DOI:
10.1039/C6RA07273A
(Paper)
RSC Adv., 2016,
6, 43345-43355
Synthesis of benzimidazole-based 1,3,4-oxadiazole-1,2,3-triazole conjugates as glycogen synthase kinase-3β inhibitors with antidepressant activity in in vivo models†
Received
19th March 2016
, Accepted 27th April 2016
First published on 28th April 2016
Abstract
A series of novel benzimidazole-based 1,3,4-oxadiazole-1,2,3-triazole conjugates has been synthesized and evaluated for GSK-3β inhibitory activity in vitro. Compounds 7q, 7b, 7e and 7n exhibited significant inhibition with sub-micromolar IC50 values (0.15, 0.27, 0.32 and 0.39 μM respectively) and were examined further for antidepressant activity by forced swim test (FST) and tail suspension test (TST) models in Wistar rats. In both TST and FST models, all the test compounds were found to significantly reduce the immobility time period (displaying antidepressant-like activity) in comparison to a normal saline treated control group. Compound 7q was found to be the most active. Molecular docking studies of the active compounds 7q, 7b, 7e and 7n were also performed against GSK-3β to gain an understanding of their binding interactions.
1. Introduction
Glycogen synthase kinase-3 (GSK-3) is a cytosolic, constitutively active, multi-tasking serine/threonine kinase and has generated great interest in the arena of medicinal chemistry. GSK-3 is present in two major isoforms, GSK-3α and GSK-3β, ubiquitously expressed in all tissues, with GSK-3β expressed more widely throughout the animal kingdom than GSK-3α.1–3 Due to the regulatory role of GSK-3 over a broad range of substrates,4,5 GSK-3 enjoins a central position in modulating a diverse range of key physiological processes like metabolism, signaling pathways, apoptosis, and development.6 Consequently the abnormal regulation of GSK-3 results in the development of psychiatric disorders like bipolar disorder, mood disorders, depression and schizophrenia,7,8 besides other non-psychiatric diseases such as cancer, aging immune disorders and metabolic disorders.9 In particular, a number of single nucleotide polymorphisms (SNPs) in the GSK3B locus or in genes involved in GSK-3β signaling have been identified as the genetic risk factors for bipolar disorder and schizophrenia.5 Deregulated GSK-3β has been found to be involved in depression. Lithium, a well-known GSK-3β inhibitor, used as a mood stabilizer in bipolar disorder as well as adjunct therapy for depression and schizophrenia for more than five decades has been proposed to exert its therapeutic action by GSK-3β inhibition.8,10 GSK-3β inhibitors have been reported to exhibit antidepressant-like effects in rodent models. The substrate-competitive peptide inhibitor L803-mts displayed antidepressant effect in the mouse forced swim test (FST),11 while AR-A014418 has shown similar effects in rats.12 Thiadiazolidinone derivative NP031115 also exhibited antidepressant activity in the mouse FST after systemic administration.13 In addition, classical antidepressants, as well as atypical antipsychotics involve inhibition of GSK3β, either directly or following its phosphorylation on Ser9 by AKT in their mechanism of action.14,15 Similarly, the NMDA receptor-blocker ketamine also involves in vivo GSK-3β inhibition, an effect proposed with its action as a rapid-acting antidepressant.16 Furthermore, the pharmacological or genetic inhibition of GSK-3β has been found to replicate the behavioral effects of these drugs in rodents.17 For example, the reduced GSK3β level in heterozygote GSK3β± knockout mice was found to be sufficient to reduce depression-like immobility in FST18 and the TST10 and reduce immobility in the TST in tryptophan hydroxylase 2 mutant mice.19 Given the potential role of GSK-3β in depression, pharmacological inhibition of GSK-3β represents an important therapeutic strategy.
Various GSK-3 inhibitors have been reported from diverse chemical families such as maleimides, indirubins, paullones, manzamines, metals, thiadiazolidinones, L803-mts, ureas, triazinones.20,21 Benzimidazole, oxadiazole, and triazole pharmacophores have also been identified as strong inhibitors of GSK-3β.22–32 These heterocycles constitute indispensible construction motifs for the development of compounds of medicinal interest due to their diverse pharmaceutical applications.33–35 Also, the various structural combinations of these heterocyclic cores in a single molecular framework as symmetrical or unsymmetrical bis, tris, or multiple heterocycles have resulted in improved GSK-3β inhibitory potential and alleviation of associated disorders. Keeping in view the pharmacological importance of benzimidazoles, oxadiazoles and triazoles as GSK-3β inhibitors, we aimed at the conjugation of these bioactive pharmacophores in one construct as unsymmetrical tris heterocycles (Fig. 1). The target compounds have been synthesized in sequential multi step reactions. All the synthesized conjugates (7a–7t) were evaluated for their in vitro GSK-3β inhibitory activity. Given the promising potential of GSK-3β inhibitors for treatment of depression and associated disorders, the compounds exhibiting pronounced GSK-3β inhibitory activity have been further investigated for antidepressant activity by well-known forced swim test (FST) and tail suspension test (TST) models. Docking studies of the active compounds have been carried out against GSK-3β to understand the possible modes of molecular interactions.
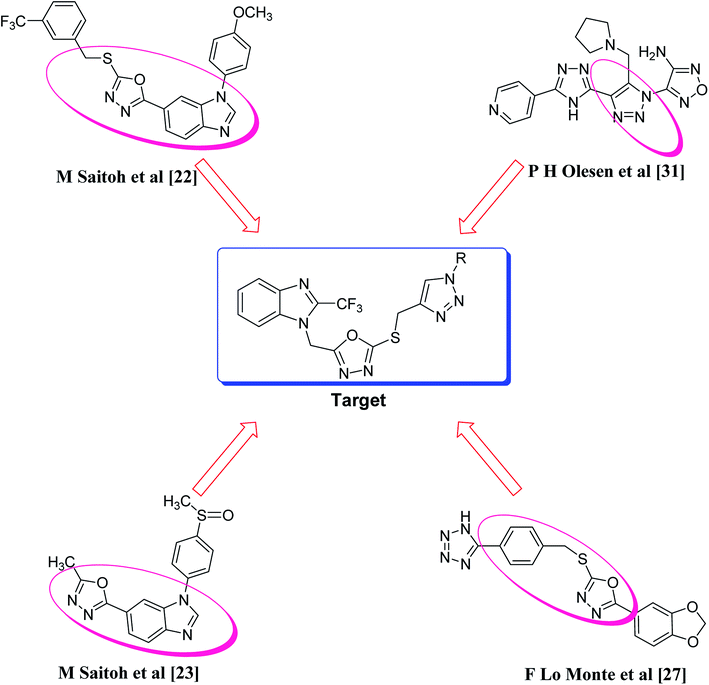 |
| Fig. 1 Conjugation of GSK-3β inhibitory pharmacophores. | |
2. Experimental
2.1. General
The chemicals and reagents used in this study were purchased from Sigma Aldrich, Merck (India) and Spectrochem. Melting points were measured using Electro-thermal IA 9100 apparatus (Shimadzu, Japan) and are uncorrected; IR spectra were recorded on a Bruker spectrophotometer (USA), NMR spectra were determined on a Bruker (300 and 400 MHz) spectrometer in CDCl3/DMSO-d6 and the chemical shifts were expressed as ppm against TMS as internal reference. Mass spectra have been recorded on 70 eV (EI Ms-QP 1000EX, Shimadzu, Japan) or Waters UPLC-TQD (ESI-MS) and column chromatography was performed on (Merck) Silica gel 60 (particle size 0.06–0.20 mm). Elemental analysis was done using Elemental Vario EL III elemental analyzer and the data is reported in % standard. All the compounds synthesized in this study are novel and were confirmed from spectral data.
2.2. Chemistry
2.2.1. Procedure for the synthesis of 2-(trifluoromethyl)-1H-benzo[d]imidazole (2). o-Phenylenediamine (5.4 g, 50 mmol) was treated with 5 ml of trifluoroacetic acid (TFA) and stirred with heating at 60 °C for 5–6 h. The reaction mixture was then cooled and poured on ice cold water to obtain a precipitate which was filtered and air dried. This compound was then used as such for the next step.
2.2.2. Procedure for the synthesis of ethyl 2-(2-(trifluoromethyl)-1H-benzo[d]imidazol-1-yl)acetate (3). 2-Triflouromethylbenzimidazole (5 g, 26.8 mmol) was added to a mixture of potassium carbonate in dry acetone and stirred for 30 minutes. This mixture was then treated with 1.5 equivalents of ethylbromoacetate and refluxed with stirring for 4–5 h with continuous monitoring of TLC. The reaction mixture was then cooled and filtered. The filtrate was concentrated and poured on ice-cold water to obtain a white precipitate. The compound was filtered, air-dried and used for the next step.
2.2.3. Procedure for the synthesis of 2-(2-(trifluoromethyl)-1H-benzo[d]imidazol-1-yl)acetohydrazide (4). To a mixture of benzimidazole ester (4.5 g, 16.5 mmol) in absolute ethanol, was added 1.5 equivalents of hydrazine hydrate (99%) and refluxed with stirring for 3 hours. The reaction mixture was concentrated and cooled. The solid obtained was filtered and dried. The compound was then re-crystallized in ethanol.
2.2.4. Procedure for the synthesis of 5-((2-(trifluoromethyl)-1H-benzo[d]imidazol-1-yl)methyl)-1,3,4-oxadiazole-2-thiol (5). A mixture of carbon disulphide (1.41 g, 18.6 mmol) and potassium hydroxide (1.04 g, 18.6 mmol) was taken in absolute ethanol and stirred at room temperature. After 30 minutes, the intermediate benzimidazole hydrazide (4 g, 15.5 mmol) was added and the reaction mixture was then refluxed with stirring for 10 h. After completion of reaction as observed from TLC, the reaction mixture was concentrated, cooled and to it were added slowly a dilute HCl solution (5 N). The colorless precipitate obtained was filtered and air dried for further use.
2.2.5. Procedure for the synthesis of 2-(trifluoromethyl)-1-((5-(prop-2-ynylthio)-1,3,4-oxadiazol-2-yl)methyl)-1H-benzo[d]imidazole (6). The oxadiazole-2-thiol intermediate (3.4 g, 11.3 mmol) was added to a mixture of potassium carbonate in dry acetone and heated to reflux with stirring. After 30 minutes, propargyl bromide (1.06 ml, 17 mmol) was added to the reaction mixture and the heating was continued with stirring for 4 h. The reaction mixture was filtered and the filtrate concentrated, cooled and poured on ice-cold water. The solid obtained was filtered and air-dried. It was purified by column chromatography by packing on a silica gel column and eluting with 5% ethyl acetate in hexane.
2.2.6. General procedure for the synthesis of benzimidazole substituted oxadiazole-based 1,2,3-triazoles (7a–7t). The propargylated intermediate (0.2 g, 0.6 mmol) was dissolved in 20 ml of t-butanol
:
water (1
:
1) solvent at ambient temperature. CuSO4·5H2O was added to it and the reaction mixture was stirred for 5 min. Sodium ascorbate was then added to the reaction mixture and stirred for 15 minutes. Azide was then added at once and the reaction mixture was continuously stirred for further 7–10 h at ambient temperature. After the completion of the reaction, monitored by TLC, the reaction mixture was quenched with water and extracted with chloroform. Organic layers were combined and dried over anhydrous sodium sulfate, filtered and then concentrated. The crude products were packed onto a silica gel column and eluted with 30–50% ethyl acetate in hexane to obtain the final purified product.
2.2.6.1. 1-((5-((1-(4-Fluorophenyl)-1H-1,2,3-triazol-4-yl)methylthio)-1,3,4-oxadiazol-2-yl)methyl)-2-(trifluoromethyl)-1H-benzo[d]imidazole (7a). Colorless powder; yield: 83%; mp 153–154 °C; IR (KBr): ν (cm−1) 3162, 3068, 2942, 1589, 1530, 1468, 1421, 1280, 1251, 1198, 1159, 1119, 1053; 1H NMR (300 MHz, CDCl3): δ 4.47 (s, 2H), 5.57 (s, 2H), 7.07–7.15 (m, 2H), 7.31–7.39 (m, 2H), 7.47–7.56 (m, 3H), 7.79 (d, 1H, J = 7.8 Hz), 7.95 (s, 1H); 13C NMR (100 MHz, CDCl3): δ 26.80, 39.01, 110.50, 116.76 (d, J = 23.0 Hz), 118.81 (q, J = 270 Hz), 121.68, 122.02, 122.56 (d, J = 9.0 Hz), 124.54, 126.38, 132.99, 134.97, 140.07 (q, J = 39.0 Hz), 140.97, 143.26, 161.27, 162.58 (d, J = 234.0 Hz), 166.20; LC-MS: 476 (M + 1)+; anal. calcd for C20H13F4N7OS: C, 50.53; H, 2.76; F, 15.98; N, 20.62; O, 3.37; S, 6.74%; found: C, 50.48; H, 2.73; N, 20.66, S, 6.75%.
2.2.6.2. 1-((5-((1-(2-Fluorophenyl)-1H-1,2,3-triazol-4-yl)methylthio)-1,3,4-oxadiazol-2-yl)methyl)-2-(trifluoromethyl)-1H-benzo[d]imidazole (7b). Colorless powder; yield: 83%; mp 129–130 °C; IR (KBr): ν (cm−1) 3152, 3073, 2920, 1588, 1530, 1504, 1468, 1417, 1267, 1190, 1099; 1H NMR (300 MHz, CDCl3): δ 4.62 (s, 2H), 5.70 (s, 2H), 7.31–7.35 (m, 2H), 7.40–7.51 (m, 3H), 7.61 (d, 1H, J = 7.5 Hz), 7.87–7.92 (m, 2H), 8.18 (s, 1H); 13C NMR (100 MHz, CDCl3): δ 26.85, 39.06, 110.53, 117.06 (d, J = 20.0 Hz), 118.83 (q, J = 268 Hz), 122.00, 124.48 (d, J = 6.0 Hz), 124.92 (d, J = 8.0 Hz), 125.28, 126.37, 130.48, 134.98, 140.08 (q, J = 39.0 Hz), 140.99, 142.70, 153.35 (d, J = 250.0 Hz), 161.33, 166.06; ESI-MS: 476 (M + 1)+; anal. calcd for C20H13F4N7OS: C, 50.53; H, 2.76; F, 15.98; N, 20.62; O, 3.37; S, 6.74%; found: C, 50.56; H, 2.72; N, 20.59; S, 6.75%.
2.2.6.3. 1-((5-((1-(2-Chlorophenyl)-1H-1,2,3-triazol-4-yl)methylthio)-1,3,4-oxadiazol-2-yl)methyl)-2-(trifluoromethyl)-1H-benzo[d]imidazole (7c). Creamish yellow powder; yield: 86%; mp 112–113 °C; IR (KBr): ν (cm−1) 3068, 2990, 2939, 1593, 1535, 1491, 1464, 1422, 1285, 1250, 1200, 1121, 1036; 1H NMR (300 MHz, CDCl3): δ 4.62 (s, 2H), 5.70 (s, 2H), 7.40–7.51 (m, 4H), 7.57–7.61 (m, 3H), 7.91 (d, 1H, J = 7.8 Hz), 8.11 (s, 1H); 13C NMR (100 MHz, CDCl3): δ 26.84, 39.06, 110.52, 118.82 (q, J = 270.0 Hz), 121.98, 124.50, 125.41, 126.37, 126.67, 127.94, 128.52, 130.80, 130.92, 134.57, 134.99, 140.07 (q, J = 39.0 Hz), 140.97, 142.06, 161.35, 166.05; ESI-MS: 492 (M + 1)+; anal. calcd for C20H13ClF3N7OS: C, 48.84; H, 2.66; Cl, 7.21; F, 11.59; N, 19.93; O, 3.25; S, 6.52%; found: C, 48.81; H, 2.64; N, 19.89; S, 6.49%.
2.2.6.4. 1-((5-((1-(3-Chlorophenyl)-1H-1,2,3-triazol-4-yl)methylthio)-1,3,4-oxadiazol-2-yl)methyl)-2-(trifluoromethyl)-1H-benzo[d]imidazole (7d). Colorless powder; yield: 84%; mp 120–121 °C; IR (KBr): ν (cm−1) 3142, 3098, 2941, 1594, 1537, 1468, 1421, 1280, 1251, 1198, 1159, 1119, 1053; 1H NMR (300 MHz, CDCl3): δ 4.60 (s, 2H), 5.70 (s, 2H), 7.41–7.52 (m, 4H), 7.58–7.62 (m, 2H), 7.74 (s, 1H), 7.92 (d, 1H, J = 7.8 Hz), 8.12 (s, 1H); 13C NMR (100 MHz, CDCl3): δ 26.76, 39.03, 110.49, 118.49, 118.82 (q, J = 270.0 Hz), 120.79, 121.42, 122.02, 124.54, 126.38, 129.03, 130.85, 134.97, 135.62, 137.55, 140.07 (q, J = 39.0 Hz), 140.98, 143.43, 161.43, 166.14; ESI-MS: 492 (M + 1)+; anal. calcd for C20H13ClF3N7OS: C, 48.84; H, 2.66; Cl, 7.21; F, 11.59; N, 19.93; O, 3.25; S, 6.52%; found: C, 48.81; H, 2.67; N, 19.91; S, 6.51%.
2.2.6.5. 1-((5-((1-Phenyl-1H-1,2,3-triazol-4-yl)methylthio)-1,3,4-oxadiazol-2-yl)methyl)-2-(trifluoromethyl)-1H-benzo[d]imidazole (7e). Colorless powder; yield: 82%; mp 161–163 °C; IR (KBr): ν (cm−1) 3126, 3098, 2962, 2917, 1593, 1530, 1502, 1459, 1420, 1266, 1193, 1115, 1095, 1052; 1H NMR (300 MHz, CDCl3): δ 4.52 (s, 2H), 5.61 (s, 2H), 7.19 (s, 1H), 7.11–7.46 (m, 4H), 7.50–7.53 (m, 1H), 7.59 (d, 2H, J = 7.5 Hz), 7.82 (d, 1H, J = 7.5 Hz), 8.02 (s, 1H); 13C NMR (100 MHz, CDCl3): δ 26.88, 39.04, 110.52, 118.82 (q, J = 269.0 Hz), 120.60, 122.02, 124.54, 126.39, 129.00, 129.80, 134.98, 136.82, 137.67, 140.07 (q, J = 39.0 Hz), 140.98, 142.89, 161.36, 164.59; ESI-MS: 458 (M + 1)+; anal. calcd for C20H14F3N7OS: C, 52.51; H, 3.08; F, 12.46; N, 21.43; O, 3.50; S, 7.01%; found: C, 52.55; H, 3.03; N, 21.41; S, 7.03%.
2.2.6.6. 1-((5-((1-(2-Bromophenyl)-1H-1,2,3-triazol-4-yl)methylthio)-1,3,4-oxadiazol-2-yl)methyl)-2-(trifluoromethyl)-1H-benzo[d]imidazole (7f). Colorless powder; yield: 86%; mp 134–136 °C; IR (KBr): ν (cm−1) 3203, 2992, 2938, 1592, 1536, 1487, 1465, 1422, 1287, 1250, 1171, 1152, 1122, 1038; 1H NMR (300 MHz, CDCl3): δ 4.62 (s, 2H), 5.69 (s, 2H), 7.37–7.51 (m, 5H), 7.59 (d, 1H, J = 7.8 Hz), 7.75 (d, 1H, J = 7.5 Hz), 7.90 (d, 1H, J = 7.5 Hz), 8.06 (s, 1H); 13C NMR (100 MHz, CDCl3): δ 26.83, 39.07, 110.51, 118.45, 118.81 (q, J = 270.0 Hz), 122.02, 124.54, 125.53, 126.40, 128.12, 128.53, 131.33, 133.96, 134.96, 136.21, 140.07 (q, J = 39.0 Hz), 140.97, 141.99, 161.33, 166.10; ESI-MS: 536 (M)+, 538 (M + 2)+; anal. calcd for C20H13BrF3N7OS: C, 44.79; H, 2.44; Br, 14.90; F, 10.63; N, 18.28; O, 2.98; S, 5.98%; found: C, 44.85; H, 2.45; N, 18.28; S, 5.99%.
2.2.6.7. 1-((5-((1-(2-Chloropyridin-3-yl)-1H-1,2,3-triazol-4-yl)methylthio)-1,3,4-oxadiazol-2-yl)methyl)-2-(trifluoromethyl)-1H-benzo[d]imidazole (7g). Colorless powder; yield: 84%; mp 137–138 °C; IR (KBr): ν (cm−1) 3162, 3068, 2987, 2939, 1589, 1571, 1535, 1478, 1417, 1287, 1253, 1199, 1175, 1118, 1099, 1036; 1H NMR (300 MHz, CDCl3): δ 4.62 (s, 2H), 5.70 (s, 2H), 7.40–7.50 (m, 3H), 7.60 (d, 1H, J = 7.8 Hz), 7.90 (d, 1H, J = 7.5 Hz), 7.97–8.00 (m, 1H), 8.25 (s, 1H), 8.56–8.58 (m, 1H); 13C NMR (100 MHz, CDCl3): δ 26.66, 39.05, 110.49, 118.81 (q, J = 268.0 Hz), 122.03, 123.41, 124.56, 125.25, 126.42, 131.81, 134.95, 135.90, 140.09 (q, J = 39.0 Hz), 140.97, 142.70, 144.89, 150.40, 161.39, 165.98; ESI-MS: 493 (M + 1)+; anal. calcd for C19H12ClF3N8OS: C, 46.30; H, 2.45; Cl, 7.19; F, 11.56; N, 22.74; O, 3.25; S, 6.51%; found: C, 46.34; H, 2.46; N, 22.72; S, 6.52%.
2.2.6.8. 1-((5-((1-(4-Methoxyphenyl)-1H-1,2,3-triazol-4-yl)methylthio)-1,3,4-oxadiazol-2-yl)methyl)-2-(trifluoromethyl)-1H-benzo[d]imidazole (7h). Colorless powder; yield: 88%; mp 135–136 °C; IR (KBr): ν (cm−1) 3175, 3058, 2968, 2943, 1613, 1589, 1535, 1517, 1467, 1421, 1282, 1252, 1199, 1174, 1109, 1053; 1H NMR (300 MHz, CDCl3): δ 3.87 (s, 3H), 4.58 (s, 2H), 5.69 (s, 2H), 7.00 (d, 2H, J = 8.7 Hz), 7.39–7.49 (m, 2H), 7.54–7.61 (m, 3H), 7.90 (d, 1H, J = 7.8 Hz), 8.01 (s, 1H); 13C NMR (100 MHz, CDCl3): δ 26.90, 39.03, 55.66, 110.54, 114.75, 118.81 (q, J = 270.0 Hz), 121.65, 122.00, 122.20, 124.53, 126.39, 130.15, 134.97, 140.07 (q, J = 38.0 Hz), 140.95, 142.84, 159.93, 161.36, 166.26; LC-MS: 488 (M + 1)+; anal. calcd for C21H16F3N7O2S: C, 51.74; H, 3.31; F, 11.69; N, 20.11; O, 6.56; S, 6.58%; found: C, 51.72; H, 3.34; N, 20.07; S, 6.55%.
2.2.6.9. 1-((5-((1-(3,4-Dichlorophenyl)-1H-1,2,3-triazol-4-yl)methylthio)-1,3,4-oxadiazol-2-yl)methyl)-2-(trifluoromethyl)-1H-benzo[d]imidazole (7i). Creamish powder; yield: 82%; mp 131–132 °C; IR (KBr): ν (cm−1) 3152, 3058, 2939, 1587, 1530, 1467, 1418, 1267, 1198, 1167, 1118, 1035; 1H NMR (400 MHz, CDCl3): δ 4.54 (s, 2H), 5.68 (s, 2H), 7.37–7.46 (m, 2H), 7.50–7.58 (m, 3H), 7.81 (d, 1H, J = 2.0 Hz), 7.86 (d, 1H, J = 8.4 Hz), 8.09 (s, 1H); 13C NMR (100 MHz, CDCl3): δ 26.69, 39.00, 110.48, 118.81 (q, J = 270.0 Hz), 119.41, 121.36, 121.99, 122.23, 124.53, 126.36, 131.46, 133.07, 134.00, 134.97, 135.70, 140.05 (q, J = 39.0 Hz), 140.96, 143.66, 161.48, 166.06; LC-MS: 528 (M + 3)+; anal. calcd for C20H12Cl2F3N7OS: C, 45.64; H, 2.30; Cl, 13.47; F, 10.83; N, 18.63; O, 3.04; S, 6.09%; found: C, 45.67; H, 2.33; N, 18.59; S, 6.06%.
2.2.6.10. 1-((5-((1-(2,4-Dichlorophenyl)-1H-1,2,3-triazol-4-yl)methylthio)-1,3,4-oxadiazol-2-yl)methyl)-2-(trifluoromethyl)-1H-benzo[d]imidazole (7j). Creamish powder; yield: 81%; mp 137–138 °C; IR (KBr): ν (cm−1) 3154, 3066, 2914, 1592, 1528, 1473, 1419, 1263, 1195, 1167, 1113, 1043; 1H NMR (400 MHz, CDCl3): δ 4.57 (s, 2H), 5.68 (s, 2H), 7.40–7.49 (m, 2H), 7.52–7.60 (m, 3H), 7.84 (d, 1H, J = 2.4 Hz), 7.90 (d, 1H, J = 8.0 Hz), 8.01 (s, 1H); 13C NMR (100 MHz, CDCl3): δ 26.74, 39.05, 110.50, 118.81 (q, J = 271.0 Hz), 122.02, 122.91, 124.53, 125.49, 126.39, 128.33, 128.39, 129.25, 130.64, 133.24, 134.96, 136.41, 140.09 (q, J = 39.0 Hz), 140.97, 161.43, 166.10; LC-MS: 528 (M + 3)+; anal. calcd for C20H12Cl2F3N7OS: C, 45.64; H, 2.30; Cl, 13.47; F, 10.83; N, 18.63; O, 3.04; S, 6.09%; found: C, 45.59; H, 2.33; N, 18.61; S, 6.11%.
2.2.6.11. 1-((5-((1-(2-Methoxyphenyl)-1H-1,2,3-triazol-4-yl)methylthio)-1,3,4-oxadiazol-2-yl)methyl)-2-(trifluoromethyl)-1H-benzo[d]imidazole (7k). Colorless powder; yield: 88%; mp 108–109 °C; IR (KBr): ν (cm−1) 3157, 2961, 1586, 1526, 1463, 1391, 1265, 1246, 1178, 1139, 1122, 1090, 1046; 1H NMR (400 MHz, CDCl3): δ 3.83 (s, 3H), 4.60 (s, 2H), 5.67 (s, 2H), 7.05–7.10 (m, 2H), 7.38–7.47 (m, 3H), 7.58 (d, 1H, J = 8.0 Hz), 7.70–7.72 (m, 1H), 7.88 (d, 1H, J = 8.0 Hz), 8.19 (s, 1H); 13C NMR (100 MHz, CDCl3): δ 27.03, 39.05, 55.88, 110.56, 112.19, 118.83 (q, J = 270.0 Hz), 121.14, 121.91, 124.46, 125.27, 125.46, 125.94, 126.31, 130.25, 135.00, 140.05 (q, J = 39.0 Hz), 140.95, 141.45, 150.96, 161.31, 166.08; ESI-MS: 488 (M + 1)+; anal. calcd for C21H16F3N7O2S: C, 51.74; H, 3.31; F, 11.69; N, 20.11; O, 6.56; S, 6.58%; found: C, 51.69; H, 3.34; N, 20.07; S, 6.61%.
2.2.6.12. 1-((5-((1-(4-tert-Butylphenyl)-1H-1,2,3-triazol-4-yl)methylthio)-1,3,4-oxadiazol-2-yl)methyl)-2-(trifluoromethyl)-1H-benzo[d]imidazole (7l). Colorless powder; yield: 82%; mp 104–105 °C; IR (KBr): ν (cm−1) 3152, 2942, 1587, 1525, 1473, 1419, 1279, 1195, 1153, 1113, 1098, 1047; 1H NMR (400 MHz, DMSO-d6): δ 1.33 (s, 9H), 4.62 (s, 2H), 6.04 (s, 2H), 7.40–7.50 (m, 2H), 7.60 (d, 2H, J = 8.8 Hz), 7.74 (d, 2H, J = 8.8 Hz), 7.81–7.87 (m, 2H), 8.64 (s, 1H); 13C NMR (100 MHz, CDCl3): δ 26.93, 31.26, 34.81, 39.03, 110.51, 118.82 (q, J = 270.0 Hz), 120.29, 121.47, 122.03, 124.53, 126.39, 126.65, 134.34, 134.99, 140.09 (q, J = 39.0 Hz), 140.99, 142.88, 152.38, 161.34, 166.27; LC-MS: 514 (M + 1)+; anal. calcd for C24H22F3N7OS: C, 56.13; H, 4.32; F, 11.10; N, 19.09; O, 3.12; S, 6.24%; found: C, 56.09; H, 4.36; N, 19.12; S, 6.21%.
2.2.6.13. 1-((5-((1-o-Tolyl-1H-1,2,3-triazol-4-yl)methylthio)-1,3,4-oxadiazol-2-yl)methyl)-2-(trifluoromethyl)-1H-benzo[d]imidazole (7m). Colorless powder; yield: 83%; mp 107–108 °C; IR (KBr): ν (cm−1) 3155, 2942, 1584, 1527, 1493, 1420, 1273, 1190, 1156, 1118, 1099, 1049; 1H NMR (400 MHz, CDCl3): δ 2.87 (s, 3H), 4.61 (s, 2H), 5.68 (s, 2H), 7.27–7.36 (m, 3H), 7.39–7.48 (m, 3H), 7.58 (d, 1H, J = 8.0 Hz), 7.84 (s, 1H), 7.89 (d, 1H, J = 8.0 Hz); 13C NMR (100 MHz, CDCl3): δ 17.82, 26.92, 39.05, 110.49, 118.87 (q, J = 269.0 Hz), 122.02, 124.52, 124.84, 125.90, 126.37, 126.88, 129.99, 131.52, 133.51, 134.98, 136.17, 140.10 (q, J = 39.0 Hz), 140.99, 142.12, 161.34, 166.22; ESI-MS: 472 (M + 1)+; anal. calcd for C21H16F3N7OS: C, 53.50; H, 3.42; F, 12.09; N, 20.80; O, 3.39; S, 6.80%; found: C, 53.54; H, 3.45; N, 20.74; S, 6.83%.
2.2.6.14. 1-((5-((1-(4-Ethylphenyl)-1H-1,2,3-triazol-4-yl)methylthio)-1,3,4-oxadiazol-2-yl)methyl)-2-(trifluoromethyl)-1H-benzo[d]imidazole (7n). Colorless powder; yield: 87%; mp 135–136 °C; IR (KBr): ν (cm−1) 3057, 2982, 1591, 1572, 1519, 1465, 1413, 1278, 1193, 1174, 1149, 1096, 1045; 1H NMR (400 MHz, CDCl3): δ 1.27 (t, 3H, J = 7.6 Hz), 2.71 (q, 2H, J = 7.6 Hz), 4.58 (s, 2H), 5.67 (s, 2H), 7.31 (d, 2H, J = 8.4 Hz), 7.39–7.48 (m, 2H), 7.55–7.59 (m, 3H), 7.89 (d, 1H, J = 8.0 Hz), 8.04 (s, 1H); 13C NMR (100 MHz, CDCl3): δ 15.47, 26.90, 28.48, 39.05, 110.53, 118.82 (q, J = 270.0 Hz), 120.59, 121.51, 122.01, 124.53, 126.39, 129.11, 134.59, 134.98, 140.09 (q, J = 39.0 Hz), 140.97, 142.89, 145.45, 161.36, 166.25; LC-MS: 486 (M + 1)+; anal. calcd for C22H18F3N7OS: C, 54.43; H, 3.74; F, 11.74; N, 20.20; O, 3.30; S, 6.60%; found: C, 54.47; H, 3.71; N, 20.17; S, 6.62%.
2.2.6.15. 1-((5-((1-(4-Chlorophenyl)-1H-1,2,3-triazol-4-yl)methylthio)-1,3,4-oxadiazol-2-yl)methyl)-2-(trifluoromethyl)-1H-benzo[d]imidazole (7o). Creamish powder; yield: 85%; mp 157–159 °C; IR (KBr): ν (cm−1) 3153, 3072, 2942, 1589, 1530, 1501, 1475, 1454, 1415, 1267, 1192, 1165, 1116, 1093, 1047; 1H NMR (400 MHz, CDCl3): δ 4.59 (s, 2H), 5.67 (s, 2H), 7.39–7.52 (m, 5H), 7.56–7.58 (m, 2H), 7.89 (d, 1H, J = 8.0 Hz), 8.08 (s, 1H); 13C NMR (100 MHz, CDCl3): δ 26.77, 39.03, 110.49, 118.85 (q, J = 268.0 Hz), 120.59, 121.71, 122.04, 124.56, 126.39, 129.97, 134.77, 134.96, 135.22, 139.24, 140.10 (q, J = 38.0 Hz), 140.98, 161.41, 166.59; LC-MS: 492 (M + 1)+; anal. calcd for C20H13ClF3N7OS: C, 48.84; H, 2.66; Cl, 7.21; F, 11.59; N, 19.93; O, 3.25; S, 6.52%; found: C, 48.79; H, 2.64; N, 19.97; S, 6.53%.
2.2.6.16. 1-((5-((1-(3,4-Dimethylphenyl)-1H-1,2,3-triazol-4-yl)methylthio)-1,3,4-oxadiazol-2-yl)methyl)-2-(trifluoromethyl)-1H-benzo[d]imidazole (7p). Colorless powder; yield: 84%; mp 134–136 °C; IR (KBr): ν (cm−1) 3162, 3055, 2984, 2939, 1586, 1525, 1461, 1420, 1275, 1247, 1197, 1147, 1114, 1098, 1046; 1H NMR (400 MHz, CDCl3): δ 2.31 (s, 3H), 2.33 (s, 3H), 4.58 (s, 2H), 5.68 (s, 2H), 7.27–7.24 (m, 1H), 7.34–7.36 (m, 1H), 7.39–7.48 (m, 3H), 7.59 (d, 1H, J = 8.0 Hz), 7.89 (d, 1H, J = 8.0 Hz), 8.04 (s, 1H); 13C NMR (100 MHz, CDCl3): δ 19.51, 19.91, 26.92, 39.05, 110.53, 117.84, 118.82 (q, J = 270.0 Hz), 121.51, 121.66, 122.00, 124.52, 126.38, 130.63, 134.63, 134.98, 137.79, 138.42, 140.07 (q, J = 39.0 Hz), 140.97, 142.80, 161.35, 166.27; LC-MS: 486 (M + 1)+; anal. calcd for C22H18F3N7OS: C, 54.43; H, 3.74; F, 11.74; N, 20.20; O, 3.30; S, 6.60%; found: C, 54.45; H, 3.73; N, 20.25; S, 6.57%.
2.2.6.17. 1-((5-((1-(Pyridin-3-yl)-1H-1,2,3-triazol-4-yl)methylthio)-1,3,4-oxadiazol-2-yl)methyl)-2-(trifluoromethyl)-1H-benzo[d]imidazole (7q). Colorless powder; yield: 89%; mp 135–136 °C; IR (KBr): ν (cm−1) 3158, 3052, 2967, 2941, 1594, 1537, 1473, 1418, 1279, 1253, 1198, 1175, 1113, 1090, 1047; 1H NMR (400 MHz, DMSO-d6): δ 4.64 (s, 2H), 6.03 (s, 2H), 7.37–7.41 (m, 1H), 7.45–7.49 (m, 1H), 7.63 (dd, 1H, J = 8.4 Hz and 4.8 Hz), 7.80–7.84 (m, 2H), 8.26 (dd, 1H, J = 8.4 Hz and 1.2 Hz), 8.69 (d, 1H, J = 4.4 Hz), 8.79 (s, 1H), 9.08 (s, 1H); 13C NMR (100 MHz, DMSO-d6): δ 26.86, 111.88, 118.81 (q, J = 270.0 Hz), 121.25, 122.65, 124.86, 125.30, 126.62, 128.72, 133.44, 135.44, 139.76 (q, J = 38.0 Hz), 140.31, 141.26, 144.14, 150.10, 163.07, 164.81; ESI-MS: 459 (M + 1)+; anal. calcd for C19H13F3N8OS: C, 49.78; H, 2.86; F, 12.43; N, 24.44; O, 3.49; S, 6.99%; found: C, 49.73; H, 2.88; N, 24.46; S, 6.98%.
2.2.6.18. 1-((5-((1-(4-Bromophenyl)-1H-1,2,3-triazol-4-yl)methylthio)-1,3,4-oxadiazol-2-yl)methyl)-2-(trifluoromethyl)-1H-benzo[d]imidazole (7r). Colorless powder; yield: 85%; mp 164–166 °C; IR (KBr): ν (cm−1) 3128, 3071, 297 7, 2942, 1587, 1536, 1494, 1473, 1453, 1415, 1266, 1190, 1163, 1116, 1092, 1045; 1H NMR (300 MHz, CDCl3): δ 4.59 (s, 2H), 5.70 (s, 2H), 7.28–7.30 (m, 1H), 7.41–7.51 (m, 2H), 7.56–7.67 (m, 4H), 7.91 (d, 1H, J = 7.5 Hz), 8.10 (s, 1H); 13C NMR (100 MHz, CDCl3): δ 26.78, 39.03, 110.48, 118.81 (q, J = 271.0 Hz), 121.33, 121.92, 122.04, 122.65, 124.55, 126.39, 132.93, 134.96, 135.70, 140.08 (q, J = 39.0 Hz), 140.99, 143.43, 161.42, 166.18; ESI-MS: 536 (M)+, 538 (M + 2)+; anal. calcd for C20H13BrF3N7OS: C, 44.79; H, 2.44; Br, 14.90; F, 10.63; N, 18.28; O, 2.98; S, 5.98%; found: C, 44.74; H, 2.45; N, 18.27; S, 5.96%.
2.2.6.19. 1-((5-((1-(3-(Trifluoromethyl)phenyl)-1H-1,2,3-triazol-4-yl)methylthio)-1,3,4-oxadiazol-2-yl)methyl)-2-(trifluoromethyl)-1H-benzo[d]imidazole (7s). Colorless powder; yield: 81%; mp 141–142 °C; IR (KBr): ν (cm−1) 3162, 3055, 2987, 2942, 1590, 1527, 1479, 1421, 1267, 1200, 1117, 1097, 1036; 1H NMR (400 MHz, DMSO-d6): δ 4.65 (s, 2H), 6.04 (s, 2H), 7.38–7.42 (m, 1H), 7.46–7.50 (m, 1H), 7.81–7.89 (m, 4H), 8.20–8.23 (m, 2H), 8.90 (s, 1H); 13C NMR (100 MHz, DMSO-d6): δ 26.63, 111.79, 116.73, 118.67 (q, J = 270.0 Hz), 120.93, 122.36, 123.94, 124.04, 124.88, 125.24, 125.82, 130.49 (q, J = 32.0 Hz), 131.27, 135.31, 136.84, 139.30 (q, J = 38.0 Hz), 140.21, 143.52, 162.71, 163.97; ESI-MS: 526 (M + 1)+; anal. calcd for C21H13F6N7OS: C, 48.00; H, 2.49; F, 21.69; N, 18.66; O, 3.05; S, 6.10%; found: C, 48.05; H, 2.48; N, 18.62; S, 6.11%.
2.2.6.20. 4-(4-((5-((2-(Trifluoromethyl)-1H-benzo[d]imidazol-1-yl)methyl)-1,3,4-oxadiazol-2-ylthio)methyl)-1H-1,2,3-triazol-1-yl)benzonitrile (7t). Colorless powder; yield: 82%; mp 155–156 °C; IR (KBr): ν (cm−1) 3162, 3053, 2967, 2928, 1589, 1535, 1473, 1419, 1278, 1199, 1117, 1097, 1046; 1H NMR (400 MHz, DMSO-d6): δ 4.65 (s, 2H), 6.04 (s, 2H), 7.39–7.43 (m, 1H), 7.47–7.51 (m, 1H), 7.81–7.86 (m, 2H), 8.07–8.13 (m, 4H), 8.87 (s, 1H); 13C NMR (100 MHz, DMSO-d6): δ 26.55, 111.09, 111.79, 118.05, 118.68 (q, J = 270.0 Hz), 120.43, 120.95, 122.25, 124.07, 125.85, 134.24, 135.30, 139.28, 139.29 (q, J = 38.0 Hz), 140.21, 143.73, 162.69, 163.96; ESI-MS: 483 (M + 1)+; anal. calcd for C21H13F3N8OS: C, 52.28; H, 2.72; F, 11.81; N, 23.23; O, 3.32; S, 6.65%; found: C, 52.32; H, 2.71; N, 23.21; S, 6.63%.
2.3. Pharmacology
2.3.1. Chemicals. Human recombinant GSK-3β and pre-phosphorylated polypeptide substrate GS-2 were obtained from Merck-Millipore Corporation (India). Kinase-Glo Luminescent Kinase Assay (catalog no. V6713) was purchased from Promega Corporation (Madison, WI). Kinases CDK2/CycA2 (Cat. no. PV3267), PKA (Cat. no. P2912) and AKT1 (Cat. no. P2999) were purchased from Invitrogen. Z′-LYTE™ kinase assay kits for CDK2/CycA2 (Cat. no. PV3673), PKA (Cat. no. PV3174) and AKT1 (Cat. no. P3179) were also purchased from Invitrogen. Staurosporine was purchased from Sigma-Aldrich Chemicals Pvt. Limited, Bangalore, India. Fluoxetine was procured from the local market.
2.3.2. GSK-3β inhibitory assay. The compound library was assessed for their GSK-3β inhibition as per the Kinase-Glo assay method of Baki et al.36 This assay determines the activity of the kinase by quantifying the amount of ATP which remains in solution following a kinase reaction. In a typical assay, 10 μL of test compound of various concentrations (dissolved in dimethyl sulfoxide [DMSO] and diluted with assay buffer) and 10 μL (20 ng) of enzyme were added to each well followed by 20 μL of assay buffer containing substrate and ATP to obtain a concentration of 25 μM substrate and 1 μM ATP per well. The final DMSO concentration in the reaction mixture was kept less than 1%. After incubation at 30 °C for 30 min, the enzymatic reaction was quenched with 40 μL of Kinase-Glo reagent. Luminescence was recorded after 10 min using Infinite F200®PRO multimode reader (Tecan). The activity is proportional to the difference of the total and consumed ATP. The activity was highest in the absence of inhibitor and was used to calculate the inhibitory activities of test compounds. Staurosporine was used as a standard GSK3β inhibitor.
2.3.3. CDK2/CycA2, PKA and AKT1 inhibition assays. The kinase assays were performed using the Invitrogen Z′-LYTE™ kinase assay kits as carried out by Qing Ye et al.37 Staurosporine was taken as the standard against all the three kinases.
2.3.4. Antidepressant activity. The antidepressant activity was evaluated by the two well-accepted behavioural models – forced swim test and tail suspension test.
2.3.4.1. Animals. Wistar rats of either sex (150–180 g) were acquired from Central Animal House, Hamdard University (Jamia Hamdard), New Delhi and kept in colony cages at an ambient temperature of 25 ± 2 °C and relative humidity 45–55% with 12 h light/dark cycles after initial acclimatization for about 1 week. They were provided free access to standard rodent pellet diet and water ad libitum. The experimental study was conducted in accordance with the guidelines of the Institutional Animal Ethics Committee. The test compounds were suspended in 0.5% methylcellulose (Methocel) and administered orally 1 h before behavioural testing.
2.3.4.2. Forced swim test. The forced swim test (FST) is the most widely employed pre-clinic behavioural test for the screening of compounds for potential antidepressant activity.38 The test was performed as per the procedure described by Vincent Castagne et al.39 Rats were transferred from the housing colony room to the testing laboratory and kept undisturbed there for at least 1 h before testing. A free access to standard rodent diet and tap water was provided, except during the test. A controlled temperature of 21 ± 3 °C and standard light/dark cycle with illumination from 0700 to 1900 h were maintained. Each experimental group comprised of 6 rats. A habituation session of 15 min was conducted prior to drug administration and without behavioural recording. The rats were individually forced to swim in a plexiglas cylinders (20 cm in diameter × 40 cm high) filled with water (25 °C) to a depth of 30 cm. After 24 h, each group were administered with the appropriate dose of fluoxetine (30 mg kg−1 BW) or test compounds (20 mg kg−1 BW) or their vehicles and followed 1 h later by a 6 min test session.13,40,41 The total immobility time, defined by lack of activity, except for the necessary movements to keep their heads above water, was scored during this session. Data was compared with the data from the control and the standard group.
2.3.4.3. Tail suspension test (TST). The tail suspension test as a model of behavioural testing is related in principle to the forced swimming test, except that the immobility is induced by suspending the rat by its tail.42,43 Rats were moved to the testing laboratory from the housing colony room and kept undisturbed there for at least 1 h before testing. The dosage, temperature and other environmental conditions were kept same as in the forced swim test. The test was performed using an automated apparatus. Rats were suspended individually upside-down using an adhesive tape to fix its tail to a hook at 60 cm, which was connected to a strain gauge. A square platform was temporarily positioned 20 cm below the apparatus, just below the rat's forepaws, in order to minimize the weight sustained by the animal's tail until the start of the recording session. The movements made by the rat were picked up by the strain gauge and transmitted them to a central unit, which calculated the total duration of immobility during a 6 min test with the help of a maze software version 3.0. The rat was considered immobile when it didn't show any movements of struggling, attempting to catch the adhesive tape, body torsions, or jerks. Data collected was expressed as a mean of immobility time (in second ± the standard error mean: S.E.M).
2.4. Molecular docking studies
The in silico molecular docking studies were carried along with in vitro studies, using Schrodinger Software (Maestro Version 10). The ligands were virtually prepared by Lig-Prep module (Version 3.3). The protein was prepared by Protein Preparation Wizard through Maestro Interface (Version 10). The binding pocket was generated by selecting a grid with respect to reference ligand, 5-[1-(4-methoxyphenyl)-1H-benzimidazol-6-yl]-1,3,4-oxadiazole-2(3H)-thione. The docking was carried using Glide Module (Version 6.6) with Extra Precision (XP) mode. The docked molecules were ranked with respect to Glide score.
2.5. Statistical analysis
Results are expressed as the mean ± SEM, and different groups were compared using one way analysis of variance (ANOVA) followed by Tukey Kramer test for multiple comparisons.
3. Results and discussion
3.1. Chemistry
The synthesis of target compounds has been carried out as illustrated in Scheme 1. o-Phenylenediamine 1 was refluxed in trifluoroacetic acid (TFA) for 5–6 h to yield 2-trifluoromethyl benzimidazole 2 which was then refluxed with ethyl bromoacetate in presence of potassium carbonate in dry acetone for 4–5 h to obtain ethyl 2-(2-(trifluoromethyl)-1H-benzo[d]imidazol-1-yl)acetate 3. The intermediate 3 was subsequently treated with hydrazine hydrate in absolute ethanol to afford the 2-(2-(trifluoromethyl)-1H-benzo[d]imidazol-1-yl)acetohydrazide 4 in good yields. The resulting hydrazide intermediate 4 was refluxed with carbon disulphide in presence of potassium hydroxide in absolute ethanol for 10 h to obtain 5-((2-(trifluoromethyl)-1H-benzo[d]imidazol-1-yl)methyl)-1,3,4-oxadiazole-2-thiol 5 which was then treated with propargyl bromide in the presence of potassium carbonate in dry acetone under refluxing conditions for 4–5 h to obtain 2-(trifluoromethyl)-1-((5-(prop-2-ynylthio)-1,3,4-oxadiazol-2-yl)methyl)-1H-benzo[d]imidazole 6. The propargylated intermediate 6 was subsequently treated with various azides under click chemistry conditions to afford the target compounds, benzimidazole-based 1,3,4-oxadiazole-1,2,3-triazoles (7a–7t) in quantitative yields (Table 1). The structures of all the synthesized compounds were established by the 1H NMR, 13C NMR, IR and mass spectral techniques.
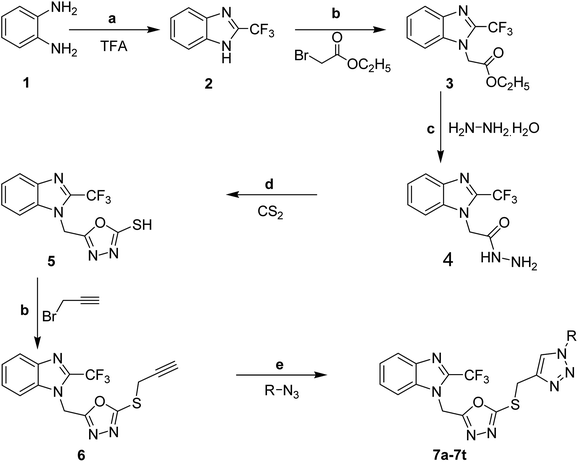 |
| Scheme 1 Synthetic approach for benzimidazole-based oxadiazole-1,2,3-triazole conjugates. (a) Reflux, 4 h, (b) acetone, K2CO3, reflix, (c) Abs. EtOH, reflux, 4 h, (d) Abs. EtOH, KOH, reflux, 12 h, (e) tert-BuOH : H2O-1 : 1,CuSO4·5H2O, Na ascorbate, RT. | |
Table 1 Benzimidazole-based oxadiazole-1,2,3-triazole conjugates and their GSK-3β inhibitory activity

|
Compound code |
R |
IC50 (μM) ± S.E. |
7a |
4-Fluoro phenyl |
0.54 ± 0.03 |
7b |
2-Fluoro phenyl |
0.27 ± 0.03 |
7c |
2-Chloro phenyl |
13.37 ± 0.89 |
7d |
3-Chloro phenyl |
6.69 ± 0.47 |
7e |
Phenyl |
0.32 ± 0.02 |
7f |
2-Bromo phenyl |
20.39 ± 1.24 |
7g |
2-Chloro-3-pyridyl |
2.84 ± 0.23 |
7h |
4-Methoxy phenyl |
0.61 ± 0.05 |
7i |
3,4-Dichloro phenyl |
1.65 ± 0.18 |
7j |
2,4-Dichloro phenyl |
9.46 ± 0.72 |
7k |
2-Methoxy phenyl |
3.28 ± 0.13 |
7l |
4-tert-Butyl phenyl |
6.64 ± 0.52 |
7m |
2-Methyl phenyl |
17.38 ± 1.06 |
7n |
4-Ethyl phenyl |
0.39 ± 0.03 |
7o |
4-Chloro phenyl |
0.64 ± 0.05 |
7p |
3,4-Dimethyl phenyl |
12.55 ± 0.92 |
7q |
3-Pyridyl |
0.15 ± 0.02 |
7r |
4-Bromo phenyl |
0.93 ± 0.08 |
7s |
3-Trifluoromethyl phenyl |
1.08 ± 0.07 |
7t |
4-Cyano phenyl |
0.59 ± 0.05 |
Staurosporine |
— |
0.05 ± 0.01 |
3.2. Biological activity
3.2.1. GSK-3β inhibitory activity. All the synthesized benzimidazole-based 1,3,4-oxadiazole-1,2,3-triazoles were assessed for their in vitro GSK-3β inhibition potential by Kinase-Glo™ luminescent method of Baki et al.36 From the in vitro assay data obtained, it was observed that some of the compounds displayed significant GSK-3β inhibition potential as illustrated in Table 1. Conjugates 7q, 7b, 7e and 7n were found to inhibit significantly with IC50 values of 0.15 μM, 0.27 μM, 0.32 μM and 0.39 μM respectively in comparison to Staurosporine exhibiting IC50 value of 0.05 μM. Compounds 7a, 7t, 7h and 7o also showed a strong inhibition of GSK-3β with respective IC50 values of 0.54 μM, 0.59 μM, 0.61 μM and 0.64 μM. The rest of the compounds displayed either moderate or weak inhibition.In addition, the kinase selectivity of compounds 7q, 7b, 7e and 7n showing significant GSK-3β inhibition was also evaluated by checking their inhibitory potency against other kinases (CDK2/CycA2, PKA and AKT1). Staurosporine which is a known inhibitor of these enzymes was taken as the reference. The compounds were tested at a concentration of 2 μM and were found to show inhibition of less than 20%, indicating their good selectivity towards GSK-3β (Table 2).
Table 2 The selectivity of compounds 7q, 7b, 7e and 7n against tested kinases
Kinase assay |
Inhibition % at 2 μM |
7b |
7e |
7n |
7q |
CDK2/CycA2, PKA and AKT1 |
<20 |
<20 |
<20 |
<20 |
A preliminary structure–activity relationship was analyzed to gain an insight into the effect of different substitutions in or around the aryl ring of triazole core to the efficacy of their GSK-3β inhibition. Un-substituted phenyl conjugate (7e) was found to be more active than most of the conjugates with substitution around the aryl ring. Insertion of nitrogen atom in the aryl ring (7q) led to further increase in GSK-3β inhibition and resulted in most active compound of the series with IC50 value of 0.15 μM. However, the substitution on the pyridyl ring (7g) resulted in significant loss of activity. Among the halogenated compounds, the para-halo substituted conjugates were found to exhibit better activity when compared to the ortho-halogenated compounds except fluoro-substituted compounds. Regarding the effect of the nature of halo-group on activity, o-fluoro substituted conjugate (7b) demonstrated the best activity against GSK-3β followed by o-chloro and o-bromo substituted conjugates (7c and 7f). The same order was shown by the para-halogenated conjugates (7a > 7o > 7s) suggesting decrease in activity observed with increase in the size of halogen irrespective of the position on the aryl ring. In addition, presence of chloro at para-position conferred greater activity than m-chloro or di-chloro substituted conjugates. Among the alkyl substitutions, ethyl group (7n) at para-position imparted significant activity in comparison to bulky tert-butyl group (7l) at the same position, while among the alkoxy groups introduced, methoxy at para-position conferred increased activity than at the ortho-position. Regarding the effect of the nature of electron donating or electron withdrawing group on the aromatic ring, there wasn't any particular trend observed in regards to activity.
3.2.2. Antidepressant activity. In view of the putative role of GSK-3β in the pathophysiology of depression, and the potential therapeutic properties of GSK-3β inhibitors as antidepressants, the compounds 7q, 7b, 7e and 7n exhibiting potent GSK-3β inhibitory activity were further evaluated for antidepressant activity by the widely accepted forced swim test and tail suspension test (TST and FST) models.
3.2.2.1. Forced swim test (FST). The effect of the test compounds 7q, 7b, 7e and 7n on immobility in the forced swim test is illustrated in Fig. 2. All the test compounds significantly reduced the immobility time in experimental rats as compared to normal saline treated control group (P < 0.05). Compound 7q was found to be the most active in lowering the immobility time by 1.5-fold in comparison to normal saline group. Compounds 7b and 7e exhibited activity comparable to that of the standard fluoxetine, while as 7n was found to be slightly less active than fluoxetine.
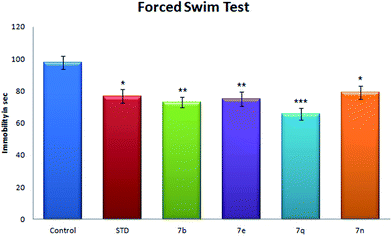 |
| Fig. 2 Forced swim test. Data is represented as mean ± SEM of 6 rats and analyzed by one way ANOVA followed by Tukey Kramer multiple comparison test, *P < 0.05, **P < 0.01, ***P < 0.001 when compared with normal control. Fluoxetine is taken as standard (STD). | |
3.2.2.2. Tail suspension test (TST). The results illustrating the effect of compounds 7q, 7b, 7e and 7n on immobility in the tail suspension test are depicted in Fig. 3. The test compounds significantly shortened the immobility time periods in experimental rats in comparison to normal saline treated control group (P < 0.05). Compound 7q was particularly most active. Pre-treatment with 7q resulted in a considerable decrease in mean duration of immobility by 1.45-fold relative to normal control group. Compounds 7q and 7b were also found to be more effective in reducing the immobility period as compared to fluoxetine. The activity of compound 7e was comparable with the fluoxetine while as that of 7n was slightly lower than fluoxetine.
 |
| Fig. 3 Tail suspension test. Data is represented as mean ± SEM of 6 rats and analyzed by one way ANOVA followed by Tukey Kramer multiple comparison test, *P < 0.05, **P < 0.01, ***P < 0.001 when compared with normal control. Fluoxetine is taken as standard (STD). | |
The compounds displaying potent GSK-3β inhibition (7q, 7b, 7e, 7n) thus exhibited the antidepressant-like effects in both forced swim test and tail suspension test (TST and FST) models, particularly compound 7q which displayed highest activity in both models. Furthermore, the antidepressant activity of the tested compounds was observed in conformity with respect to their GSK-3β inhibitory activity, leading to an impression that these compounds exert their anti-depressive behavior through GSK-3β inhibition mechanism. These results indicate that these conjugates as GSK-3β inhibitors may hold a significant potential for the treatment of depression and related disorders.
3.3. Molecular docking studies
In order to have a better comprehension of the binding pattern of synthesized molecules, molecular docking studies of the active compounds (7q, 7b, 7e and 7n) were performed against GSK-3β protein (PDB ID: 3F88). The reference ligand 5-[1-(4-methoxyphenyl)-1H-benzimidazol-6-yl]-1,3,4-oxadiazole-2(3H)-thione forms hydrogen bond with backbone atoms of VAL-135. Other residues like ILE-62, VAL-70, ALA-83 and LEU-188 form the hydrophobic pocket. As shown in Table 3, compound 7q was found to exhibit highest Glide score of −7.88 in comparison to standard with glide score of −9.01. It formed H-bond interaction with Val-135 and was also deeply buried into the hydrophobic pocket (ILE-62, VAL-70, ALA-83) forming hydrophobic interactions within the GSK-3β pocket (Fig. 4). These interactions might account for its observed GSK-3β inhibitory activity in vitro.
Table 3 Glide scores with respect to GSK-3β protein target (PDB ID: 3F88)
Compound code |
Glide score |
7q |
−7.88 |
7b |
−6.96 |
7e |
−6.82 |
7n |
−6.76 |
Standard |
−9.01 |
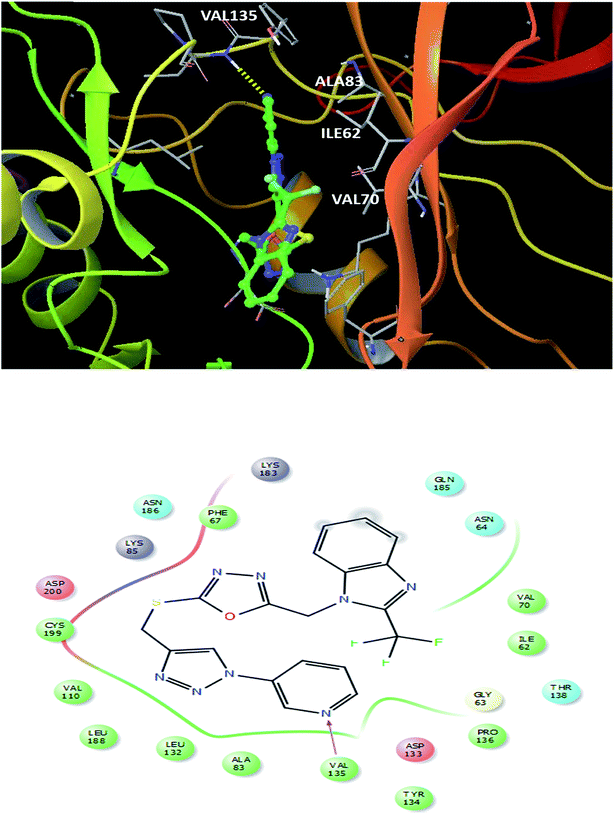 |
| Fig. 4 Docking pose and ligplot of ligand 7q with respect to GSK-3β protein (PDB ID: 3F88). | |
4. Conclusions
A focused library of twenty conjugates of benzimidazole-based 1,3,4-oxadiazole–1,2,3-triazoles scaffold has been synthesized and assessed for in vitro GSK-3β inhibitory activity. The compounds 7q, 7b, 7e and 7n exhibited significant GSK-3β inhibition with IC50 values of 0.15, 0.27, 0.32 and 0.39 μM respectively and were also found to be selective against some other tested kinases (CDK2/CycA2, PKA and AKT1). In view of the promising potential of GSK-3β inhibitors in the treatment of depression and associated complications, the compounds 7q, 7b, 7e and 7n were evaluated for antidepressant activity by FST and TST models. All the test compounds were observed to significantly shorten the immobility period in the experimental rats in comparison to normal control group in both the models. Compound 7q showed maximum activity and was more effective than the standard drug fluoxetine. The docking study of the active compounds showed their key interactions with the GSK-3β protein target. The encouraging results of our work, suggest that the benzimidazole-based 1,3,4-oxadiazole-1,2,3-triazole conjugates as GSK-3β inhibitors may lead to molecules providing a significant improvement over the existing antidepressants and encourage further exploration of the pathological implications of GSK-3β.
Acknowledgements
The authors wish to express their thanks to Dr G. N. Qazi, Vice-Chancellor, Jamia Hamdard for providing necessary facilities to carry out this work. The authors thank DST-SERB, Govt. of India for awarding research project to HH and fellowship to IK. The author MAT is thankful to HNF for providing research assistance.
References
- J. R. Woodgett, EMBO J., 1990, 9, 2431–2438 CAS.
- L. T. Alon, S. Pietrokovski, S. Barkan, L. Avrahami, O. Kaidanovich-Beilin, J. R. Woodgett, A. Barnea and H. Eldar-Finkelman, FEBS Lett., 2011, 585, 1158–1162 CrossRef CAS PubMed.
- H. Wang, J. Brown and M. Martin, Cytokine, 2011, 53, 130–140 CrossRef CAS PubMed.
- C. Xu, N.-G. Kim and B. M. Gumbiner, Cell Cycle, 2009, 8, 4032–4039 CrossRef CAS PubMed.
- T. Del’Guidice, C. Latapy, A. Rampino, J. Khlghatyan, M. Lemasson, B. Gelao, T. Quarto, G. Rizzo, A. Barbeau, C. Lamarre, A. Bertolino, G. Blasi and J.-M. Beaulieu, Proc. Natl. Acad. Sci. U. S. A., 2015, 112, E4610–E4619 CrossRef PubMed.
- W. J. Song, E. A. Song, M. S. Jung, S. H. Choi, H. H. Baik, B. K. Jin, J. H. Kim and S. H. Chung, J. Biol. Chem., 2015, 290, 2321–2333 CrossRef CAS PubMed.
- A. R. Cole, FEBS J., 2013, 280, 5213–5227 CrossRef CAS PubMed.
- T. D. Gould, C. A. Zarate and H. K. Manji, J. Clin. Psychiatry, 2004, 65, 10–21 CrossRef CAS PubMed.
- J. A. McCubrey, L. S. Steelman, F. E. Bertrand, N. M. Davis, M. Sokolosky, S. L. Abrams, G. Montalto, A. B. D'Assoro, M. Libra, F. Nicoletti, R. Maestro, J. Basecke, D. Rakus, A. Gizak, Z. N. Demidenko, L. Cocco, A. M. Martelli and M. Cervello, Oncotarget, 2014, 5, 2881–2911 CrossRef PubMed.
- J. M. Beaulieu, S. Marion, R. M. Rodriguiz, I. O. Medvedev, T. D. Sotnikova, V. Ghisi, W. C. Wetsel, R. J. Lefkowitz, R. R. Gainetdinov and M. G. Caron, Cell, 2008, 132, 125–136 CrossRef CAS PubMed.
- O. Kaidanovich-Beilin, A. Milman, A. Weizman, C. G. Pick and H. Eldar-Finkelman, Biol. Psychiatry, 2004, 55, 781–784 CrossRef CAS PubMed.
- T. D. Gould, H. Einat, R. Bhat and H. K. Manji, Int. J. Neuropsychopharmacol., 2004, 7, 387–390 CrossRef CAS PubMed.
- A. O. Rosa, M. P. Kaster, R. W. Binfare, S. Morales, E. Martin-Aparicio, M. L. Navarro-Rico, A. Martinez, M. Medina, A. G. Garcia, M. G. Lopez and A. L. Rodrigues, Prog. Neuro-Psychopharmacol. Biol. Psychiatry, 2008, 32, 1549–1556 CrossRef CAS PubMed.
- X. Li, M. A. Frye and R. C. Shelton, Neuropsychopharmacology, 2012, 37, 77–101 CrossRef CAS PubMed.
- J. M. Beaulieu, J. Neuropsychiatry Clin. Neurosci., 2012, 37, 7–16 CrossRef PubMed.
- E. Beurel, L. Song and R. S. Jope, Mol. Psychiatry, 2011, 16, 1068–1070 CrossRef CAS PubMed.
- J. M. Beaulieu, R. R. Gainetdinov and M. G. Caron, Annu. Rev. Pharmacol. Toxicol., 2009, 49, 327–347 CrossRef CAS PubMed.
- W. T. O'Brien, A. D. Harper, F. Jove, J. R. Woodgett, S. Maretto, S. Piccolo and P. S. Klein, J. Neurosci., 2004, 24, 6791–6798 CrossRef PubMed.
- J. M. Beaulieu, X. Zhang, R. M. Rodriguiz, T. D. Sotnikova, M. J. Cools, W. C. Wetsel, R. R. Gainetdinov and M. G. Caron, Proc. Natl. Acad. Sci. U. S. A., 2008, 105, 1333–1338 CrossRef CAS PubMed.
- F. Prati, A. De Simone, P. Bisignano, A. Armirotti, M. Summa, D. Pizzirani, R. Scarpelli, D. I. Perez, V. Andrisano, A. Perez-Castillo, B. Monti, F. Massenzio, L. Polito, M. Racchi, A. D. Favia, G. Bottegoni, A. Martinez, M. L. Bolognesi and A. Cavalli, Angew. Chem., Int. Ed., 2015, 54, 1578–1582 CrossRef CAS PubMed.
- T. Silva, J. Reis, J. Teixeira and F. Borges, Ageing Res. Rev., 2014, 15, 116–145 CrossRef CAS PubMed.
- M. Saitoh, J. Kunitomo, E. Kimura, Y. Hayase, H. Kobayashi, N. Uchiyama, T. Kawamoto, T. Tanaka, C. D. Mol, D. R. Dougan, G. S. Textor, G. P. Snell and F. Itoh, Bioorg. Med. Chem., 2009, 17, 2017–2029 CrossRef CAS PubMed.
- M. Saitoh, J. Kunitomo, E. Kimura, H. Iwashita, Y. Uno, T. Onishi, N. Uchiyama, T. Kawamoto, T. Tanaka, C. D. Mol, D. R. Dougan, G. P. Textor, G. P. Snell, M. Takizawa, F. Itoh and M. Kori, J. Med. Chem., 2009, 52, 6270–6286 CrossRef CAS PubMed.
- T. Onishi, H. Iwashita, Y. Uno, J. Kunitomo, M. Saitoh, E. Kimura, H. Fujita, N. Uchiyama, M. Kori and M. Takizawa, J. Neurochem., 2011, 119, 1330–1340 CrossRef CAS PubMed.
- M. A. Khanfar, R. A. Hill, A. Kaddoumi and K. A. El Sayed, J. Med. Chem., 2010, 53, 8534–8545 CrossRef CAS PubMed.
- A. G. Koryakova, Y. A. Ivanenkov, E. A. Ryzhova, E. A. Bulanova, R. N. Karapetian, O. V. Mikitas, E. A. Katrukha, V. I. Kazey, I. Okun, D. V. Kravchenko, Y. V. Lavrovsky, O. M. Korzinov and A. V. Ivachtchenko, Bioorg. Med. Chem. Lett., 2008, 18, 3661–3666 CrossRef CAS PubMed.
- F. Lo Monte, T. Kramer, J. Gu, U. R. Anumala, L. Marinelli, V. La Pietra, E. Novellino, B. Franco, D. Demedts, F. Van Leuven, A. Fuertes, J. M. Dominguez, B. Plotkin, H. Eldar-Finkelman and B. Schmidt, J. Med. Chem., 2012, 55, 4407–4424 CrossRef CAS PubMed.
- D. Shin, S.-C. Lee, Y.-S. Heo, W.-Y. Lee, Y.-S. Cho, Y. E. Kim, Y.-L. Hyun, J. M. Cho, Y. S. Lee and S. Ro, Bioorg. Med. Chem. Lett., 2007, 17, 5686–5689 CrossRef CAS PubMed.
- A. J. Peat, J. A. Boucheron, S. H. Dickerson, D. Garrido, W. Mills, J. Peckham, F. Preugschat, T. Smalley, S. L. Schweiker, J. R. Wilson, T. Y. Wang, H. Q. Zhou and S. A. Thomson, Bioorg. Med. Chem. Lett., 2004, 14, 2121–2125 CrossRef CAS PubMed.
- S. M. Sondhi, N. Singh, A. Kumar, O. Lozach and L. Meijer, Bioorg. Med. Chem., 2006, 14, 3758–3765 CrossRef CAS PubMed.
- P. H. Olesen, A. R. Sørensen, B. Ursø, P. Kurtzhals, A. N. Bowler, U. Ehrbar and B. F. Hansen, J. Med. Chem., 2003, 46, 3333–3341 CrossRef CAS PubMed.
- F. Lo Monte, T. Kramer, J. Gu, M. Brodrecht, J. Pilakowski, A. Fuertes, J. M. Dominguez, B. Plotkin, H. Eldar-Finkelman and B. Schmidt, Eur. J. Med. Chem., 2013, 61, 26–40 CrossRef CAS PubMed.
- R. Saha, O. Tanwar, A. Marella, M. M. Alam and M. Akhter, Mini-Rev. Med. Chem., 2013, 13, 1027–1046 CrossRef CAS PubMed.
- Y. Bansal and O. Silakari, Bioorg. Med. Chem., 2012, 20, 6208–6236 CrossRef CAS PubMed.
- S. G. Agalave, S. R. Maujan and V. S. Pore, Chem.–Asian J., 2011, 6, 2696–2718 CrossRef CAS PubMed.
- A. Baki, A. Bielik, L. Molnár, G. Szendrei and G. M. Keserü, Assay Drug Dev. Technol., 2007, 5, 75–83 CrossRef CAS PubMed.
- Q. Ye, W. Mao, Y. Zhou, L. Xu, Q. Li, Y. Gao, J. Wang, C. Li, Y. Xu, Y. Xu, H. Liao, L. Zhang, J. Gao, J. Li and T. Pang, Bioorg. Med. Chem., 2015, 23, 1179–1188 CrossRef CAS PubMed.
- J. F. Cryan, A. Markou and I. Lucki, Trends Pharmacol. Sci., 2002, 23, 238–245 CrossRef CAS PubMed.
- V. Castagne, P. Moser, S. Roux and R. D. Porsolt, Current protocols in neuroscience/editorial board, J. N. Crawley, et al., 2011, ch. 8, Unit 8 10A Search PubMed.
- M. A. Roni and S. Rahman, Pharmacol., Biochem. Behav., 2015, 139, 1–6 CrossRef CAS PubMed.
- J. F. Cryan, C. Mombereau and A. Vassout, Neurosci. Biobehav. Rev., 2005, 29, 571–625 CrossRef CAS PubMed.
- R. Chermat, B. Thierry, J. A. Mico, L. Steru and P. Simon, J. Pharmacol., 1986, 17, 348–350 CAS.
- C. Millon, A. Flores-Burgess, M. Narvaez, D. O. Borroto-Escuela, L. Santin, C. Parrado, J. A. Narvaez, K. Fuxe and Z. Diaz-Cabiale, Int. J. Neuropsychopharmacol., 2015, 18, 1–13 Search PubMed.
Footnote |
† Electronic supplementary information (ESI) available. See DOI: 10.1039/c6ra07273a |
|
This journal is © The Royal Society of Chemistry 2016 |
Click here to see how this site uses Cookies. View our privacy policy here.