenase (LOX) estimations
Tissue homogenate (10%) in TRIS buffer (50 mM) was centrifuged at 5000 rpm for 5 min followed by sonication. The tissue supernatant (10 μl) was incubated for 5 min with TRIS buffer (160 μl). A 10 μl each of TMPD reagent and arachidonic acid (AA) solution were added and read at 630 nm using multiplate reader (ALERE Microplate Reader, AM-2100) at 0 and 30 s interval for COX assay. AA solution was prepared by mixing 50 μl of the 40 mM AA with 50 μl of 0.1 N potassium hydroxide using vortexing and subsequently 900 μl of double distilled water. TMPD stock solution was prepared by dissolving 0.3 mg in 1 ml of distilled water and subsequent 1
:
10 dilution was prepared for the assay.23,24 The assay was performed in the whole serum and therefore reflects the nonspecific COX activity.
For LOX assay, 25 μl of AA solution was added to the 475 μl supernatant (as prepared for COX assay) and incubated for 6 min. A 500 μl of ferrithiocyanate (FTC) reagent was added and read at 480 nm using UV spectrophotometer (Cary 60, Agilent Technologies International Private Limited, CA United States) after 5 min. FTC reagent was prepared by mixing the reagent 1 (4.5 mM FeSO4 in 0.2 M HCl) and reagent 2 (3% NH4SCN in methanolic solution) in 1
:
1 ratio.25
2.10. Morphological evaluation
The intestinal tissues from all the groups were evaluated for their morphological changes using scanning electron microscopy. Samples were fixed in 2.5% glutaraldehyde for 6 h at 4 °C and washed with 0.1 M phosphate buffer, for 3 changes each of 15 min at 4 °C. 1% osmium tetroxide was used as a post fixative for 2 h at 4 °C and samples were washed in 0.1 M phosphate buffer for 3 changes each of 15 min at 4 °C to remove the unreacted fixative. Specimens were dehydrated by using increasing concentrations of acetone viz. 30%, 50%, 70%, 90%, 95%, 100% (dry acetone) to remove water at 4 °C for 30 min periods. Subsequently, samples were air dried (critical point i.e. at 1100 psi). The specimens were mounted on to the aluminum stub with adhesive tape and the specimens were observed using a scanning electron microscope (JEOL JSM-6490LV).26
2.11. Histopathology
Colon tissues were evaluated histopathologically using haematoxylin and eosin staining. The tissues were fixed in paraformaldehyde for overnight, succeeded by 70% isopropanol overnight. Tissues were further exposed to augmenting concentration of isopropanol (70%, 90%, and 100%), superseded by dehydration with 100% xylene. The tissues were embedded in paraffin wax and blocks were prepared. 5 μm sections were prepared using microtome followed by staining with haematoxylin and eosin. The sections were visualize and photographed at 40× using digital biological microscope (N120, BR-Biochem Life Sciences, New Delhi, India).27
2.12. Culture and maintenance of strains
C. elegans strains, Bristol N2 (wild type), were procured from Caenorhabditis Genetics Center, (University of Minnesota, MN, USA) and grown on nematode growth medium (NGM), using Escherichia coli OP50 as a food source. All experiments were conducted at 22 °C.
2.13. Aldicarb assay
Aldicarb assay was done as described in previous literature.28 Considering lack of studies on C. elegans with PAL, dose titration study ranging from 1 μM to 32 μM of PAL was performed for aldicarb and levamisole assay (data not represented). No significant variation could be recorded between the 8 μM, 16 μM and 32 μM concentration of PAL and therefore further studies were confined for the concentration range of 2 μM to 8 μM. Briefly the age-synchronized embryos were added to treatment plates and incubated at 22 °C for 48 h. Adult worms were washed thrice from treatment plates using M9 buffer. A fixed number of worms were transferred to 0.5 mM aldicarb NGM plates. The worms were scored for paralysis every 30 min and were prodded using eye lash. As a convention the worms that lacked movement even after prodding thrice were considered as paralyzed. Any worms lost or damaged were disregarded from the study. The experiment was done in duplicate sets and percentage of worms paralyzed was calculated.
2.14. Levamisole assay
Levamisole assay was performed as described by Qian et al.29 with some modifications. Briefly the age synchronized embryos were added to treatment plates and incubated at 22 °C for 48 h. Adult worms were washed thrice from treatment plates using M9 buffer. Approximately 20 to 30 worms were transferred to each well of 96 well plates. Equal volume of 50 μM levamisole solution was added to each well and worms were scored for paralysis. The worms that lacked movement were considered as paralyzed. Percentage of worms paralyzed at every time interval was calculated.
2.15. Quantification of gross acetylcholine (Ach) levels
Ach levels were determined using Amplex Red Ach/AchE (Catalogue no A12217, Thermo Fischer Scientific) estimation kit according to the manufacturer's protocol. Briefly the age-synchronized embryos were added to treatment plates and incubated at 22 °C for 48 h. Adult worms were washed thrice from treatment plates using M9 buffer and sonicated in 1× reaction buffer (supplied in the kit) for 3 min. Worm suspension was centrifuged at 7000 rpm for 7 min. 100 μl of reaction mixture which was prepared by adding 200 μl of 20 mM Amplex red solution (prepared by dissolving 1 mg of Amplex red in 200 μl of DMSO), 100 μl of 200 U ml−1 HRP solution (prepared by dissolving contents of one vial of AchE in 600 μl of 1× reaction buffer) and 100 μl of 20 U ml−1 choline oxidase solution (prepared by dissolving content of 1 vial of choline oxidase in 600 μl of 1× reaction buffer) in 10 ml q.s. of 1× reaction buffer in black well plates. The plates were incubated at room temperature for 30 min and fluorescence was read using 96 well plate fluorimeter (BMG Polarstar Galaxy) at excitation 544 nm and emission at 590 nm. The relative fluorescence so obtained was normalized with protein content of sample calculated using Bradford method30 and RFU per μg of protein was calculated to estimate the relative Ach levels.
2.16. Quantitative RT-PCR
Primers for real time were designed online using primer quest tool from the IDT DNA technologies website (http://www.idtdna.com). The amplicon size was kept between 100 and 200 base pairs, GC% was kept above 50% and melting temperature was kept between 58 °C and 62 °C. The specific sequences of the forward and reverse primers are specified in Table 1.
Table 1 The sequence of forward and reverse primers used for quantitative RT-PCR
Primer |
Sequence |
ace-1F |
CTG CTA CCA CGT CAG ATA C |
ace-1R |
TGA GCC AGA GCC ATT TC |
ace-2F |
CTG TAG CTC ACC GGA TAT G |
ace-1R |
GTC GCC CTG GAA GAA AT |
cha-1F |
GGG AAA GGG AAG AAA CGA |
cha-2R |
GGA CCA CTG CAC CAT AC |
unc-17F |
ACC ATC ACA ACC TGG ATG TCC GAA |
unc-17R |
TCC ATA GCC AAC CCA ACC ATA GCA |
unc-29F |
CGA GGA CCA AGA ACT CAT C |
unc-29R |
ACT GTC CAA CTC CTG GTA |
unc-38F |
CGC TGA CAG CAA CTA CA |
unc-38R |
CAG GAG CCG AAC TTC AA |
unc-50F |
CAT CCC AGT CAC CGA TTT |
unc-50R |
TGA GAG CTT GGC GAA TG |
Total RNA was extracted from adult age-synchronized animals using trizol reagent (Invitrogen, Life Technologies) according to the manufacturer's instructions. Briefly worms were washed off treatment plates using 0.1% DEPC water. The worms were crushed in 250 μl trizol reagent using micro pestles. Another 750 μl of trizol reagent was added to make the final volume to 1 ml, followed by addition of 200 μl of chloroform and mixing for 2 to 5 min on a vortex mixer. The suspension was then centrifuged at 14
000 rpm, 4 °C for 15 min and upper aqueous phase was gently pipette out in the fresh vials. RNA was precipitated by addition of 500 μl chilled isopropanol. The vials were kept at room temperature for 10 min and were centrifuged at 14000 rpm, 4 °C for 10 min and RNA pellet, so obtained was washed twice with 75% ethanol (chilled) at 7500 rpm, 4 °C for 5 min. The RNA pellet was finally dissolved in 15 μl of 0.1% DEPC water. To quantify RNA absorbance was read using nano drop (Qua Well Q5000).
cDNA synthesis was done from 1 μg of total C. elegans RNA in a 96 well thermal cycler (BioRad, C1000) with steps including, incubation at 25 °C for 10 min, 37 °C for 120 min, 85 °C for 5 min and 4 °C forever RNA using high capacity cDNA synthesis Kit (Applied Biosystems). cDNA sample were quantified using nanodrop and were stored at −80 °C until use. 125 ng of cDNA was used as template for each reaction of qRT-PCR with gpd-1 as housekeeping control using light cycler 480 machine (Roche Diagnostics, Germany). For each primer pair, a melting curve analysis was performed according to instrument. The program in brief was an initial incubation of 50 °C for 2 min hold (UDG incubation) and 95 °C for 10 min followed by 40 cycles at 95 °C for 15 s (denaturation), 58 °C for 30 s (annealing) and final extension at 72 °C for 20 s. Differential expression was calculated by 2−ΔΔCT method. Gpd-1 was used as internal control and used to normalize ratios between samples.31
2.17. Statistical analysis
All data were presented as mean ± SEM/SD and analyzed by one-way ANOVA followed by Bonferroni's multiple comparison tests for the possible significance identification between the various groups. *p < 0.05, **p < 0.01, ***p < 0.001, were considered as statistically significant. Statistical analysis was performed using Graph Pad Prism software (5.02) (Trial Version), San Diago, CA.
3. Results
3.1. ECG and HRV
The oral administration of PAL to the DMH treated albino wistar rats affected the various parameters of ECG (Fig. 1). Comparing the normal control and toxic control there was no prominent difference in RR interval. Heart rate was increased in toxic control group (378.1 ± 29.96) which was similarly found in higher dose (0.5 mg kg−1) of drug and the same was decreased by lower dose (303.74 ± 84.74) of PAL treatment. P duration of normal control (0.01 ± 0.002) and toxic control (0.01 ± 0.001) groups as well as higher dose (0.01 ± 0.003) of treatment were comparatively similar but were significantly increased in lower dose (0.02 ± 0.003) of the PAL. QRS complex of all the groups were same (0.01 ± 0.001) with slight increase (0.02 ± 0.004) in toxic control group. The lower frequency (LF) was adversely decreased in toxic control (0.21 ± 0.17) group and was increased in the treatment groups. Similar pattern of changes were evident with higher frequency variables (HF) (Tables 2 and 3).
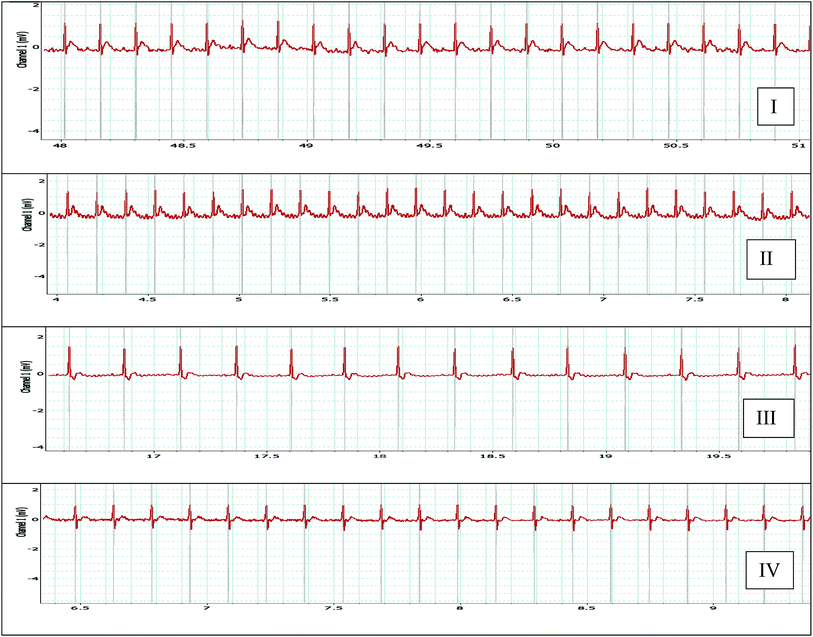 |
| Fig. 1 Representative ECG recordings of the control, DMH and PAL treated animals. (I) Control (1 mM EDTA + saline, 2 ml kg−1, s.c.); (II) toxic control (DMH, 20 mg kg−1, s.c.); (III) PAL + DMH (0.25 mg kg−1, p.o. + 20 mg kg−1, s.c.); (IV) PAL + DMH (0.5 mg kg−1, p.o. + 20 mg kg−1, s.c.). | |
Table 2 Effect of PAL on ECG changes in DMH treated animalsa
ECG parameters |
Control (1 mM EDTA + saline, 2 ml kg−1, s.c.) |
Toxic control (DMH, 20 mg kg−1, s.c.) |
PAL + DMH (0.25 mg kg−1, p.o. + 20 mg kg−1, s.c.) |
PAL + DMH (0.5 mg kg−1, p.o. + 20 mg kg−1, s.c.) |
(Values are presented as mean ± SEM), each group contains 8 animals. Comparisons were made on the basis of the one-way ANOVA followed by Bonferroni multiple test. All groups were compared to the toxic control group (*p < 0.05, **p < 0.01, ***p < 0.001). |
RR interval (s) |
0.20 ± 0.02 |
0.20 ± 0.01 |
0.21 ± 0.10 |
0.20 ± 0.01 |
Heart rate (BPM) |
334.84 ± 49.65 |
378.1 ± 29.96 |
303.74 ± 84.74*** |
378.4 ± 19.20 |
PR interval (s) |
0.04 ± 0.003 |
0.04 ± 0.01 |
0.04 ± 0.01 |
0.04 ± 0.004 |
P duration (s) |
0.01 ± 0.002 |
0.01 ± 0.001 |
0.02 ± 0.003*** |
0.01 ± 0.003*** |
QRS interval (s) |
0.01 ± 0.001*** |
0.02 ± 0.004 |
0.01 ± 0.002*** |
0.01 ± 0.003*** |
QT interval (s) |
0.08 ± 0.01 |
0.07 ± 0.02 |
0.05 ± 0.01 |
0.06 ± 0.01 |
QTc (s) |
0.18 ± 0.01 |
0.16 ± 0.05 |
0.10 ± 0.04* |
0.17 ± 0.03 |
JT interval (s) |
0.06 ± 0.01 |
0.05 ± 0.02 |
0.03 ± 0.01* |
0.04 ± 0.01 |
T peak T end interval (s) |
0.03 ± 0.01 |
0.03 ± 0.02 |
0.02 ± 0.01 |
0.02 ± 0.01 |
P amplitude (mV) |
0.09 ± 0.05 |
0.03 ± 0.01 |
0.06 ± 0.01** |
0.02 ± 0.01 |
Q amplitude (mV) |
0.03 ± 0.02 |
−0.25 ± 0.57 |
−0.01 ± 0.03 |
0.02 ± 0.004 |
R amplitude (mV) |
1.74 ± 0.52 |
1.31 ± 0.64 |
1.05 ± 0.33** |
0.86 ± 0.10*** |
S amplitude (mV) |
−0.15 ± 0.17 |
−0.17 ± 0.27 |
−0.17 ± 0.21 |
−0.6 ± 0.10 |
ST height (mV) |
0.13 ± 0.09* |
−0.02 ± 0.09 |
−0.04 ± 0.09 |
−0.03 ± 0.01 |
T amplitude (mV) |
0.38 ± 0.19 |
0.10 ± 0.33 |
−0.003 ± 0.22*** |
0.20 ± 0.02*** |
Table 3 Effect of PAL on HRV against DMH induced colon carcinogenesisa
HRV |
Control (1 mM EDTA + saline, 2 ml kg−1, s.c.) |
Toxic control (DMH, 20 mg kg−1, s.c.) |
PAL + DMH (0.25 mg kg−1, p.o. + 20 mg kg−1, s.c.) |
PAL + DMH (0.5 mg kg−1, p.o. + 20 mg kg−1, s.c.) |
(Values are presented as mean ± SEM), each group contains 8 animals. Comparisons were made on the basis of the one-way ANOVA followed by Bonferroni multiple test. All groups were compared to the toxic control group (*p < 0.05, **p < 0.01, ***p < 0.001). |
Time domain |
Average RR (ms) |
183.91 ± 31.38 |
159.76 ± 13.92 |
218.7 ± 90.92*** |
169.00 ± 2.00 |
Median RR (ms) |
183.00 ± 28.78 |
159.4 ± 13.68 |
218.57 ± 89.85*** |
170.00 ± 1.00 |
SDRR (ms) |
9.99 ± 12.58 |
19.97 ± 3.03 |
13.25 ± 1.87** |
6.00 ± 6.10*** |
SDARR (ms) |
6.22 ± 6.03** |
2.38 ± 2.98 |
6.15 ± 4.93** |
3.80 ± 4.30 |
CVRR |
0.05 ± 0.04** |
0.02 ± 0.01 |
0.05 ± 0.03*** |
0.03 ± 0.03 |
![[thin space (1/6-em)]](https://www.rsc.org/images/entities/char_2009.gif) |
Frequency domain |
LF (μs2) |
17.38 ± 44.19*** |
0.21 ± 0.17 |
18.99 ± 1.09*** |
11.7 ± 23.10*** |
HF (μs2) |
67.84 ± 17.16*** |
1.05 ± 0.01 |
49.99 ± 10.40*** |
73.7 ± 14.9*** |
LF/HF (μs2) |
0.29 ± 0.07 |
0.23 ± 0.08 |
0.37 ± 0.03 |
0.20 ± 0.10 |
3.2. pH, total acidity, percentage weight variation and ACF count
The gastric pH of toxic control group (6.9 ± 0.28) was slightly decreased from normal control rats (7.2 ± 0.43). Total acidity (mEq l−1) was very high in toxic control group (136.76 ± 6.41) from normal (109.68 ± 9.52). Similarly it was higher (111.4 ± 10.43) in low dose but lowest (73.58 ± 9.60) in higher dose of the treatment. The ACF count was found to be more in toxic control group (89.3 ± 2.00) as compared to normal control group (7.3 ± 4.0) and found to be significantly decreased in PAL treated rats. There was positive percentage weight variation found among all the groups, normal control (21.0 ± 10.4), low dose PAL (12.2 ± 0.3), high dose PAL (22.4 ± 0.3) except toxic control (−8.0 ± 0.3) (Table 4).
Table 4 Effect of PAL on pH, total acidity and ACF formation against DMH induced colon carcinogenesisa
Parameters |
Control (1 mM EDTA + saline, 2 ml kg−1, s.c.) |
Toxic control (DMH, 20 mg kg−1, s.c.) |
PAL + DMH (0.25 mg kg−1, p.o. + 20 mg kg−1, s.c.) |
PAL + DMH (0.5 mg kg−1, p.o. + 20 mg kg−1, s.c.) |
(Values are mean ± SEM), each group contains 8 animals. Comparisons were made on the basis of the one-way ANOVA followed by Bonferroni test. All groups were compared to the toxic group (*p < 0.05, **p < 0.01, ***p < 0.001). |
Weight variation (%) |
21.0 ± 1.40*** |
−8.0 ± 2.30 |
12.2 ± 0.30*** |
22.4 ± 0.30*** |
pH |
7.2 ± 0.43 |
6.9 ± 0.28 |
7.6 ± 0.53* |
7.4 ± 0.23 |
Total acidity (mEq l−1) |
109.68 ± 9.52 |
136.76 ± 6.41 |
111.4 ± 10.43* |
73.58 ± 9.60*** |
Aberrant crypts (NoS) |
7.30 ± 4.00*** |
89.30 ± 2.0 |
39.30 ± 9.20** |
19.00 ± 9.20*** |
3.3. Antioxidant assay
DMH made evident upsurge in MDA level (4.7 ± 0.2) in comparison to normal control rats. Higher dose of PAL significantly (<0.001) decrease the MDA level (1.4 ± 0.2). AchEs levels (0.05 ± 0.002) were also increased in DMH treated rats. Furthermore, treatment with PAL (0.5 mg kg−1) decreased the AchEs levels non-significantly. Similarly, decrease in SOD activity was observed in the toxic control group (1.62 ± 0.04) in counterpart to the normal control (1.80 ± 0.02). Down regulated GSH after DMH treatment (0.28 ± 0.02) was observed and this was significantly increased after the PAL treatment at low dose (Table 5).
Table 5 Effect of PAL on oxidative stress markers against DMH induced colon carcinogenesisa
Parameters |
Control (1 mM EDTA + saline, 2 ml kg−1, s.c.) |
Toxic control (DMH, 20 mg kg−1, s.c.) |
PAL + DMH (0.25 mg kg−1, p.o. + 20 mg kg−1, s.c.) |
PAL + DMH (0.5 mg kg−1, p.o. + 20 mg kg−1, s.c.) |
(Values are mean ± SEM), each group contains 8 animals. Comparisons were made on the basis of the one-way ANOVA followed by Bonferroni test. All groups were compared to the toxic group (*p < 0.05, **p < 0.01, ***p < 0.001). |
TBARS (nM of MDA per μg of protein) |
2.50 ± 0.20** |
4.7 ± 0.20 |
2.9 ± 0.20** |
1.4 ± 0.10*** |
GSH × 10−4 (μg%) |
0.33 ± 0.01*** |
0.28 ± 0.02 |
0.46 ± 0.02*** |
0.37 ± 0.01* |
SOD (unit of SOD per μg of protein) |
1.80 ± 0.02** |
1.62 ± 0.04 |
1.70 ± 0.05 |
1.83 ± 0.03** |
Catalase (nM of H2O2 disappeared per min per μg of protein) |
0.60 ± 0.01*** |
0.40 ± 0.03 |
0.80 ± 0.10*** |
0.60 ± 0.10*** |
AchE (nM per min per ml of unit) |
0.02 ± 0.01*** |
0.05 ± 0.002 |
0.02 ± 0.001*** |
0.01 ± 0.002*** |
3.4. COX and LOX levels
Up regulated COX activity was observed in the DMH treated animals which was subsided by the PAL treatment significantly (Fig. 2A). LOX expression in toxic control group was decreased from normal control. It was over expressed in lower dose of treatment (Fig. 2B).
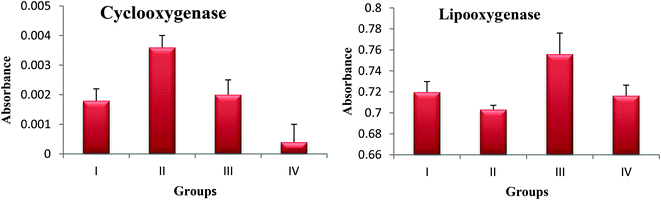 |
| Fig. 2 Effect of PAL on inflammatory markers against DMH induced colon carcinogenesis. (Values are mean ± SEM), each group contains 8 animals. Comparisons were made on the basis of the one-way ANOVA followed by Bonferroni test. All groups were compared to the toxic group (*p < 0.05, **p < 0.01, ***p < 0.001); (I) control (1 mM EDTA + saline, 2 ml kg−1, s.c.); (II) toxic control (DMH, 20 mg kg−1, s.c.); (III) PAL + DMH (0.25 mg kg−1, p.o. + 20 mg kg−1, s.c.); (IV) PAL + DMH (0.5 mg kg−1, p.o. + 20 mg kg−1, s.c.). | |
3.5. Morphological and histopathological evaluation
ACF were evident in the colonic mucosa of the DMH treated animals when studied morphologically at 500× (Fig. 3F). Small neoplastic lesions were also observed in the colonic tissues of the DMH treated animals when viewed at 2000× (Fig. 3J). Histopathological evaluation revealed normal crypts, globet cells along with lamina propria (Fig. 3M). Treatment with DMH revealed loss of crypts, globet cells and distorted lamina propria (Fig. 3N). PAL treatment helped to restore the normal architecture of colonic mucosa (Fig. 3O and P).
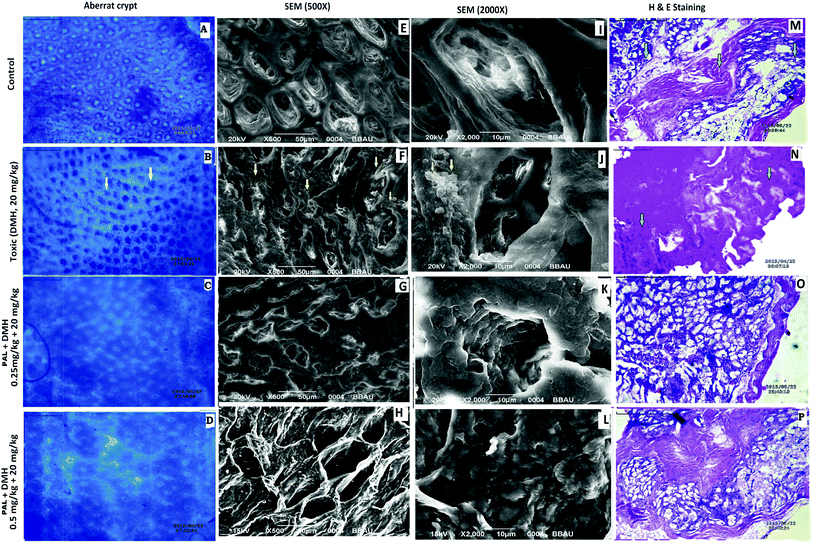 |
| Fig. 3 Methylene blue staining, scanning electron microscopy and histopathological evaluation of the colonic tissue. (A–D) Methylene blue staining of the colonic tissue. (A) Control; (B) toxic control; (C) PAL (0.25 mg kg−1); (D) PAL (0.5 mg kg−1) [ denotes the abberant crypts in the toxic control group]. (E–H) Scanning electron microscopy of colonic tissue (500×). (E) Control; (F) toxic control; (G) PAL (0.25 mg kg−1); (H) PAL (0.5 mg kg−1) [ denotes the abberant crypts in the toxic control group]. (I–L) Scanning electron microscopy of the colon tissue (2000×). (I) Control; (J) toxic control; (K) PAL (0.25 mg kg−1); (L) PAL (0.5 mg kg−1) [ depicts the small neoplastic lesion on the mucosal tissue]. (M–P) Histopathological evaluation of the colonic tissue with H & E staining. (M) Control; (N) toxic control; (O) PAL (0.25 mg kg−1); (P) PAL (0.5 mg kg−1) [ depicts various layers of the mucosal tissue]. | |
3.6. Aldicarb assay
In order to justify our hypothesis regarding increased neuronal stimulation mediated anti-inflammatory effect mitigating colon cancer progression we directed our study to define the effect of PAL on Ach function using a simple model organism, C. elegans, for which we employed aldicarb assay, an indirect assay to determine the relative effect on synaptic Ach levels. As a convention greater number of worms paralyzed at a given time point indicates elevated synaptic Ach levels and vice versa. In comparison to the percentage of worms paralyzed in control (44.32 ± 2.0) we observed a significant increase in percentage of worms paralyzed upon treatment with 4 μM PAL (71.66 ± 1.66, p value = 0.008) and 8 μM PAL (85.62 ± 4.37, p value = 0.002). However no effect on percentage of worms paralyzed was observed in worms treated with 4 μM PAL (45.83 ± 0.67, p value = 0.980). The results implicate enhanced cholinergic neurotransmission as a result of PAL treatment (Fig. 4A).
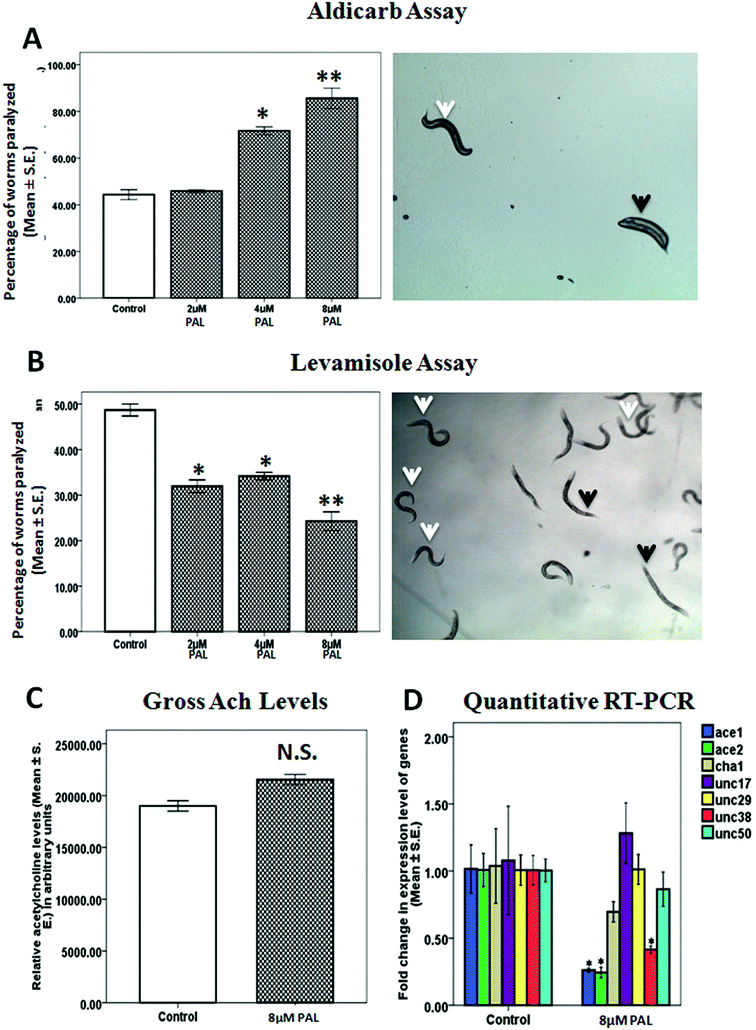 |
| Fig. 4 Effect of PAL on various stages of cholinergic neurotransmission in C. elegans. Paralyzed and unparalysed worms are indicated by black and white arrows respectively. PAL treatment resulted in increased in synaptic Ach levels in a dose dependent manner (A); PAL treatment resulted in reduced sensitivity of nicotinic acetylcholine receptor (B); treatment with 8 μM PAL did not alter gross acetylcholine levels (C); treatment with 8 μM PAL resulted in down-regulation of ace-1, ace-2 and unc-38 (D). | |
3.7. Levamisole assay
After assessment of the synaptic Ach levels, we tried to ascertain the involvement of nAchR by employing levamisole assay. Similar to the aldicarb assay the greater number of worms paralyzed in response to levamisole indicates higher activity of nAchR. In comparison to the percentage of worms paralyzed in control (48.68 ± 1.31), we observed decreased in percentage of worms paralyzed upon treatment with 2 μM PAL (31.94 ± 1.38, p value = 0.0006) and 4 μM (34.16 ± 0.83, p value = 0.10) and 8 μM (24.26 ± 2.04, p value = 0.001). The results implicate dose dependent repressive effect of PAL on nAchR (Fig. 4B).
3.8. Gross Ach levels
We studied the gross levels of Ach in worms subjected to treatment with 8 μM PAL, where we observed that in comparison to control (19
001.70 ± 511.68), there was a marginal increase in Ach levels in worms treated with 8 μM PAL (21
554.39 ± 500.73, p value = 0.070), however the alteration was statistically insignificant (Fig. 4C).
3.9. Quantitative RT-PCR
After ascertaining that PAL treatment was leading to elevated cholinergic transmission, our next objective was to identify the genomic contributors for the observed phenotype, which was achieved through quantitative PCR for genes ace-1, ace-2, cha-1, unc-17, unc-29, unc-38 and unc-50. In comparison to the control we observed a significant down regulated expression of gene ace-1 (0.26 ± 0.01, p value = 0.052), ace-2 (0.24 ± 0.03, p value = 0.028) and unc-38 (0.41 ± 0.02, p value = 0.035). The fold change in relative expression of rest of the genes i.e. cha-1, unc-17, unc-29 and unc-50 was however close to normal (Fig. 4D).
4. Discussions
The present study reveals the effect of PAL on DMH induced carcinogenesis in albino wistar rats. Deteriorated electrocardiographic changes and poor HRV has been associated with cancer patients.32 In fact direct coordination has been derived between HRV and cancer prognosis. Lower HRV is a measure of autonomic dysfunction in advanced cancer and higher HRV has been associated with lower levels of tumor markers.33,34 Similar pattern of diminished HRV in the time and frequency domain was recorded in the DMH treated animals. Treatment with PAL demonstrated significant restoration of time and frequency domain paradigms towards normal. Results suggest restoration of the autonomic function subsequent to PAL treatment.
Carcinogenesis is a multistep process and oxygen radicals promote all stages of cancer progression.35 Association between serious oxidative stress and colon cancer progression is a well studied phenomenon whereby, previous studies have reported increased levels of lipid peroxide formation, and SOD along with decrease in catalase and reduced GSH in clinical IV stage of colon cancer.36 In the present study, we perceived increase in lipid peroxide formation along with decrease in CAT and reduced GSH levels in colonic tissue of DMH treated animals. In contravention to the previous reports, we observed decrease in the levels of SOD. It would be appropriate to mention that both CAT and SOD work in tandem and constitute a major supportive team of defense against free radicals. SOD scavenges superoxide radical to form H2O2 and molecular oxygen. H2O2 is subsequently scavenged by catalase and protects the tissue from highly reactive hydroxyl radical.20 Therefore, one can perceive similar pattern of changes for catalase and SOD and same was evident in the present experiment. The decreased levels of SOD, catalase and GSH were recorded after the DMH treatment, which could be attributed to their increased utilization during the course of oxidative stress. Similar pattern of changes has been reported by us during the course of oxidative stress.18,20 All in all, PAL helped to restore the biochemical markers of oxidative stress towards normal. Prima facie, one can commensurate that PAL can positively modulate the deleterious effects of DMH in experimental animals.
Inflammation plays a crucial role in carcinogenesis. COX and LOX constitute a major team of enzymes participating in the inflammatory cascade. COX in particular COX-2 has been shown to be over expressed in epithelial cancer, including colon cancer.37,38 In the same line, we observed increased levels of COX in the DMH treated animals and PAL helped to downregulate the increased levels of COX. Increased inflammation leads to epithelial cell degeneration and inappropriate proliferation leading to mild cryptal architectural abnormalities called as aberrant crypts eventually forming invasive cancers.39 These histopathological characteristics are considered as paramount for the early detection and prevention of cancer.40 Increased ACF was evident in the DMH treated animals when scrutinized using methylene blue staining.
Morphological features of classical elevated ACF (500×) and small flat neoplastic lesions (2000×) in the colon tissue of DMH treated rats as observed by surface examination under scanning electron microscope. Treatment with PAL demonstrated decrease in ACF, restored crypts with normal mucosa. The normal histology of colonic tissue describes three layers comprising of mucosa, submucosa and muscularis. Intact colonic architecture was recorded in the normal control group demonstrating all three reported layers but toxic control group shows interference of layers and arrangement of cells in the colonic tissue. The lower treatment group shows the tissue approximately similar to normal control group. The dose independent effect was also found here in the treatment with PAL.
Authors would to like summarize the results from the in vivo studies, that PAL has significant effect on the progression of colon carcinogenesis through positively modulating the physiological, oxidative stress and inflammatory markers. The positive prognosis by the PAL was also evident through hemodynamic changes as observed. All in all PAL demonstrated a significant effect against the DMH induced preneoplastic colon damage and the same is hypothesized to be mediated through cholinergic anti-inflammatory signaling mediated by the α-7nAchR partial agonistic activity of PAL or by down regulating the AchEs, as observed in the current experiment.
It would be appropriate to mention that the mechanism of neurotransmission in C. elegans and mammalian models is highly conserved with AchE inhibitors showing potent activity in both the models. Moreover, C. elegans has already been demonstrated as an efficient model to study the biology of the synapse and significant set of studies exist where the findings from worms models are sufficiently replicated and corroborated the findings obtained from rodent models.28,41 Considering the importance of C. elegans as a biological tool to study synaptic transmission and in order to justify our hypothesis, we proceeded with elucidation of molecular events linked with cholinergic neurotransmission.
In order to determine the effect of PAL treatment on cholinergic transmission and for selection of an effective dose, we studied the effect of PAL at three different doses using aldicarb assay. Aldicarb is an AchEs inhibitor and results in buildup of synaptic Ach culminating paralysis in worms. The percentage of worms paralyzed at a given time point is proportional to the levels of Ach in the synapse. We observed the 8 μM dose to be the most potent in enhancing cholinergic neurotransmission. The beneficial effects on cholinergic transmission pointed towards possible linkage of elevated Ach levels and cancer regression through cholinergic anti inflammatory pathway. We further addressed the possible role of nAchR in this context by determining the effect of PAL treatment (2 μM, 4 μM and 8 μM) through levamisole assay. We observed that the chronic PAL treatment was negatively modulating the activity of nAchR in a dose dependent manner. 8 μM PAL being the optimum dose culminating elevated cholinergic transmission was selected for detailed studies. The next question arises that, if enhanced neurotransmission was due to increased synthesis of Ach or some other mechanisms. To answer this we performed the estimation of gross Ach levels, where we observed that there was some increase in gross Ach levels in PAL treated worms but the change was statistically insignificant. To further elucidate the possible mechanism we performed qPCR studies for the genes involved in Ach synthesis, transport, degradation and receptor. We observed a significant downregulation in genes ace-1, ace-2 and unc-38. Notably genes ace-1, ace-2 and unc-38 codes AchEs42 and α-7 subunit of nAchR42 respectively. Our findings from the qPCR studies clearly corroborated the findings about synaptic Ach levels and studies on AchR. The increased synaptic Ach levels as evident through aldicarb assay can be attributed to the decreased levels of AchE, the major enzyme responsible for degradation of Ach in the synapse which was evident from decreased genomic levels of ace-1 and ace-2. These findings were also supported by the fact that we observed decreased AchE activity in PAL treated rats, as shown in Table 5. In addition the down regulation in expression of unc-38 (one of the necessary components of nAchR43) sufficiently links with the decreased nAchR activity. The other genes viz. cha-1 (coding for choline acetyltransferase), unc-17 (coding for acetylcholine transporter), unc-29 (coding for non α subunit of nAchR) and unc-50 (regulator of nAchR trafficking) were devoid of any significant alteration.
These findings refuted our original hypothesis that PAL can mediate cholinergic antiinflammatory pathway through its partial agonistic action towards α-7nAchR. Rather the results indicated that continuous PAL treatment results in reduced activity of nAchR (mediated through down regulation of unc-38); however there was a gross increase in cholinergic transmission (accounting for modulation of cholinergic anti-inflammatory pathway); mediated through down regulation of genes coding for AchEs, as depicted in the scheme in Fig. 5. The downregulation in the AchEs as observed in the C. elegans was also evident in the rat model and therefore represents the replicability and connectivity of results obtained using two different model organisms encouraging researchers to utilize diverse model organisms connected with similar genes.
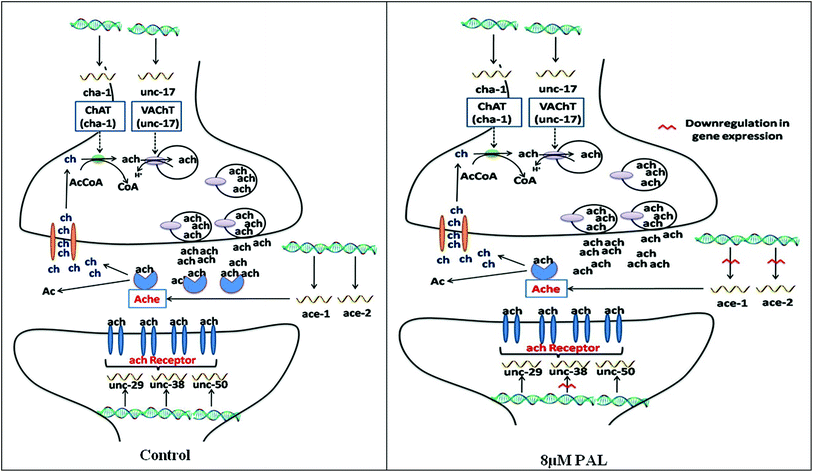 |
| Fig. 5 Schematic representation of Ach vascular and synaptic neurotransmission and its modulation by PAL. Scheme depicting the molecular events in modulation of neurotransmission upon treatment with 8 μM PAL: PAL treatment resulted in downregulation of unc-38 and lowered activity of nAchR. However subsequent downregulation of ace-1 and ace-2 resulted in lowered activity of AchE which led to significant elevation of synaptic Ach levels as was observed through aldicarb assay. | |
Authors would like to conclude that treatment with PAL can positively modulate the preneoplastic colon damage through the up regulating the cholinergic anti-inflammatory pathway mediated by downregulation of the genes coding for AchEs and thereby inhibiting Ach degradation. All in all, our findings emphasize the potential role of PAL in suppression of colon carcinogenesis and opens future endeavors of research towards exploring the AchE inhibiting potential of PAL.
Declaration
The submission complies with the Helsinki Declaration with no information that has been published elsewhere. There are also no diagnostic testing and copyrighted material. The authors declare no potential conflicts of interest with respect to the authorship and/or publication of this article.
Author's contribution
RKM, JKR and RKY performed the experimental work on albino rats; SRS performed the experimental work on C. elegans; SR, MS, UD and SG performed the electrocardiographic studies, SEM, histopathological studies; MNA and ASS performed the statistical studies and compiled the data; RP designed the experiment on C. elegans; GK and SAS perceived the idea, designed and supervised the overall study. Performed the revision. Prepared and proof read the final manuscript.
References
- G. Matteoli and G. E. Boeckxstaens, Gut, 2013, 62, 1214–1222 CrossRef CAS PubMed.
- L. V. Borovikova, S. Ivanova, D. Nardi, M. Zhang, H. Yang, M. Ombrellino and K. J. Tracey, Auton. Neurosci., 2000, 85, 141–147 CrossRef CAS PubMed.
- G. Vida, G. Peña, E. A. Deitch and L. Ulloa, J. Immunol., 2011, 186, 4340–4346 CrossRef CAS PubMed.
- J. A. Martiney, A. J. Rajan, P. C. Charles, A. Cerami, P. C. Ulrich, S. Macphail, K. J. Tracey and C. F. Brosnan, J. Immunol., 1998, 160, 5588–5595 CAS.
- K. Åkerlund, H. E. Harris, K. Tracey, H. Wang, T. Fehniger, L. Klareskog, J. Andersson and U. Andersson, Clin. Exp. Immunol., 1999, 115, 32 CrossRef.
- V. A. Pavlov, H. Wang, C. J. Czura, S. G. Friedman and K. J. Tracey, Mol. Med., 2003, 9, 125 CAS.
- J. Meregnani, D. Clarençon, M. Vivier, A. Peinnequin, C. Mouret, V. Sinniger, C. Picq, A. Job, F. Canini and M. Jacquier-Sarlin, Auton. Neurosci., 2011, 160, 82–89 CrossRef CAS PubMed.
- F. The, C. Cailotto, J. Van Der Vliet, W. De Jonge, R. Bennink, R. Buijs and G. Boeckxstaens, Br. J. Pharmacol., 2011, 163, 1007–1016 CrossRef CAS PubMed.
- A. H. Milby, C. H. Halpern and G. H. Baltuch, Neurotherapeutics, 2008, 5, 75–85 CrossRef PubMed.
- H. C. Reinhardt and B. Schumacher, Trends Genet., 2012, 28, 128–136 CrossRef CAS PubMed.
- L. M. Coussens and Z. Werb, Nature, 2002, 420, 860–867 CrossRef CAS PubMed.
- T. Shintaku, T. Ohba, H. Niwa, T. Kushikata, K. Hirota, K. Ono, Y. Matsuzaki, T. Imaizumi, K. Kuwasako and D. Sawamura, J. Pharmacol. Sci., 2014, 126, 351–358 CrossRef CAS PubMed.
- K. Shukla, P. Raj, A. Kumar, M. Kumar and G. Kaithwas, Sci. World J., 2014, 2014 Search PubMed.
- P. Khinchi, S. Saha, S. A. Saraf and G. Kaithwas, Pharmacol. Rep., 2014, 66, 165–168 CrossRef CAS PubMed.
- R. P. Bird, Cancer Lett., 1987, 37, 147–151 CrossRef CAS PubMed.
- M. Ochiai, Y. Hippo, M. Izumiya, M. Watanabe and H. Nakagama, Cancer Sci., 2014, 105, 943–950 CrossRef CAS PubMed.
- S. Kumar, M. Singh, J. K. Rawat, S. Gautam, S. A. Saraf and G. Kaithwas, Toxicol. Mech. Methods, 2014, 24, 666–671 CrossRef CAS PubMed.
- P. Raj, M. Singh, J. K. Rawat, S. Gautam, S. A. Saraf and G. Kaithwas, RSC Adv., 2014, 4, 60397–60403 RSC.
- N. Renu, G. Kaithwas, P. Ramteke and S. Saraf, Acta Gastro-Enterol. Belg., 2012, 75, 331–335 CAS.
- G. Kaithwas and D. K. Majumdar, Eur. J. Lipid Sci. Technol., 2012, 114, 1237–1245 CrossRef CAS.
- S. K. Gupta, S. Gautam, J. K. Rawat, M. Singh, S. A. Saraf and G. Kaithwas, RSC Adv., 2015, 5, 9354–9360 RSC.
- G. L. Ellman, K. D. Courtney, V. Andres and R. M. Featherstone, Biochem. Pharmacol., 1961, 7, 88–95 CrossRef CAS PubMed.
- D. Riendeau, M. Percival, C. Brideau, S. Charleson, D. Dube, D. Ethier, J.-P. Falgueyret, R. Friesen, R. Gordon and G. Greig, J. Pharmacol. Exp. Ther., 2001, 296, 558–566 CAS.
- L. Cullen, L. Kelly, S. O. Connor and D. J. Fitzgerald, J. Pharmacol. Exp. Ther., 1998, 287, 578–582 CAS.
- W. Lu, X. Zhao, Z. Xu, N. Dong, S. Zou, X. Shen and J. Huang, Anal. Biochem., 2013, 441, 162–168 CrossRef CAS PubMed.
- T. Cooke, N. Kirkham, D. Stainthorp, C. Inman, N. Goeting and I. Taylor, Gut, 1984, 25, 748–755 CrossRef CAS PubMed.
- B. Belur, N. Kandaswamy and K. Mukherjee, Med. Lab. Technol., 1990, 1124–1188 Search PubMed.
- T. R. Mahoney, S. Luo and M. L. Nonet, Nat. Protoc., 2006, 1, 1772–1777 CrossRef CAS PubMed.
- H. Qian, A. P. Robertson, J. A. Powell-Coffman and R. J. Martin, FASEB J., 2008, 22, 3247–3254 CrossRef CAS PubMed.
- M. M. Bradford, Anal. Biochem., 1976, 72, 248–254 CrossRef CAS PubMed.
- A. Giulietti, L. Overbergh, D. Valckx, B. Decallonne, R. Bouillon and C. Mathieu, Methods, 2001, 25, 386–401 CrossRef CAS PubMed.
- Y. Guo, S. Koshy, D. Hui, J. L. Palmer, K. Shin, M. Bozkurt and S. W. Yusuf, J. Clin. Neurophysiol., 2015, 32, 516–520 CrossRef PubMed.
- C. Mouton, A. Ronson, D. Razavi, F. Delhaye, N. Kupper, M. Paesmans, M. Moreau, J.-M. Nogaret, A. Hendlisz and Y. Gidron, Auton. Neurosci., 2012, 166, 96–99 CrossRef CAS PubMed.
- Y. Guo, J. L. Palmer, F. Strasser, S. W. Yusuf and E. Bruera, Eur. J. Canc. Care, 2013, 22, 612–616 CrossRef CAS PubMed.
- E. Skrzydlewska, A. Stankiewicz, M. Sulkowska, S. Sulkowski and I. Kasacka, J. Toxicol. Environ. Health, Part A, 2001, 64, 213–222 CrossRef CAS PubMed.
- E. Skrzydlewska, S. Sulkowski, M. Koda, B. Zalewski, L. Kanczuga-Koda and M. Sulkowska, World J. Gastroenterol., 2005, 11, 403–406 CrossRef CAS PubMed.
- F. A. Sinicrope and S. Gill, Cancer Metastasis Rev., 2004, 23, 63–75 CrossRef CAS PubMed.
- N. Kawai, M. Tsujii and S. Tsuji, Prostaglandins Other Lipid Mediators, 2002, 68, 187–196 CrossRef PubMed.
- S. Khare, K. Chaudhary, M. Bissonnette and R. Carroll, in Cancer Epidemiology, Springer, 2009, pp. 373–386 Search PubMed.
- M. J. Wargovich, V. R. Brown and J. Morris, Cancers, 2010, 2, 1705–1716 CrossRef CAS PubMed.
- S. S. Chaudhaery, K. K. Roy, N. Shakya, G. Saxena, S. R. Sammi, A. Nazir, C. Nath and A. K. Saxena, J. Med. Chem., 2010, 53(17), 6490–6505 CrossRef CAS PubMed.
- D. Combes, Y. Fedon, J.-P. Toutant and M. Arpagaus, Int. Rev. Cytol., 2001, 209, 207–239 CAS.
- J. T. Fleming, M. D. Squire, T. M. Barnes, C. Tornoe, K. Matsuda, J. Ahnn, A. Fire, J. E. Sulston, E. A. Barnard and D. B. Sattelle, J. Neurosci., 1997, 17, 5843–5857 CAS.
Footnote |
† Both authors have contributed equally. |
|
This journal is © The Royal Society of Chemistry 2016 |
Click here to see how this site uses Cookies. View our privacy policy here.