DOI:
10.1039/C6RA07277A
(Paper)
RSC Adv., 2016,
6, 46828-46833
Synthesis, structure and characterization of 1-D polymer {[Sr(CHZ)2(ClO4)]ClO4}n: a new concept for designing primary explosives†
Received
21st March 2016
, Accepted 4th May 2016
First published on 6th May 2016
Abstract
A novel alkaline-earth metal perchlorate polymer complex {[Sr(CHZ)2(ClO4)]ClO4}n (1, CHZ = carbohydrazide) was synthesized and characterized. The X-ray single crystal diffraction results illustrate that the structure of the title complex belongs to the triclinic system, space group P
. In 1, every Sr2+ ion was ennea-coordinated with four CHZ groups and one perchlorate group. Noticeably, CHZ adopted a tridentate coordination mode with different Sr2+ ions, leading to a multinuclear structure. Therefore, the whole molecule was extended to a super 1-D polymer, and the configuration of Sr2+ ion was a tricapped trigonal prism of a tetradecahedron. The DSC result shows only one exothermal decomposition with peak temperature at 296.1 °C. The TG–DTG curve demonstrates a 67.8% mass loss from 266 °C to 332 °C. Non-isothermal kinetic and thermodynamic parameters were obtained via Kissinger's method as well as Ozawa's method. The critical temperature of thermal explosion, ΔS, ΔH, and ΔG were calculated as 248.1 °C, 35.61 J K−1 mol−1, 161.15 kJ mol−1, 152.42 kJ mol−1, respectively. Additionally, the sensitivities towards impact and friction were assessed via relevant standard methods.
Introduction
High-energy-density materials (HEDMs) have attracted increasing attention in the past few decades, such as CL-20, plastic bonded explosives, various tetrazolium and triazolium salts, as well as heavy-metal-free energetic polymers.1–6 In present works, energetic polymers are extensively applied as binders in explosive materials and propellants, because they can add to the total energy release in addition to performing required viscoelastic and adhesion functions.7 Coordination polymers of organic–metal frameworks have attracted plenty of attention owing to their promising applications as special functional materials.8–12 The considerable amount of research into infinite one-, two-, and three-dimensional networks has become a rapidly-growing field, especially into those compounds with backbones constructed by metal ions as connectors and ligands as linkers.8,13–15 Our group are trying to introduce the polymeric chain structure, which may lead to lower sensitivities due to their compact molecular relationship, to the compounds used as primary explosives.
In our previous works, azotic and oxygenic chain ligands obtained much attention, such as carbohydrazide (CHZ) and semicarbazide (SCZ) due to their high nitrogen content and the chelating effect which did enhance the molecular structural stability.16–18 According to the literatures,16,19,20 CHZ is a kind of azotic and oxygenic ligand with nitrogen- and oxygen-atom lone electron pairs, which can be either monodentate or multidentate while coordinating with metal ions (Fig. 1). As a ramification of hydrazine, CHZ possesses strong reduction ability so that it can react simultaneously with metal ions and oxidizing groups. Several metal carbohydrazido compounds,16,17 such as cadmium tri-(carbohydrazide)perchlorate and zinc tri-(carbohydrazide)perchlorate,21 have been successfully synthesized by our group and prove to be outstanding in explosive applications. Meanwhile, researchers also paid much attention to energetic materials based on alkaline-earth metals. In 2009, Klapötke22 observed calcium 5-nitriminotetrazolate as the most probable replacement of lead azide in primary explosives. In early 2002, we tended our interest to energetic polymers, and successfully investigated {[Ca(CHZ)2(H2O)](NTO)2·3.5H2O}n and {[Sr(CHZ)2(H2O)](NTO)2·3.5H2O}n (NTO = 3-nitro-1,2,4-triazolo-5-one). However, due to the existence of coordinated water, the obtained powder could not perform well in explosion. Magnesium tri-(carbohydrazide) perchlorate was also synthesized in 2012, but it didn't show excellent explosive properties, either.23
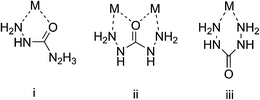 |
| Fig. 1 Coordination patterns of CHZ. | |
Herein, we continued our studies on the polymeric coordination based on carbohydrazide, and finally found a compound that possessed outstanding explosive performances with proper sensitivities and decomposition temperature: {[Sr(CHZ)2(ClO4)]ClO4}n. Here, the synthesis, structure, and thermal behaviour of 1, as well as its practical performances were reported.
Results and discussion
Structure description
A perspective molecular structure of 1 and coordination polyhedron of central strontium are illustrated in Fig. 2. Selected bond lengths and bond angles are listed in Tables 1 and 2 separately.
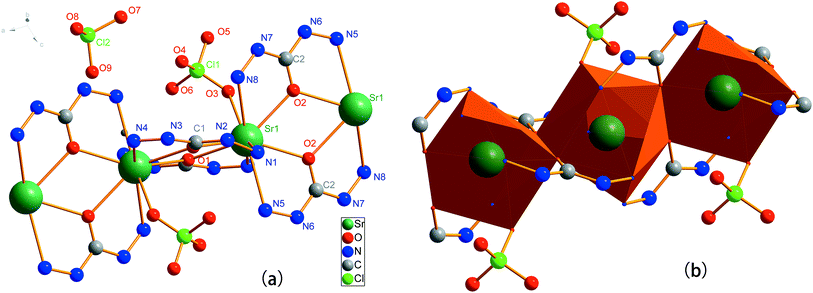 |
| Fig. 2 Molecular structure of 1 (a), and coordination polyhedron of Sr ion (b). | |
Table 1 Selected bond lengths for 1
Bond |
Length/Å |
Symmetry: 1 − x, 1 − y, 1 − z. Symmetry: −x, 1 − y, 1 − z. |
Sr1–O1a |
2.6016(14) |
Sr1–O1 |
2.5694(13) |
Sr1–O2b |
2.5962(14) |
Sr1–O2 |
2.5649(13) |
Sr1–N1 |
2.7870(18) |
Sr1–N4a |
2.7858(18) |
Sr1–N5 |
2.7801(18) |
Sr1–N8b |
2.7779(17) |
Sr1–O3 |
2.6798(15) |
Table 2 Selected bond angles for 1
Bond |
Angle/(°) |
Bond |
Angle/(°) |
Symmetry: −x, 1 − y, 1 − z. Symmetry: 1 − x, 1 − y, 1 − z. |
O2a–Sr1–O1 |
131.30(4) |
N5–Sr1–N1 |
73.94(5) |
O2–Sr1–O1b |
125.17(4) |
N5–Sr1–N4b |
74.08(5) |
O2a–Sr1–O1b |
139.55(4) |
N8a–Sr1–O1 |
72.11(4) |
O2–Sr1–O1 |
149.61(4) |
N8a–Sr1–O1b |
111.61(4) |
N1–Sr1–O1 |
60.41(4) |
N8a–Sr1–O2a |
59.78(4) |
N1–Sr1–O1b |
120.38(4) |
N8a–Sr1–O2 |
119.26(4) |
N1–Sr1–O2a |
95.35(5) |
N8a–Sr1–N1 |
71.30(5) |
N1–Sr1–O2 |
94.95(4) |
N8a–Sr1–N4b |
140.49(5) |
N4b–Sr1–O1 |
123.16(4) |
N8a–Sr1–N5 |
145.13(5) |
N4b–Sr1–O1b |
60.04(4) |
O3–Sr1–O1 |
106.47(4) |
N4b–Sr1–O2a |
100.04(5) |
O3–Sr1–O1b |
71.41(4) |
N4b–Sr1–O2 |
67.91(4) |
O3–Sr1–O2a |
68.30(4) |
N4b–Sr1–N1 |
147.98(5) |
O3–Sr1–O2 |
103.85(4) |
N5–Sr1–O1 |
93.15(4) |
O3–Sr1–N1 |
144.94(5) |
N5–Sr1–O1b |
88.32(5) |
O3–Sr1–N4b |
67.07(5) |
N5–Sr1–O2a |
121.80(4) |
O3–Sr1–N5 |
141.12(5) |
N5–Sr1–O2 |
61.01(4) |
O3–Sr1–N8a |
73.70(5) |
Compound 1 crystallizes in the triclinic system, space group P
. The SrII ion has sp3d5 hybridization and contributes nine empty orbits to accommodate the lone pair of electrons from ligands. Namely, the coordination number of strontium ion is nine, which is definitely rare for this kind of compounds. As presented in Fig. 2, every Sr ion coordinates with four CHZ molecules. The terminal N atom and the carbonyl O atom from one CHZ molecule simultaneously coordinate to the same Sr ion forming a five-membered cyclic. The four CHZ molecules that coordinated with one Sr ion can be divided into two different groups, of which every two interlaced CHZ molecules form bonds with the same two Sr ions. Meanwhile, two oxo bridges, composed by the carbonyl O atoms from CHZ molecules, are lying between every two neighbouring Sr ions forming a four-membered cyclic. That is to say, the molecule is extended to a ring-like 1-D chelating polymer. Additionally, coordination bond also connects central metal ion to O atom of a perchlorate group, while another perchlorate ion performs as outer anion of the complex. Therefore, the configuration of Sr ion can be depicted as a tricapped trigonal prism of tetradecahedron. In 1, the Sr–N coordination bond lengths range from 2.7870(18) to 2.7779(17) Å, which is longer than the Sr–O (terminal O of CHZ) coordination bonds with lengths from 2.5649(13) to 2.6016(14) Å.
As shown in Fig. 3, in the solid state adjacent Sr atoms of polymer 1 are linked by two pairs of CHZ ligands to form an infinite polymeric chain with the Sr⋯Sr separation of 4.38 Å. Noticeably, every CHZ group is distorted to certain degrees while performing as bridging ligand, whereas C, N, O atoms from one CHZ molecule are supposed to be in a same plane generally. That is to say, the five-membered chelate rings composed by Sr–N–N–C–O are all of distorted. In contrast, the four-membered rings composed by Sr–O–Sr–O are totally planar. Selected torsion angles are listed in Table 3. In addition, each 1D chain is surrounded by four adjacent 1D chains with N–H⋯O hydrogen bonds to form the 3D network exhibiting a primitive cubic topology, which can be regarded as discrete MOFs (Metal–Organic-Frameworks).
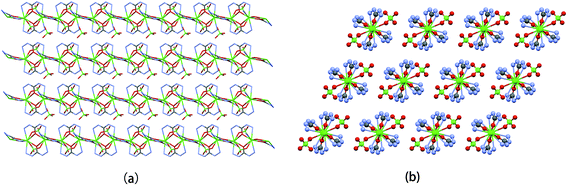 |
| Fig. 3 Packing structure of 1 along different viewing directions. | |
Table 3 Torsion angles for 1
Torsion angles |
Degrees/° |
N(8)–N(7)–C(2)–O(2) |
−3.8(3) |
N(8)–N(7)–C(2)–N(6) |
177.06(17) |
N(8)–N(7)–C(2)–Sr(1) |
30.5(4) |
N(5)–N(6)–C(2)–O(2) |
6.9(3) |
N(5)–N(6)–C(2)–N(7) |
173.93(17) |
N(5)–N(6)–C(2)–Sr(1) |
−6.75(17) |
N(4)–N(3)–C(1)–O(1) |
2.5(3) |
N(4)–N(3)–C(1)–N(2) |
179.69(16) |
N(4)–N(3)–C(1)–Sr(1) |
29.8(4) |
N(1)–N(2)–C(1)–O(1) |
−17.8(2) |
N(1)–N(2)–C(1)–N(3) |
165.06(16) |
N(1)–N(2)–C(1)–Sr(1) |
−25.19(14) |
N(5)–Sr(1)–C(2)–O(2) |
157.53(16) |
N(1)–Sr(1)–C(2)–O(2) |
−95.12(14) |
N(5)–Sr(1)–C(2)–N(6) |
4.30(11) |
N(1)–Sr(1)–C(2)–N(6) |
66.71(11) |
O(1)–Sr(1)–O(1)–Sr(1) |
0 |
O(2)–Sr(1)–O(2)–Sr(1) |
0 |
Furthermore, due to the existence of perchlorate groups, lots of intermolecular and intramolecular as well hydrogen bonds are lying in the molecule, which make an important contribution to enhance the thermal stability of the title compound. The hydrogen bonds lengths and angles are summarized in Table 4. The H-bonds donated by N1 and N5 atoms from adjacent CHZ groups can be described as intramolecular H-bonds, while the others belong to intermolecule.
Table 4 Hydrogen bond lengths/Å and bond angles/° for 1
D–H⋯A |
d(D–H) |
d(H⋯A) |
d(D⋯A) |
DHA |
N1–H1A⋯O9 |
0.89 |
2.311 |
3.156 |
158.51 |
N1–H1B⋯O6 |
0.89 |
2.482 |
3.152 |
132.43 |
N2–H2⋯O5 |
0.89 |
2.129 |
2.937 |
150.65 |
N3–H3⋯O1 |
0.89 |
2.357 |
3.059 |
135.79 |
N4–H4A⋯O3 |
0.89 |
2.592 |
3.019 |
110.33 |
N4–H4B⋯O7 |
0.89 |
2.336 |
3.198 |
163.35 |
N5–H5A⋯O9 |
0.89 |
2.533 |
3.386 |
160.57 |
N5–H5B⋯O8 |
0.89 |
2.283 |
3.171 |
175.78 |
N6–H6⋯O4 |
0.89 |
2.179 |
3.05 |
165.95 |
N7–H7⋯O5 |
0.89 |
2.04 |
2.913 |
166.68 |
N8–H8A⋯O8 |
0.89 |
2.275 |
3.128 |
160.26 |
N8–H8B⋯O10 |
0.89 |
2.522 |
3.155 |
128.53 |
Thermal decomposition mechanism
In order to investigate the thermal behaviours of 1, DSC and TG–DTG measurements were performed under linear heating rate of 10 K min−1 in a nitrogen atmosphere. As demonstrated in Fig. 4, only one exothermic peak was detected with the onset temperature of 284.6 °C and peak temperature of 296.1 °C, illustrating that compound 1 possesses high energy property and rapid exothermic process. Corresponding to DSC curve, the TG–DTG curve reveals a rapid weight loss progress with 47% between 266 and 350 °C. It reaches the largest rate of mass loss percentage of 15% per K at 291 °C. Afterwards, it continues losing weight but on a slower velocity from 350 to 490 °C. The mass of the final residue was 32.2% of initial. Drawing from above mentioned two curves, compound 1 decomposes very rapidly but with a rarely high decomposition temperature, unlike most of other perchlorate compounds. In general, perchlorates are always introduced into energetic materials due to its explosibility, but problem accompanies: low thermal stability. However, to our delight, the 1-D polymer and multiple cyclic structures as well as the chelate rings of 1 stabilize the whole molecule, resolving the conflict precisely.
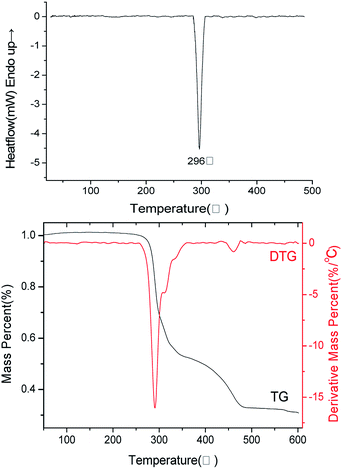 |
| Fig. 4 DSC and TG–DTG curves for 1 in a nitrogen atmosphere with a heating rate of 10 K min−1. | |
Non-isothermal kinetic and thermodynamic parameters
In present works, Kissinger's24 and Ozawa's25 methods have been widely used in academic researches to determine the kinetic parameters such as apparent activation energy (Ea) and the pre-exponential factor (A).26–29 The instructive equations of the Kissinger's and Ozawa's methods are as follows, respectively: |
ln β/Tp2 = ln(RA/Ea) − Ea/(RTp)
| (1) |
|
lg β = lg[AEa/RG(α)] − 2.315 − 0.4567Ea/(RTp)
| (2) |
where β is the linear heating rate (K min−1), Tp is the peak temperature (K), Ea is the apparent activation energy (kJ mol−1), A is the pre-exponential factor (s−1), R is the gas constant (8.314 J K−1 mol−1), and G(α) is the reaction mechanism function.
Both Kissinger's and Ozawa's methods are based on the first exothermic peak temperatures measured at four different heating rates of 5, 10, 15, and 20 K min−1. Furthermore, from the original data, the apparent activation energy Ea, pre-exponential factor A (not necessary in Ozawa method),29 linear coefficient Rc and standard deviations S were calculated by the original experimental data and shown in Table 5. In particular, the apparent activation energies (Ea) determined by two methods are in substantial agreement. The average value of two methods are used as a reasonable result. Consequently, the Arrhenius equations could be expressed as follows:
ln k = 34.29 − 163.63 × 103/(RT) |
Table 5 Peak temperatures of the first exotherm and chemical kinetics parameters
β/K min−1 |
Tp/°C |
Parameter |
Kissinger's method |
Ozawa's method |
5 |
280.1 |
Ea/kJ mol−1 |
163.18 |
164.07 |
10 |
293.7 |
ln A |
34.29 |
— |
15 |
296.9 |
Rc |
−0.982 |
−0.984 |
20 |
300.9 |
S |
0.132 |
0.057 |
The peak temperature of thermal decomposition (Tp) and the critical temperature of thermal explosion (Tb) are generally regarded as significant indicators of energetic materials while considering their thermal safety.30 The calculation method of two temperatures using DSC data are as follows:31
|
E(Tb − T0) − RTb2 = 0
| (4) |
where
b and
c are coefficients,
Tp0 is the peak temperature when
β tends to zero (K),
T0 is the onset temperature from the non-isothermal DSC curves.
The thermodynamic parameters including the entropy of activation (ΔS≠), enthalpy of activation (ΔH≠), and free energy of activation (ΔG≠) were also determined based on the DSC data according to the following equations:32,33
|
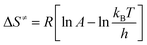 | (6) |
where
kB is the Boltzmann constant (1.381 × 10
−23 J K
−1), and
h is the Planck constant (6.626 × 10
−34 J s). The calculated results were listed in the
Table 6.
Table 6 Thermodynamics of 1
Tp0/°C |
Tb/°C |
ΔS≠ J K−1 mol−1 |
ΔH≠ kJ mol−1 |
ΔG≠ kJ mol−1 |
244.9 |
248.1 |
35.61 |
161.15 |
152.42 |
Sensitivity tests
For initial safety testing, the impact and friction sensitivities34 were determined by following BAM standard methods.35,36 According to the “UN Recommendations on the Transport of Dangerous Goods”,37 compound 1 could be depicted as “very sensitive” to both impact (2.5 J) and friction (36 N). As mentioned above, thermal decomposition temperature of 1 is around 296 °C,21,38,39 which could be named as “thermal-stable primary explosive”. However, in previous studies, a sensitive charge always means unstable thermal properties, especially those who contain perchlorates.
Now, thanks to the introducing of structure of 1-D polymers and chelating rings, the conflict seems to be well solved. Simultaneously, investigation results of compound 1 provide us with a new potential route to reconsider the primary explosives.
Experimental
Materials and physical techniques
All the solvents and reagents were commercially obtained and of analytical grade without further purification. Elemental analyses were performed with a Flash EA 1112 fully automatic trace element analyzer. The FT-IR spectra was recorded with a Bruker Equinox 55 infrared spectrometer (KBr pellets) in the range of 4000–400 cm−1 with a resolution of 4 cm−1. DSC and TG–DTG measurements were performed with Pyris-1 differential scanning calorimeter and Pyris-1 thermogravimetric analyzer (Perkin-Elmer, USA) in a dry nitrogen gas atmosphere with flowing rate of 20 mL min−1. The condition of the thermal analyses was as follows: the crystal sample was powdered and sealed in an aluminum pans with a linear heating rate of 20 K min−1 from 20 °C to 500 °C. The impact sensitivity was determined by Fall Hammer Method using BAM FALL HAMMER BFH-10, and the friction sensitivity was determined with a BAM FRICTION APPARATUS FSKM-10 by following a BAM standard.
Caution! The compound 1 we report in this paper is very sensitive and tend to explode in certain conditions. Therefore, appropriate safety precautions must be taken at all times, especially while manipulating. Laboratories and personnel should be properly grounded and safety equipments.
Synthesis
Carbohydrazide (1.8 g, 20 mmol) was dissolved in deionized water (20 mL), which was charged into a glass reactor with a thermo-water bath. The reaction solution was stirred with a mechanical agitator and heated to 80 °C for using. Then an aqueous solution (10 mL) containing strontium perchlorate (2.87 g, 10 mmol) was added to the above mixture with continuous stirring and keeping at 80 °C for 60 min. Afterwards, while the colorless solution was cooled to the room temperature naturally, they were poured into a beaker by filtration. The 1 would precipitate in 2–3 days. Finally, the product was collected by filtration, washed with ethanol and dried in an explosion-proof water-bath dryer. Yield: 78% (3.64 g). Elemental analysis calcd for C2H12C12N8O10Sr (%): C 5.14, H 2.57, N 23.99; found (%): C 5.08, H 2.61, N 23.87. FT-IR (KBr pellet) data (ν cm−1): ν 3370 (N–H), ν 3338 (N–H), ν 1668 (C
O), δ 1508 (N–H), δ 1565(N–H), ν 1062 (ClO4−), ν 624 (ClO4−).
X-ray data collection and structure refinement
The colourless crystal was selected carefully for X-ray determination. X-ray diffraction data collection was performed by a Rigaku AFC-10/Saturn 724+ CCD detector diffractometer with graphite monochromated Mo-Kα radiation (λ = 0.071073 nm) with ϕ and ω modes at 103(2) K. The structure was solved by direct methods of using SHELXS-97 (ref. 40) and refined by full-matrix least-squares methods on F2 with SHELXL-97 program.41 All non-hydrogen atoms were obtained from the different Fourier maps and subjected to anisotropic refinement by full-matrix least-squares on F2. Detailed information concerning crystallographic data collection and structure refinement are summarized in Table 7.
Table 7 Crystal data and structure of compound 1
{[Sr(CHZ)2(ClO4)]ClO4}n |
Empirical formula |
C2H12Cl2N8O10Sr |
Formula weight |
466.72 |
Crystal system |
Triclinic |
Space group |
P![[1 with combining macron]](https://www.rsc.org/images/entities/char_0031_0304.gif) |
a, b, c/Å |
8.749(2), 9.827(3), 10.261(3) |
α, β, γ/° |
108.825(2), 107.023(2), 103.786(2) |
Volume/Å3 |
742.3(3) |
Z |
2 |
ρcal/g cm−3 |
2.088 |
μ/mm−1 |
4.059 |
F(000) |
461.7 |
Crystal size/mm3 |
0.34 × 0.29 × 0.165 |
Radiation Mo-Kα (λ) |
0.71073 |
2θ range/° |
5 to 58.26 |
h, k, l |
−11 to 11, −13 to 13, −14 to 14 |
Reflns collected |
9918 |
Indep reflections |
3925 |
S |
0.949 |
R, wR2 [I ≥ 2σ(I)] |
R1 = 0.0236, wR2 = 0.0581 |
R, wR2 [all data] |
R1 = 0.0271, wR2 = 0.0596 |
Largest diff. peak/hole/e Å−3 |
0.48, −0.70 |
CCDC |
1457051 |
Conclusions
From detailed experimental investigation of the energetic compound {[Sr(CHZ)2(ClO4)]ClO4}n (1, CHZ = carbohydrazide), the following conclusions can be drawn.
A new polymer energetic complex, which is heavy-metal-free, has been successfully synthesized and also characterized by single crystal X-ray diffraction, as well as IR spectra. In 1, Sr2+ performs a rare ennea-coordination mode with two CHZ groups and one perchlorate group. All of the carbohydrazide molecules serve as tridentate ligands to coordinate with Sr2+ ions to form five-membered rings, which gives the complex a 1-D polymer structure. Being worth mentioning, perchlorate groups perform both as ligands and outer anions in 1. The great oxidizability of perchlorate provides the compound with high-energy output and sensitive performance when stimulated. Thermal analysis curves reveal that the decomposition of 1 starts at 284.6 °C with a sharp exothermic peak at 296.1 °C. The thermal parameters Ea, ln
A, Rc, S were obtained by two methods and the Arrhenius equation of 1 can be expressed as: ln
k = 34.29 − 163.63 × 103/(RT). Furthermore, the critical temperature of thermal explosion, ΔS, ΔH, and ΔG were calculated out as 248.1 °C, 35.61 J °C−1 mol−1, 161.15 kJ mol−1, 152.42 kJ mol−1, respectively. In summary, complex 1, as a thermally stable but mechanically sensitive energetic material, could be a kind of prominent and potential primary charge for practical applications. The introducing of polymer structure to energetic molecule are also on the way.
Acknowledgements
The authors acknowledge financial support from the State Key Laboratory of Explosion and Technology (No. QNKT12-02), Science and Applied Physical Chemistry Laboratory (No. 9140C370303120C37142). We are truly grateful to Dr Fugang Li, Yong-an Feng and Sitong Chen for many helpful discussions.
Notes and references
- A. A. Dippold and T. M. Klapötke, Chem.–Asian J., 2013, 8, 1463–1471 CrossRef CAS PubMed.
- D. Fischer, T. M. Klapötke, M. Reymann and J. Stierstorfer, Chem.–Eur. J., 2014, 20, 6401–6411 CrossRef CAS PubMed.
- M. A. Kettner, K. Karaghiosoff, T. M. Klapötke, M. Suceska and S. Wunder, Chem.–Eur. J., 2014, 20, 7622–7631 CrossRef CAS PubMed.
- V. Thottempudi, P. Yin, J. H. Zhang, D. A. Parrish and J. M. Shreeve, Chem.–Eur. J., 2014, 20, 542–548 CrossRef CAS PubMed.
- S. Cudzilo and M. Nita, J. Hazard. Mater., 2010, 177, 146–149 CrossRef CAS PubMed.
- Z. H. Mi, Y. G. Bi, Y. A. Feng and T. L. Zhang, Z. Anorg. Allg. Chem., 2016, 642, 317–322 CrossRef CAS.
- D. S. Moore and S. D. McGrane, J. Mol. Struct., 2003, 661, 561–566 CrossRef.
- S. Kitagawa, R. Kitaura and S. Noro, Angew. Chem., 2004, 43, 2334–2375 CrossRef CAS PubMed.
- S. Kitagawa and M. Kondo, Bull. Chem. Soc. Jpn., 1998, 71, 1739–1753 CrossRef CAS.
- M. Kondo, T. Yoshitomi, K. Seki, H. Matsuzaka and S. Kitagawa, Angew. Chem., Int. Ed., 1997, 36, 1725–1727 CrossRef CAS.
- H. Li, M. Eddaoudi, M. O'Keeffe and O. M. Yaghi, Nature, 1999, 402, 276–279 CrossRef CAS.
- O. M. Yaghi, H. L. Li and T. L. Groy, Inorg. Chem., 1997, 36, 4292–4293 CrossRef CAS PubMed.
- B. Moulton and M. J. Zaworotko, Chem. Rev., 2001, 101, 1629–1658 CrossRef CAS PubMed.
- J. Lee, O. K. Farha, J. Roberts, K. A. Scheidt, S. T. Nguyen and J. T. Hupp, Chem. Soc. Rev., 2009, 38, 1450–1459 RSC.
- S. R. Batten and R. Robson, Angew. Chem., Int. Ed., 1998, 37, 1460–1494 CrossRef.
- W. Tong, R. Zhang, T. Zhang and L. Yang, RSC Adv., 2015, 5, 22031–22037 RSC.
- Z. H. Liu, T. L. Zhang, J. G. Zhang, L. Yang, J. Zhang and Y. Zang, Chin. J. Energ. Mater., 2008, 16, 663 CAS.
- Y. M. Yang, T. L. Zhang, J. G. Zhang and S. B. Guo, Chin. J. Struct. Chem., 2002, 21, 321–324 CAS.
- R. L. Dutta, J. Inorg. Nucl. Chem., 1981, 43, 2557–2559 CrossRef CAS.
- T. S. Konkova, Y. N. Matyushin, V. P. Sinditskii and A. E. Fogelsang, Khim. Fiz., 1995, 14, 109–113 CAS.
- T. L. Zhang, Z. R. Wei and C. H. Lv, Chin. J. Explos. Propellants, 1999, 28, 16–19 CAS.
- T. M. Klapötke and J. Stierstorfer, A green replacement for lead azide: calcium 5-nitriminotetrazolate, Czech Republic, 2009 Search PubMed.
- Z.-M. Li, T.-L. Zhang, L. Yang, Z.-N. Zhou and J.-G. Zhang, J. Coord. Chem., 2012, 65, 143–155 CrossRef CAS.
- H. E. Kissinger, Anal. Chem., 1957, 29, 1702–1706 CrossRef CAS.
- T. Ozawa, Bull. Chem. Soc. Jpn., 1965, 38, 1881 CrossRef CAS.
- R. Liu, W. Y. Zhao, T. L. Zhang, L. Yang, Z. N. Zhou and S. Y. Qi, RSC Adv., 2014, 4, 39463–39471 RSC.
- R. Liu, T. L. Zhang, Z. N. Zhou and L. Yang, RSC Adv., 2014, 4, 9810–9818 RSC.
- Z. Li, Z. Zhou, T. Zhang, Z. Tang, L. Yang and J. Zhang, Polyhedron, 2012, 44, 59–65 CrossRef.
- M. J. Starink, Thermochim. Acta, 1996, 288, 97–104 CrossRef CAS.
- J. Y. Lv, L. P. Chen, W. H. Chen, H. S. Gao and M. J. Peng, Thermochim. Acta, 2013, 571, 60–63 CrossRef CAS.
- T. L. Zhang, R. Z. Hu, Y. Xie and F. P. Li, Thermochim. Acta, 1994, 244, 171–176 CrossRef CAS.
- J. H. Yi, F. Q. Zhao, B. Z. Wang, Q. A. Liu, C. Zhou, R. Z. Hu, Y. H. Ren, S. Y. Xu, K. Z. Xu and X. N. Ren, J. Hazard. Mater., 2010, 181, 432–439 CrossRef CAS PubMed.
- R. Liu, T. L. Zhang, L. Yang, Z. N. Zhou and X. C. Hu, Cent. Eur. J. Chem., 2013, 11, 774–781 CAS.
- M. Sućeska, Test Methods for Explosives, Springer, New York, 1995 Search PubMed.
-
(a) NATO standardization agreement (STANAG) on explosives, impact-sensitivity tests, no. 4489, 1st edn, 17 September 1999 Search PubMed;
(b) NATO standardization agreement (STANAG) on explosive, friction sensitivity tests, no. 4487, 1st edn, 22 August 2002 Search PubMed.
- http://www.bam.de.
- Impact: insensitive >40 J, less sensitive ≥35 J, sensitive ≥4 J, very sensitive ≤3 J. Friction: insensitive >360 N, less sensitive = 360 N, 80 N < sensitive < 360 N, very sensitive <80 N, extremely sensitive <10 N.
- J. Zhang, T. Zhang, L. Yang, J. Zhang, Y. Cui, X. Hu and Z. Liu, Propellants, Explos., Pyrotech., 2009, 34, 24–31 CrossRef.
- H. X. Gao and J. M. Shreeve, RSC Adv., 2014, 4, 24874–24880 RSC.
- G. M. Sheldrick, SHELXL 97, Program for the Solution of Crystal Structures, University of Göttingen, Germany, 1990 Search PubMed.
- G. M. Sheldrick, SHELXL 97, Program for Crystal Structure Refinement from Diffraction Data, University of Göttingen, Germany, 1997 Search PubMed.
|
This journal is © The Royal Society of Chemistry 2016 |
Click here to see how this site uses Cookies. View our privacy policy here.