DOI:
10.1039/C6RA06858H
(Paper)
RSC Adv., 2016,
6, 54938-54948
Non-carbonyl Curcuma longa [NCCL] protects the heart from myocardial ischemia/reperfusion injury by reducing endothelial microparticle mediated inflammation in rats†
Received
15th March 2016
, Accepted 24th May 2016
First published on 2nd June 2016
Abstract
Endothelial cell mediated inflammation flags and mediates the progression of pre and post myocardial infarction. The present study evaluated the effect of NCCL (a fraction of curcuma oil) in MI/RP injury. Using myocardial left coronary artery ligation and the reperfusion model, here, we show that NCCL-100 mg kg−1 possessed a better salutary effect than its parent fraction at a lower dose. Pre and post NCCL treatment in MI/RP induced rats resulted in reduced serum-CKMB and circulating levels of TNF-α, IFN-γ, IL-6 and plasma endothelial microparticle levels and improved endothelial functionality as assessed by vasoreactivity. Mechanistically, NCCL reduced the release of inflammatory mediators and endothelial microparticles from the inflamed endothelium both in vitro (10 μg mL−1) and in vivo, it also inhibited the interaction between THP-1 monocytes and endothelial cells resulting in reduced inflammation post MI/RP. Overall, our results conclude that NCCL shows a significant reduction in MI/RP rats by improving the endothelial functionality. NCCL reduced endothelial cell mediated inflammation by reducing endothelial microparticle generation.
1. Introduction
Endothelial dysfunction is the primary mediator of inflammation both in post and pre myocardial stress.1,2 Myocardial ischemia/reperfusion (MI/RP) injury is a well characterized inflammatory condition which results in endothelial cell injury, neutrophil and monocyte accumulation culminating in full-fledged inflammation, all these events lead towards tissue scaring and heart wall degradation.3–5 Endothelial cells have been endowed with vasomodulatory effects in several disease conditions. In MI/RP the endothelial function becomes disturbed aggravating the disease pathophysiology.6 Excessive endothelial inflammation is known to generate endothelial microparticles (EMPs). Clinically, high levels of microparticles have been detected in the plasma of MI patients aggravating MI/RP.7–9 Despite, inflammation being a primary defense mechanism, needs to be tightly regulated in pathological conditions to contain post ischemic complications and heart attack.
Non-carbonyl fraction (NCCL) of Curcuma longa is a novel chemically modified bioactive fraction derived from curcuma oil (C. oil).10 C. oil fraction of Curcuma longa has been previously reported as a remedy for various pathological ailments including stroke,11 cerebral12 and myocardial ischemia reperfusion injury.13 This parent compound was further processed for its new fraction named NCCL, is a mixture non-carbonyl compounds such as zingiberine, curcumene, β-bisabolene, β-sesquiphellandrene, curzerene, curcumenol, iso-curcumenol, germacrene D, gamma-elemene, furanodiene etc. (Indian Patent application filed number 1940/DEL/2014). The NCCL fraction comprises of potent active molecules that gives the efficacy for curcuma oil.
The parent compound C. oil has conferred protection against MI/RP injury in the rat model.13 We hypothesized that NCCL could have even more beneficial effect on MI/RP injury in rats and might reduce the MI/RP induced stress on endothelial cells. In support of this concept, we observed that pre and post treatment with NCCL reduced the infarct size, plasma EMP level and down regulation of adhesion molecules. Further, NCCL treatment significantly decreased the adhesion of THP-1 cells to endothelial cells and rescued the TNF-α mediated endothelial microparticle generation.
2. Materials and methods
2.1 Animals and reagents
Male Wistar rats with initial body weight 270–320 g were obtained from the National Laboratory Animal Centre of CSIR-Central Drug Research Institute (CDRI), Lucknow, India were housed under proper conditions with free access to standard diet and water ad libitum. EA.hy926 endothelial cells generated by C. J. S. Edgell (was generously provided as a gift from the University of North Carolina, Lineberger Comprehensive Cancer Centre) and human THP-1 cells from acute monocytic leukemia patients were cultured in DMEM and RPMI-1640 (Himedia, India) respectively supplemented with 10% FBS and 1% penicillin/streptomycin. All the animal experimental procedures were approved and performed according to the guidelines given by the Institutional Animal Ethical Committee (Approval no. IAEC/2012/60), Council of Scientific and Industrial Research-Central Drug Research Institute (CSIR-CDRI), Lucknow, India. All the efforts were taken during surgical procedures for minimizing the animal suffering using proper anesthesia.
2.2 Preparation of NCCL extract
5 g of hexane soluble fraction of Curcuma longa called curcuma oil was taken in a round bottomed flask with semicarbazide hydrochloride (10 g) and ethyl alcohol (100 mL, 99% pure), few drops of glacial acetic acid were added and refluxed for 48 hours. Further addition of semicarbazide hydrochloride (10 g) at an interval of 5 to 6 hours was continued till the total amount of semicarbazide hydrochloride came up to 50 g. The completion of reaction was monitored by TLC and finally by HPLC for the confirmation of complete removal of carbonyl portion. The reaction mixture was cooled, filtered, residual portion was washed with ethyl alcohol and filtrate was concentrated under vacuum. Further HPLC grade hexane was added to the concentrate and refluxed for 5 hours to extract NCCL from ethanol, care was taken to see that no crystal formation was observed on retaining the NCCL. Yield of NCCL: 3 g per 5 g of C. oil (60%) (Indian Patent application filed no. 1940/DEL/2014).
2.3 Isolation and identification of marker compound from NCCL
The HPLC system (Class-VP, Shimadzu Corporation, Kyoto, Japan) consisted of binary gradient pump (LC-20AP), photo diode array (PDA) detector (SPD M20A) and system controller (CBM-20A). CLASS-VP workstation was used for data acquisition. The preparative column C18 (250 mm, 20.0 mm, 15 μm, Phenominex) was used for the study. The mobile phase consisted of acetonitrile
:
water (50
:
50, v/v) was duly filtered through 0.22 μm Millipore filter (Billerica, USA) and degassed ultrasonically for 20 min prior to use. The diode array detector was set at 220 and 254 nm (PDA detection) with a total runtime of 60 min. The flow rate was maintained at 5 mL min−1. NCCL (200 mg) was dissolved in acetonitrile (5 mL) and injected onto a prep HPLC column. The fractions containing marker compound were separated and concentrated under vacuum. The marker compound was characterized as 7,7-dimethyl-5-(2-p-tolylpropyl)-6,7-dihydro-1,3,4-oxadiazepin-2-amine (compound-I) employing different spectral techniques like FTIR, 1H and 13C NMR (Fig. S4A and B†), mass. The purity of these samples were checked by Thin Layer Chromatography (TLC) and HPLC (Fig. S4C†) and found >99%.
1H NMR (400 MHz, DMSO-d6): δ 7.13 (d, 2H, J = 7.68), δ 7.08 (d, 2H, J = 7.56), δ 5.93 (S, 2H), δ 3.01 (dd, 1H, J = 6.90), δ 2.5 (m, 4H), δ 2.24 (s, 3H), δ 1.31 (s, 3H), δ 1.23 (s, 3H), δ 1.18 (d, 3H, J = 6.72).
13C NMR (400 MHz, DMSO-d6): 155.41, 153.28, 142.96, 135.04, 128.86, 126.69, 61.66, 50.85, 37.98, 36.55, 26.00, 25.78, 22.29, 20.59.
IR (neat) ν (cm−1) 3411.7, 3015.2, 1667.2, 1564.5, 1514.7, 1448.0, 1216.1, 1054.7, 759.7, 669.1, 544.7.
Mass m/z 274.5 (M+ + 1), (M+ + 2) 275.6, HRMS m/z 274.1932 (M+ + 1), 275.1956 (M+ + 2).
2.4 Preparation of standard and quality control samples
Stock solution of NCCL-200 μg mL−1 was prepared and serially diluted up to 2 μg mL−1 with methanol. All the dilutions of NCCL (2–200 μg mL−1) were stored at −20 °C and were brought to room temperature before use. To prepare the standard plasma calibration samples, 50 μL of standard dilutions were fortified to 200 μL of blank plasma. The mixture was then treated following extraction procedure. Standard plasma concentrations of NCCL were in the range 0.5–50 μg mL−1 for NCCL.
2.5 Bioavailability studies
Determination of bioavailability was based on estimation of marker compound-(I) 7,7-dimethyl-5-(2-p-tolylpropyl)-6,7-dihydro-1,3,4-oxadiazepin-2-amine (Fig. S1D†) present in NCCL. Based on the area% compound (I) was not less than 25% of NCCL. Its purity was ascertained by TLC and HPLC and it was found to be more than 99% pure. The fingerprint profile of NCCL containing marker compound (I) eluting at 4.3 min was established.
The in vivo oral bioavailability studies were performed in rats. NCCL was administered orally at a dose of 100 mg kg−1. Blood samples were collected at pre-defined time intervals (0, 1, 3, 6, 9, 12, 24, 36, and 48 hours). Three animals were available at each time point. Plasma was separated by centrifuging the blood at 13
000 rpm for 10 min and stored frozen at −80 ± 5 °C until analysis. To 0.20 mL of plasma, 0.5 mL of methanol was added and the resulting solution was thoroughly vortex-mixed for 2 minutes. After centrifugation at 3000g for 10 min, the supernatant layer was transferred into a clean test tube, concentrated to dryness under vacuum, reconstituted in 50 μL of methanol and 20 μL of which were injected into the HPLC system for analysis. Concentrations were calculated from the respective calibration curve and pharmacokinetic parameters were evaluated.
2.6 Myocardial ischemia reperfusion (MI/RP) injury
Wistar rats were grouped into vehicle (0.25% carboxymethyl cellulose; CMC) treated sham, vehicle (0.25% carboxymethyl cellulose; CMC) treated MI/RP, NCCL (50
100
125 mg kg−1) treated sham or NCCL (50
100
125 mg kg−1) treated MI/RP groups and each group comprised of a minimum of six rats. All the rats underwent MI/RP surgery as described previously.13 In brief, rats were anesthetized with intraperitoneal injection of ketamine/xylazine (80/10 mg kg−1). Left anterior descending coronary artery (LAD), 3–4 mm from its origin, was ligated for a period of 30 min by using 5–0 suture (Ethicon, non-absorbable surgical suture U.S.P, Johnson & Johnson) and reperfused for 3 days. During the study all the animals were treated with either vehicle or NCCL before and after surgery as described in Fig. 1A. TTC was used to determine the infarct size as described previously.13
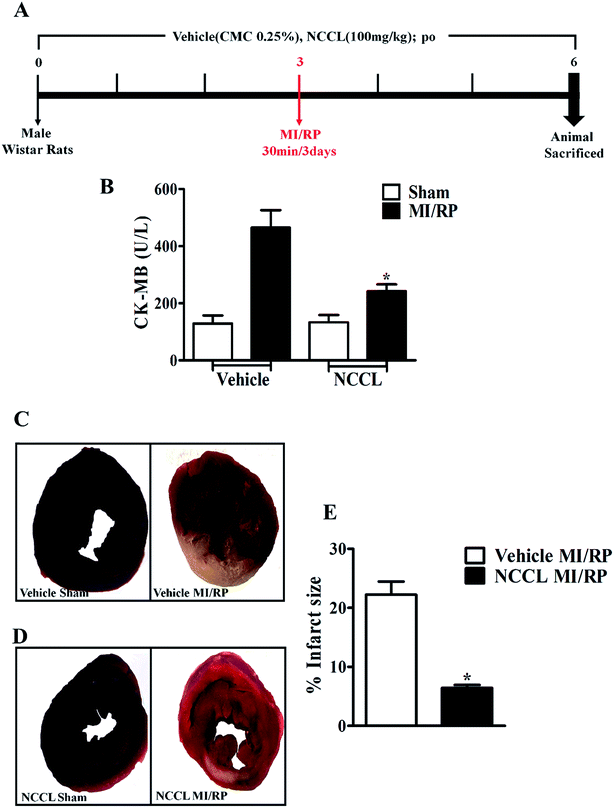 |
| Fig. 1 NCCL exerts protection against MI/RP injury in rats. (A) Pre and post treatment schedule for rats undergoing MI/RP injury. B, serum CK-MB levels (U L−1) after 3 hours of reperfusion injury, *p = 0.0443 versus vehicle MI/RP. (C and D), TTC-stained heart sections obtained after 3 days of reperfusion injury with vehicle (CMC 0.25%) and NCCL (100 mg kg−1). (E) Percent infarct size of TTC-stained heart sections, *p < 0.0001 versus vehicle MI/RP. All the values are expressed in mean ± SEM. N = 3. | |
2.7 Biochemical estimation
As described previously, CK-MB activity was measured in serum after a period of 3 hours of MI/RP and change in absorbance (ΔA) was measured spectrophotometrically at the wavelength of 340 nm as per manufacturer's protocol (Merck Diagnostic). Enzyme activity was expressed as CK-MB activity (U L−1) = ΔA/min × 1650.13
2.8 Endothelial microparticle (EMP) generation and characterization
To mimic inflammatory condition, EA.hy926 endothelial cells were stimulated either with TNF-α-10 ng mL−1 or NCCL-10 μg mL−1 simultaneously for 6 hour. After 6 hours, supernatant was collected and centrifuged at 1931g for 15 min to remove cell debris and subsequently ultracentrifuged at 100
000g for 90 min at 4 °C to pellet down the endothelial microparticles. Endothelial microparticles were labelled with anti-CD105-FITC (Abcam, AB53318) for 30 min and analyzed using flow cytometry. The cytometer was calibrated with predetermined sized beads (Invitrogen) and plotted in FSC-SSC dot plot; the number of fluorescent events lying within this EMP gating was measured. Estimation of endothelial microparticles in plasma samples after MI/RP was performed using anti-CD146-PE (Abcam, AB78488) antibody labelling as described above.14,15
2.9 Endothelial dysfunction
The thoracic aorta was excised from the anaesthetized animals and immediately placed in ice cold Krebs bicarbonate solution (118 mM NaCl; 5 mM KCl; 2.5 mM CaCl2; 1.2 mM MgSO4; 1.2 mM KH2PO4; 25 mM NaHCO3; 11 mM glucose; and 0.03 mM EDTA; pH 7.4). The aorta was cut into rings (5 mm in length) after clearing the connective tissue and fat. The rings were mounted vertically between two transducers in organ chambers filled with 10 mL Krebs solution maintained at 37 ± 0.5 °C constantly bubbled with 95% O2/5% CO2. For the recording of isometric tension one stirrup was connected to an anchor and the other to a force transducer (SPEL Advanced Isosys organ bath, Experimetria, Hungary). Then, rings were equilibrated followed by 60 min washing. After equilibration period, contraction–relaxation response was determined with phenylephrine/acetylcholine in all groups of animals. Ring viability and maximum contraction were assessed by 80 mM KCl.16
2.10 ELISA
After 3 days of post treatment, animals were sacrificed blood was collected with 1.9% tri-sodium citrate and plasma was separated. The isolated plasma samples were analyzed for TNF-α, IL-6 and IFN-γ cytokines (BD Biosciences) as per the manufacturer's protocol.
2.11 Isolation of rat myocardial endothelial cells
Rat heart specific endothelial cells were isolated using same protocol as described previously.13,17 In brief, heart was dissected, and excess blood was washed off with chilled phosphate buffer saline (pH 7.4) and minced. Hearts were digested by using collagenase (2 mg mL−1) supplemented with 1% BSA at 37 °C. Rat Anti Mouse CD31 (BD Biosciences) coated magnetic beads were used to separate CD31+ cells and processed further for RT-PCR.
2.12 Real-time polymerase chain reaction (RT-PCR)
RNA was extracted from EA.hy926 endothelial cells and myocardial endothelial cells using Trizol (Invitrogen), and a total of 1 μg of RNA was processed for cDNA synthesis using cDNA Synthesis Kit as per the manufacturer's protocol (Fermentas). Different genes like PDGF, vWF, Annexin-V, PDGF-B, E-selectin, Lox-1, ICAM-1, GAPDH and 18s RNA were amplified using Real-time PCR (Roche cycler 480). Expression of each target gene was normalized to GAPDH or 18s RNA and the data were expressed as fold change.
2.13 Static and flow based cellular interaction
EA.hy926 endothelial cells were grown on collagen (0.5 mg mL−1) precoated slides, either treated with 10 μg mL−1 dose of NCCL, LPS (1 μg mL−1), polymyxin B-(10 μg mL−1) or vehicle control for 6 hours. THP-1 monocytic cells were labeled with Calcein-AM (C3100 MP, Invitrogen, India) were allowed to interact with EA.hy926 endothelial cells under static conditions for 10 min or under flow conditions with a flow rate of 1 mL min−1. The adhered cells were fixed with neutral buffered formalin (pH 7.4) and mounted using an aqueous mounting medium (Fisher Scientific, India). The interaction of EA.hy926 endothelial cells with THP-1 monocytes was micrographed using a fluorescent microscope (Leica DM5000 and Leica DM6000). All the fields were fixed and mounted using water based mounting medium and quantified using Leica Qwin software (Leica QWinPlus V 3.5.1, Switzerland).
2.14 Statistics
Data expressed as mean ± SEM from a minimum of three different experiments. All the groups were evaluated for the level of significance by using unpaired Students t-test. A probability value of ≤0.05 was considered as statistically significant.
3. Results
3.1 NCCL exerts protection against MI/RP injury in rats
To ascertain the infarct healing potential, rats were treated with NCCL-100 mg kg−1 pre and post MI/RP injury (Fig. 1A). CK-MB is widely considered as a reliable biomarker of successful MI/RP injury, here we observed a significant decrease in plasma CK-MB level in NCCL treated MI/RP rats after three hour of reperfusion (242.35 U L−1 vs. 464.67 U L−1, p = 0.0443) as compared to the vehicle MI/RP rats (Fig. 1B). TTC stain was used as a hallmark of viability, gross observation revealed a significant reduction in infarct zone in NCCL treated rats as compared to vehicle treated MI/RP rats, furthermore, no pale color was observed in sham animals of vehicle and NCCL treated groups (Fig. 1C and D). Quantification of ischemic ventricular area revealed a marked reduction in NCCL treated MI/RP rats (6.44% vs. 22.22%, p < 0.0001) as compared to the vehicle MI/RP left ventricular area after three days of reperfusion period (Fig. 1E). Further, 100 mg kg−1 dose is effective and had similar potent inhibitory effect compared to 125 mg kg−1 dose. However, 50 mg kg−1 dose of NCCL also reduced the infarct zone compared to the control but not as effective to 100 mg kg−1 dose. Hence, our further experiments were limited to the 100 mg kg−1 dose (Fig. S1A–C and E†).
3.2 NCCL decreased the circulatory pro-inflammatory cytokines and endothelial microparticles after MI/RP injury in rats
Post MI/RP injury has been shown to induce a variety of stress factors and inflammatory cytokines like TNF-α, IL-6 and IFN-γ are released into circulation18–20 these circulating inflammatory cytokines, overtly activates endothelium thus affecting its functionality. To ascertain the levels of circulatory pro-inflammatory cytokines in MI/RP rats, we performed ELISA on NCCL treated MI/RP rats. In NCCL treated rats, we found a significant reduction in the levels of TNF-α (34.08 pg mL−1 vs. 90.12 pg mL−1, p < 0.0001, Fig. 2A), IL-6 (34.19 pg mL−1 vs. 73.95 pg mL−1, p < 0.0001, Fig. 2B) and IFN-γ (24.68 pg mL−1 vs. 79.45 pg mL−1, p = 0.0363, Fig. 2C) as compared to that of vehicle MI/RP rats, while sham animals had not shown any significant change in their plasma levels after NCCL treatment compared to vehicle sham group. Overtly activated endothelium has been shown to release endothelial microparticles. We hypothesized that the reduced pro inflammatory factors, might affect endothelial microparticle release. NCCL treated MI/RP rats showed a significant decrease in plasma EMP levels three days post MI/RP injury (10.12 vs. 35.94, p = 0.0138) as compared to vehicle MI/RP rats, while in sham animals no significant change in EMP levels was observed (Fig. 2D and S2A†).
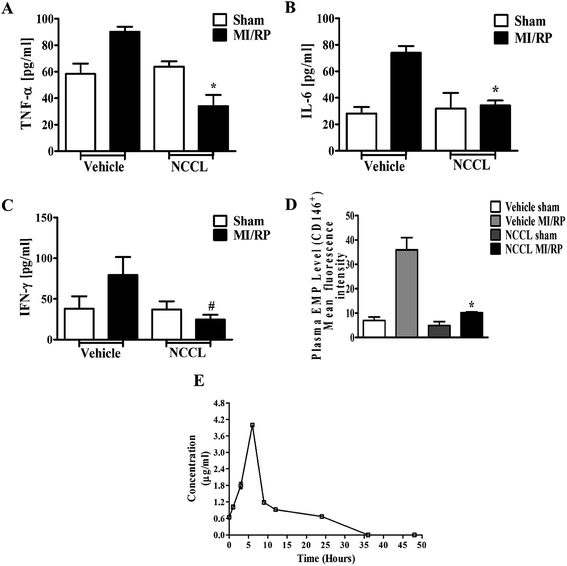 |
| Fig. 2 NCCL treatment reduced inflammatory cytokine levels after 3 days of MI/RP injury. (A) Plasma TNF-α level. (B) Plasma IL-6 level. (C) Plasma IFN-γ level. (D) Plasma EMP level after 3 days of treatment with vehicle (CMC 0.25%) and NCCL (100 mg kg−1) in MI/RP injured rats, *p = 0.0138 versus vehicle MI/RP. (E) Plasma pharmacokinetic profiling in rats. All the values are expressed in mean ± SEM. *p < 0.0001, #p = 0.0363 versus vehicle MI/RP. N = 3. | |
The plasma concentration of marker compound (I) of NCCL in extracted plasma samples was checked after storage over 5 days and found to be stable under the storage conditions (Fig. S1D†). All subjects exhibited late absorption of marker compound (I), which appeared to peak at 6 hours. Cmax for compound (I) was about 4.00 ± 0.09 μg mL−1 equivalent to NCCL; Tmax being 6 hours. Plasma concentration of compound (I) were below detection limit at and above 36 hours; thus validating late absorption and elimination of marker compound (I). Overall systemic availability of compound (I) was found to be 52.56 ± 4.11 h μg mL−1 equivalent to NCCL (Fig. 2E).
3.3 NCCL improves endothelial function in MI/RP induced rats
To assess the vasomodulatory effect, we performed the endothelial function assay using myograph after MI/RP injury in rats. Sections of aorta relaxed efficiently with different doses of acetylcholine (Ach) (3 nM to 300 μM) induced concentration dependent vasodilation. NCCL treated rat aortic segments after MI/RP injury, showed a significantly effective relaxation at 300 nM (39.57% ± 4.49 vs. 17.70% ± 2.54, p = 0.0128), 3 μM (57.40% ± 6.35 vs. 28.43% ± 2.15, p < 0.0001), 30 μM (69.79% ± 4.49 vs. 31.38% ± 1.41, p < 0.0001) and 300 μM (73.02% ± 4.36 vs. 33.80% ± 1.03, p < 0.0001) dose of acetylcholine as compared to that of vehicle MI/RP rats. However, the sham animals did not affect the relaxation pattern upon induction with acetylcholine, in vehicle as well as NCCL treated rats (Fig. 3A and B). These results suggest that NCCL treatment improved the endothelial function in MI/RP rats.
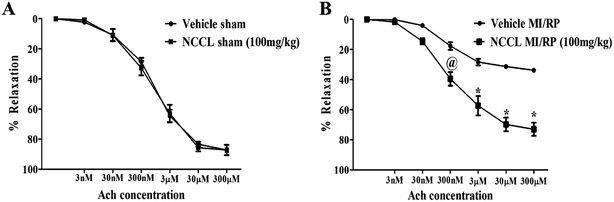 |
| Fig. 3 NCCL treatment had improved endothelial function in MI/RP injured rats. Concentration-dependent relaxation of acetylcholine (3 nM to 300 μM) in rat thoracic aortic rings pre-contracted with phenylephrine hydrochloride (1 mM). (A) Relaxation pattern observed in vehicle sham and NCCL sham treated rats. (B) Relaxation pattern in vehicle and NCCL treated MI/RP rats, at 300 nM, @p = 0.0128 NCCL MI/RP versus vehicle MI/RP, at 3 μM, 30 μM and 300 μM, *p < 0.0001 NCCL MI/RP versus vehicle MI/RP at 300 nM, 3 μM, 30 μM and 300 μM. All the values are expressed in mean ± SEM. N = 3. | |
3.4 NCCL exerts its effect by regulating the inflammatory and apoptotic factors induced under MI/RP condition on endothelial cells
To determine the possible mechanism behind NCCL anti-inflammatory property, mRNA expression of various genes regulating inflammatory factors, specifically assessed on endothelial cells, isolated from ischemic and non-ischemic zone of rat heart were analyzed using real time PCR. After three days of reperfusion, pre and post treatment of NCCL significantly reduced the mRNA expression levels of pro-inflammatory factor vWF, on endothelial cells from ischemic zone (fold change 0.550 vs. 1.53, p < 0.0344, Fig. 4A) as compared to non-ischemic zone. Similar patterns were observed in the mRNA expression of PDGF and Lox-1 (fold change 0.534 vs. 0.788, p = 0.0240; 0.091 vs. 0.373, p = 0.0186 Fig. 4B and C). The levels of Annexin V mRNA expression was also significantly reduced in NCCL treated endothelial cells from the MI/RP ischemic zone (fold change 0.511 vs. 1.34, p = 0.0084) as compared to the non-ischemic endothelial cells.
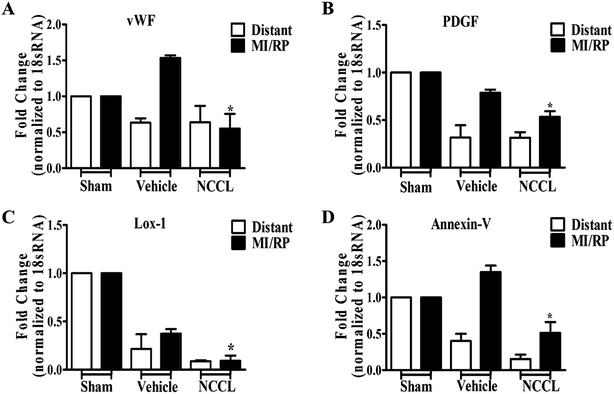 |
| Fig. 4 NCCL treatment reduced the expression of inflammatory and apoptotic marker onto endothelial cells induced by MI/RP injury. (A) Expression of von Willebrand factor, *p = 0.0344 versus vehicle MI/RP. (B) The expression of PDGF, *p = 0.0240 versus vehicle MI/RP. (C) Expression of lectin-type oxidized LDL receptor-1 (Lox-1), *p = 0.0186 versus vehicle MI/RP. (D) Expression of Annexin-V, *p = 0.0084 versus vehicle MI/RP, on endothelial cells isolated from heart after 3 days of reperfusion injury. All the values are expressed in mean ± SEM. N = 3. | |
3.5 NCCL treatment reduced the recruitment of monocytes to endothelial cells under static and flow based conditions
We hypothesized that reduced inflammatory signaling would lead to lesser activation of endothelial cells. We used static and flow based cell–cell interaction to ascertain the effect of change in inflammatory factors. NCCL treated EA.hy926 endothelial cells showed a significant reduction (222.32 vs. 566.874, *p = 0.0191, Fig. 5A and B) in the adherence of calcein labelled THP-1 cells as compared to vehicle control under static based conditions. While under, flow based condition NCCL treated cells has markedly reduced the interaction between monocytes and endothelial cells (119.66 vs. 358.98, *p = 0.0325, Fig. 5C and D) as compared to vehicle control. Phase contrast images were captured to show the equal number of endothelial cells was plated in both the groups (Fig. 5 and S3A–D†). Further, to compare the effect of NCCL with a known anti-inflammatory agent, we used polymyxin B, as inhibitor of LPS mediated effects. Addition of LPS increased the interaction between THP-1 monocytes and endothelial cells (LPS vs. control; 1258.45 vs. 390.35 and 1793.2 vs. 547.94) both under static and flow based condition respectively. However, the interaction between THP-1 monocytes and endothelial cells were markedly reduced with the treatment of polymyxin B (polymyxin B vs. LPS; 397.24 vs. 1258.45, *p = 0.0004) and NCCL (NCCL vs. LPS; 441.983 vs. 1258.45, #p = 0.0005) under static condition (Fig. 5E and F and S3E–H†). Similar reduction was observed in polymyxin B (polymyxin B vs. LPS; 517.158 vs. 1793.2, *p = 0.0003) and NCCL (NCCL vs. LPS; 639.49 vs. 1793.2, #p = 0.0020) under flow based condition (Fig. 5G and H and S3I–L†). These results suggest that NCCL inhibits the inflammatory cellular interaction between monocytes and endothelial cells on par with the known potent LPS inhibitor. Thus, leading to the reduced initiation of inflammation.
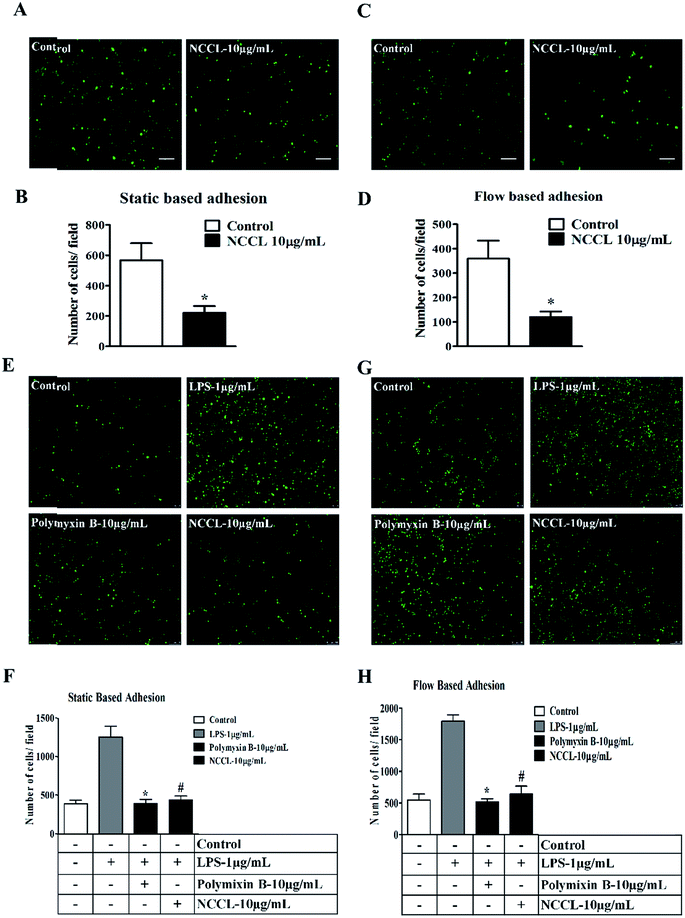 |
| Fig. 5 NCCL treatment inhibited the adhesion of monocytes to endothelial cells under static and flow based condition. (A) Adhesion of THP-1 monocytes to EA.hy926 cells treated with NCCL-10 μg mL−1 under static condition. (B) Quantification of THP-1 monocytes adhered onto EA.hy926 cells, *p = 0.0456 versus control. (C) Adhesion of THP-1 monocytes to EA.hy926 cells treated with NCCL-10 μg mL−1 under flow based conditions. (D) Quantification of THP-1 monocytes adhered onto EA.hy926 cells, *p = 0.0368 versus control. (E) Adhesion of THP-1 monocytes onto EA.hy926 endothelial cells: stimulated with LPS-1 μg mL−1 and treated with or without polymyxin-B-10 μg mL−1, and NCCL-10 μg mL−1 under static condition. (F) Quantified data of THP-1 monocytes adhered onto EA.hy926 cells, *p = 0.0004, #p = 0.0005 versus LPS. (G) Adhesion of THP-1 monocytes to EA.hy926 endothelial cells: stimulated with LPS-1 μg mL−1 and treated with or without polymyxin B-10 μg mL−1, and NCCL-10 μg mL−1 treated EA.hy926 endothelial cells under flow based condition. (H) Quantified data of THP-1 monocytes adhered to EA.hy926 endothelial cells, *p = 0.0003, #p = 0.0020 versus LPS. All the values are expressed in mean ± SEM. N = 5. | |
3.6 NCCL exhibits the down regulation of adhesion factors on endothelial cells
To elucidate the mechanism behind reduced adherence of monocytes on endothelial cells, here we evaluated the expression levels of important adhesion molecules expressed on endothelial cells during inflammation using realtime PCR. Six hours treatment of NCCL-10 μg mL−1 significantly reduced the mRNA expression of vWF (fold change 0.32, *p < 0.05), PDGF-B (fold change 0.579, *p < 0.05), ICAM-1 (fold change 0.398, #p < 0.01) and E-selectin (fold change 0.248, #p < 0.01) as compared to control cells (Fig. 6A). Further, to determine the anti-inflammatory potential of NCCL, we treated EA.hy926 endothelial cells with either TNF-α (10 ng mL−1) or NCCL-10 μg mL−1 for 6 hours, then supernatant was evaluated for EMP generation. NCCL treatment led to a significant reduction in EMP generation as compared to TNF-α (10 ng mL−1) (Fig. 6B and S2B†). Together these results indicate that NCCL has an anti-inflammatory potential which decreased the expression of adhesion factors and reduced EMP generation and hence rescued the pathological condition of inflammation.
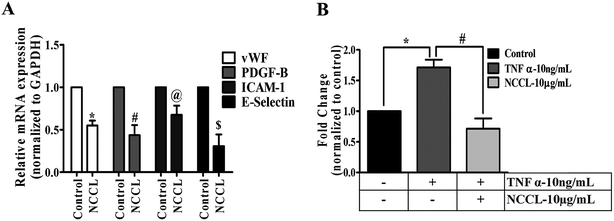 |
| Fig. 6 NCCL reduced the expression of adhesion factors on EA.hy926 endothelial cells. (A) RT-PCR for expression of adhesion factors on EA.hy926 cells after 6 hours of NCCL (10 μg mL−1) treatment. *p = 0.0013, #p = 0.0089, @p = 0.0406, $p = 0.0074, versus control. (B) EA.hy926 endothelial cells had shown a significant decrease in EMP generation after 6 hours of stimulation either with TNF-α or NCCL *p = 0.0004 versus control, #p = 0.0013 versus TNF-α. All the values are expressed in mean ± SEM. N = 3. | |
4. Discussion
Myocardial ischemia/reperfusion (MI/RP) injury is well known to activate endothelial cell mediating inflammation. The increased circulating inflammatory molecules further activate endothelial cell led to the generation endothelial microparticles. These microparticles are further known to activate the pathological condition have remained obscure. Our present work shows an important contribution of NCCL as a potent extract of C. oil, reducing endothelial microparticle generation, leading to reduced inflammation under MI/RP injury in rats.
NCCL being a potent fraction from C. oil has shown a similar salutary effect at lower dose, suggesting the concentrated effect of the active component than its parent compound. Further, pharmacokinetic study suggests the bioavailability of NCCL 52.56 ± 4.11 h μg mL−1 was higher as compared to its parent compound C. oil.21
Pre and post conditioning of rats with NCCL significantly reduced the infarct size, inflammatory cytokine load22–24 and circulating EMP levels. This reduction in infarct size might be attributed to reduced inflammatory cytokine load eventually leading to reduced activation of endothelium, there by reduced circulating endothelial microparticles. Endothelial microparticles have been shown obscurely, to aggravate the MI/RP.7 However, very obscurely it has been shown that microparticles induces trans-signaling of the endothelial cells, to flag the endothelial cells of immediate danger and induce the expression of adhesion factors to initiate inflammation.25 Further, these endothelial microparticles have the capability to travel long distances and could adhere strongly to parent cell or other cells to initiate and progress inflammation. We observed that endothelial cells isolated from infarcted region has significantly higher inflammatory signature markers as compared to the distant region from same heart after MI/RP, suggesting that in the MI/RP condition, there is spurt in the inflammatory factors specifically in the injured area and leaving the distant area unaltered. Treatment with NCCL tightly regulates the inflammation in the injured area by reducing the inflammatory markers. Several lines of evidence point that inflammation in the infarct area contributes to the disease aggravation.26 Endothelial cells once inflamed releases endothelial microparticles for stimulating distant endothelial cells to induce inflammation.27 NCCL treatment reduced the endothelial microparticle generation thus leading to reduced inflammation. This could be in part due to the reduced activation of distant endothelium by the released endothelial microparticles, thereby reducing the mass activation of endothelial cells in the distant region.
MI/RP injury results in endothelial dysfunction followed by an increased oxidative load which ultimately results change in endothelium relaxation pattern.6,28 Our data suggested that NCCL improved the endothelial functionality by improving its relaxation pattern by decreasing the inflammatory cytokine load. The improved endothelial functionality could be due to the reduced endothelial microparticle thereby reduced endothelial dysfunction. This observation could also be corroborated with the reduced adhesion of THP-1 monocytes onto the endothelium, which is known to induce endothelial dysfunction.29 NCCL treatment also reduced the LPS induced THP-1 monocytes to the endothelium on par with polymixin B, suggesting a potent anti-inflammatory effect. It could be that NCCL could directly bind to LPS binding site TLR-4 and inhibit the downstream inflammatory signals. These evidences suggest that NCCL has great potential in reducing the endothelial cell induced inflammation.
MI/RP injury activates several pro-inflammatory pathways culminating in the expression of several adhesion molecules, which further induces a potent inflammatory cascade leading towards the release of cytokines like TNF-α, IL-6, IFN-γ etc.30–32 Prolonged exposure to these pro-inflammatory cytokines leads to the activation of adhesion molecules, increased oxidative load and contribute in shedding of EMPs. Our finding has contributed towards a therapeutic lead by decreasing the expression of pro-inflammatory genes like ICAM-1 and E-selectin on endothelial cells. In support of this, we show a significant reduction in EMP level after treatment either with NCCL or TNF-α in the EA.hy926 endothelial cells.
To mimic the physiological condition, we analyzed the effect of NCCL under static and flow based condition. We observed a significant reduction in THP-1 adherence on to EA.hy926 endothelial cells under static and flow based condition by down regulating the expression of adhesion molecules. It might also be possible that NCCL might dock to several receptors blocking the adherence of THP-1 monocytes to endothelial cells or the compound could directly block the expression of transcription factors that regulate adhesion factors expression.
The current study shows a significant feature of NCCL that removal of all carbonyl groups from parent molecule has increased the biological activity and efficacy better than its parent compound. Here, through our findings we concluded that (a) NCCL treatment has shown an infarct sparing property at lower dose compared to its parent compound in MI/RP rats. (b) NCCL treatment had reduced level of inflammatory cytokines such as TNF-α, IL-6 and IFN-γ as well as circulating endothelial microparticles, tightly controlling the endothelial cell inflammation (c) similar observations were observed in vitro treatment of NCCL on endothelial cells, which had significantly decreased the expression of adhesion molecules like ICAM-1 and E-selectin, thus resulting in the reduction of adherence of THP-1 cells to EA.hy926 endothelial cells. Overall our study concludes that NCCL interference reduced the endothelial cell mediated inflammation by reduced endothelial microparticle generation leading to decreased MI/RP injury in rats.
Conflict of interest
The authors have no conflicts of interest; the data in this manuscript has not been previously published; and this data is not being considered for publication concurrently elsewhere.
Abbreviations
ΔA | Change in absorbance |
μg mL−1 | Microgram per milliliter |
μL | Microliter |
μM | Micromolar |
nM | Nanomolar |
μm | Micrometer |
mm | Millimeter |
mM | Millimolar |
C. oil | Curcuma oil |
CaCl2 | Calcium chloride |
CMC | Carboxymethyl cellulose |
EDTA | Ethylenediaminetetraacetic acid |
EMPs | Endothelial microparticles |
E-selectin | CD62 antigen-like family member E |
FBS | Fetal bovine serum |
GAPDH | Glyceraldehyde-3-phosphate dehydrogenase |
g | Gram |
HPLC | High performance liquid chromatography |
ICAM-1 | Intercellular adhesion molecule 1 |
IFN-γ | Interferon-γ |
IL-6 | Interleukin-6 |
KCl | Potassium chloride |
KH2PO4 | Potassium dihydrogen phosphate |
LAD | Left anterior descending coronary artery |
LOX-1 | Lectin-type oxidized LDL receptor 1 |
mg kg−1 | Milligram per kilogram |
mm | Millimeter |
mM | Millimolar |
MgSO4 | Magnesium sulfate |
MI/RP | Myocardial ischemia/reperfusion |
min | Minute |
NaCl | Sodium chloride |
NaHCO3 | Sodium bicarbonate |
NCCL | Non-carbonyl Curcuma longa fraction |
ng mL−1 | Nanogram per milliliter |
PDGF | Platelet-derived growth factor |
PDGF-B | Platelet-derived growth factor subunit B |
pH | Potential of hydrogen |
rpm | Revolutions per minute |
RT-PCR | Real time polymerase chain reaction |
THP-1 | Human monocyte cell line |
TLC | Thin layer chromatography |
TNF-α | Tissue necrosis factor-α |
U L−1 | Unit per liter |
vWF | Von Willebrand factor |
Acknowledgements
This work was supported by the grants from CSIR-CDRI Network project: “Towards holistic understanding of complex diseases: Unravelling the threads of complex disease” (THUNDER) BSC0102, UNDO BSC0103 of Council of Scientific and Industrial Research, Government of India and Ramalingaswami fellowship to Kumaravelu Jagavelu from Department of Biotechnology, Government of India and University grants commission Senior Research fellowship to Dipti Tripathi, Council of Scientific and Industrial Research Senior research fellowship to Dipika Goyal and Hafsa Ahmad and Indian Council of Medical Research, Senior Research fellowship to Amit Manhas. The authors acknowledge Dr A. L. Vishwakarma for assisting with Flow cytometry. This manuscript is allotted with CDRI communication number: 9241.
References
- N. G. Frangogiannis, C. W. Smith and M. L. Entman, Cardiovasc. Res., 2002, 53, 31–47 CrossRef CAS PubMed.
- K. Bhagat, Cardiovasc. Res., 1998, 39, 312–317 CrossRef CAS PubMed.
- D. L. Carden and D. N. Granger, J. Pathol., 2000, 190, 255–266 CrossRef CAS PubMed.
- J. L. Park and B. R. Lucchesi, Ann. Thorac. Surg., 1999, 68, 1905–1912 CrossRef CAS PubMed.
- D. M. Yellon and D. J. Hausenloy, N. Engl. J. Med., 2007, 357, 1121–1135 CrossRef CAS PubMed.
- A. M. Lefer, P. S. Tsao, D. J. Lefer and X. L. Ma, FASEB J., 1991, 5, 2029–2034 CAS.
- C. M. Boulanger, A. Scoazec, T. Ebrahimian, P. Henry, E. Mathieu, A. Tedgui and Z. Mallat, Circulation, 2001, 104, 2649–2652 CrossRef CAS PubMed.
- M. Zielinska, W. Koniarek, J. H. Goch, B. Cebula, M. Tybura, T. Robak and P. Smolewski, Kardiol. Pol., 2005, 62, 531–542 Search PubMed ; discussion 543–534.
- L. Bernal-Mizrachi, W. Jy, J. J. Jimenez, J. Pastor, L. M. Mauro, L. L. Horstman, E. de Marchena and Y. S. Ahn, Am. Heart J., 2003, 145, 962–970 CrossRef PubMed.
- M. Ray, R. Pal, S. Singh and N. M. Khanna, US Pat., US 6991814 B2, 2006.
- P. Dohare, P. Garg, U. Sharma, N. R. Jagannathan and M. Ray, BMC Complementary Altern. Med., 2008, 8, 55 CrossRef PubMed.
- P. Dohare, S. Varma and M. Ray, Nitric Oxide, 2008, 19, 1–11 CrossRef CAS PubMed.
- A. Manhas, V. Khanna, P. Prakash, D. Goyal, R. Malasoni, A. Naqvi, A. K. Dwivedi, M. Dikshit and K. Jagavelu, J. Cardiovasc. Pharmacol., 2014, 64, 228–236 CrossRef CAS PubMed.
- D. Povero, A. Eguchi, I. R. Niesman, N. Andronikou, X. de Mollerat du Jeu, A. Mulya, M. Berk, M. Lazic, S. Thapaliya, M. Parola, H. H. Patel and A. E. Feldstein, Sci. Signaling, 2013, 6, 1–14 CrossRef PubMed.
- V. Combes, A. C. Simon, G. E. Grau, D. Arnoux, L. Camoin, F. Sabatier, M. Mutin, M. Sanmarco, J. Sampol and F. Dignat-George, J. Clin. Invest., 1999, 104, 93–102 CrossRef CAS PubMed.
- M. Jain, M. K. Barthwal, W. Haq, S. B. Katti and M. Dikshit, Chem. Biol. Drug Des., 2012, 79, 459–469 CAS.
- R. C. Huebert, K. Jagavelu, A. F. Liebl, B. Q. Huang, P. L. Splinter, N. F. LaRusso, R. A. Urrutia and V. H. Shah, Lab. Invest., 2010, 90, 1770–1781 CrossRef PubMed.
- M. W. Irwin, S. Mak, D. L. Mann, R. Qu, J. M. Penninger, A. Yan, F. Dawood, W. H. Wen, Z. Shou and P. Liu, Circulation, 1999, 99, 1492–1498 CrossRef CAS PubMed.
- M. Gwechenberger, L. H. Mendoza, K. A. Youker, N. G. Frangogiannis, C. W. Smith, L. H. Michael and M. L. Entman, Circulation, 1999, 99, 546–551 CrossRef CAS PubMed.
- M. Nian, P. Lee, N. Khaper and P. Liu, Circ. Res., 2004, 94, 1543–1553 CrossRef CAS PubMed.
- P. Prakash, A. Misra, W. R. Surin, M. Jain, R. S. Bhatta, R. Pal, K. Raj, M. K. Barthwal and M. Dikshit, Thromb. Res., 2011, 127, 111–118 CrossRef CAS PubMed.
- A. Matsumori, Y. Furukawa, T. Hashimoto, A. Yoshida, K. Ono, T. Shioi, M. Okada, A. Iwasaki, R. Nishio, K. Matsushima and S. Sasayama, J. Mol. Cell. Cardiol., 1997, 29, 419–423 CrossRef CAS PubMed.
- R. S. Vasan, L. M. Sullivan, R. Roubenoff, C. A. Dinarello, T. Harris, E. J. Benjamin, D. B. Sawyer, D. Levy, P. W. Wilson and R. B. D'Agostino, Circulation, 2003, 107, 1486–1491 CrossRef CAS PubMed.
- X. Gao, H. Zhang, S. Belmadani, J. Wu, X. Xu, H. Elford, B. J. Potter and C. Zhang, Am. J. Physiol.: Heart Circ. Physiol., 2008, 295, H2242–H2249 CrossRef CAS PubMed.
- A. M. Curtis, P. F. Wilkinson, M. Gui, T. L. Gales, E. Hu and J. M. Edelberg, J. Thromb. Haemostasis, 2009, 7, 701–709 CrossRef CAS PubMed.
- Q. Gu, X. P. Yang, P. Bonde, A. DiPaula, K. Fox-Talbot and L. C. Becker, J. Cardiovasc. Pharmacol., 2006, 48, 320–328 CrossRef CAS PubMed.
- S. K. Lee, S. H. Yang, I. Kwon, O. H. Lee and J. H. Heo, Thromb. Haemostasis, 2014, 112, 580–588 CrossRef CAS PubMed.
- J. Bauersachs, A. Bouloumie, D. Fraccarollo, K. Hu, R. Busse and G. Ertl, Circulation, 1999, 100, 292–298 CrossRef CAS PubMed.
- A. M. van der Laan, E. N. Ter Horst, R. Delewi, M. P. Begieneman, P. A. Krijnen, A. Hirsch, M. Lavaei, M. Nahrendorf, A. J. Horrevoets, H. W. Niessen and J. J. Piek, Eur. Heart J., 2014, 35, 376–385 CrossRef CAS PubMed.
- N. G. Frangogiannis, Curr. Med. Chem., 2006, 13, 1877–1893 CrossRef CAS PubMed.
- V. R. Taqueti, R. N. Mitchell and A. H. Lichtman, Annu. Rev. Physiol., 2006, 68, 67–95 CrossRef CAS PubMed.
- D. J. Marchant, J. H. Boyd, D. C. Lin, D. J. Granville, F. S. Garmaroudi and B. M. McManus, Circ. Res., 2012, 110, 126–144 CrossRef CAS PubMed.
Footnote |
† Electronic supplementary information (ESI) available. See DOI: 10.1039/c6ra06858h |
|
This journal is © The Royal Society of Chemistry 2016 |
Click here to see how this site uses Cookies. View our privacy policy here.