DOI:
10.1039/C6RA09371J
(Paper)
RSC Adv., 2016,
6, 56549-56561
Synthesis and characterization of Ni(II)–Vanillin–Schiff base–MCM-41 composite as an efficient and reusable nanocatalyst for multicomponent reactions
Received
11th April 2016
, Accepted 7th June 2016
First published on 7th June 2016
Abstract
An efficient heterogeneous catalyst (Ni(II)–Vanillin–Schiff base–MCM-41) has been synthesized by immobilization of nickel(II)–vanillin complex on MCM-41 nanostructure and characterized by XRD, TGA, BET, EDS, SEM, ICP-OES, TEM and FT-IR spectroscopy. The catalytic activity and stability of the prepared compound has been studied in some multicomponent reactions with polyhydroquinoline, 1H-indazolo[1,2-b]phthalazine-trione and 2,3-dihydroquinazolin-4(1H)-one derivatives under mild condition reactions. All the reactions were carried out in the presence of water as the solvent and under solvent-free conditions. The simple work up, mild conditions, excellent yield, inexpensive and non-toxic catalyst; makes this protocol both attractive and economically viable. More importantly, this catalyst can be reused several times without any significant loss of its catalytic activity.
1 Introduction
The development of efficient and selective synthetic transformations using inexpensive, available, and environmentally-benign catalysts and reagents is of great interest in modern organic synthesis.1 The heterogenization of homogeneous catalysts has become a major area of research, since the potential advantages of these materials such as simplified recovery and reusability over homogeneous systems can have positive environmental effects.2 Many porous materials have been widely studied with regard to technical applications as heterogeneous catalysts and excellent adsorbents. Typical porous materials include three classes: microporous (pore size < 2 nm), mesoporous (2–50 nm),3,4 and macroporous (>50 nm) materials.5 Recently, among the different types of supports used in heterogeneous catalysis, considerable interesting research was carried out on mesoporous MCM-41 materials,6 because they can display some advantageous properties, such as high activity, good accessibility, lager surface area, uniform pore structure, excellent stability (chemical and thermal), associated with high thioresistance for the hydrogenation of aromatics found in diesel fuels, and inert environment for immobilization of transition metal nanoparticles.7–9 Vanillin (4-hydroxy-3-methoxybenzaldehyde) is a readily available, inexpensive, non-toxic, ease and safe to handle aldehyde. It can be anchored on NH2–MCM-41 and act as a Schiff base ligand. Vanillin is currently used as a flavouring agent in foods, beverages, and pharmaceuticals. Multicomponent reactions (MCRs) as an important subclass of tandem reactions are in agreement with principles of green chemistry in chemical biology and drug discovery.10–13 Polyhydroquinoline, 1H-indazolo[1,2-b]phthalazine-triones and 2,3-dihydroquinazolin-4(1H)-ones are important building blocks in the synthesis of many natural products with a wide range of pharmacological and biological activities including antifertility, anticonvulsant, antibacterial, anticancer, antifungal, antitumor, and also as a mono amine oxidase inhibitor.14–20 Additionally, 2,3-dihydroquinazolin-4(1H)-one derivatives are the key intermediate for the synthesis of quinazolin-4(1H)-ones which have been found to possess a broad spectrum of pharmacological activities.21 Based on green chemistry desires, the development of new strategies for very low cost, relatively non-toxic and recycling of this catalyst, which minimize the energy and time required in achieving separations, can result in significant economic and environmental benefits. However, some of the catalytic applications of Schiff-base complexes of nickel immobilized on MCM-41 in organic reactions such as: epoxidation of olefins,22,23 oxidative coupling of thiols, oxidation of sulfides24 and also, other Ni-complex for organic reactions such as synthesis of 2,3-dihydroquinazolin-4(1H)-ones25 have been reported in the literature. In this work, we report an efficient procedure for the preparation of 2,3-dihydroquinazolin-4(1H)-one and polyhydroquinoline derivatives in water and 1H-indazolo[1,2-b]phthalazine-triones derivatives in solvent free conditions in good to excellent yields through a one-step reaction using various aromatic and aliphatic aldehyde in the presence of Ni(II)–Vanillin–Schiff base–MCM-41 as an efficient, green, reusable and inexpensive catalyst.
2 Experimental
2.1. Materials
The tetraethylorthosilicate (TEOS, 98%), cationic surfactant cetyltrimethylammonium bromide (CTAB, 98%), nickel(II) nitrate hexahydrate [Ni(NO3)2·6H2O], 4-hydroxy-3-methoxybenzaldehyde (vanillin), aromatic and aliphatic aldehydes, dimedon, ethyl acetoacetate, ammonium acetate, solvents and other reagents were purchased either from Merck, Aldrich or Fluka companies and were used as received without further purification.
2.2. Synthesis of MCM-41 modified with (3-aminopropyl)-triethoxysilane (3-APTES)
In a typical synthesis, 1 g of cetyltrimethylammonium bromide (CTAB) was added into an aqueous solution containing 3.5 mL of NaOH solution (2 M) and stirred at 80 °C until the solution became uniform. To this solution 5 mL tetraethylorthosilicate (TEOS) was slowly added. The resulting solution was refluxed for 2 h at the same temperature. The product was collected and washed with deionized water, and dried in an oven at 70 °C. Finally, the dried powders were calcined at 550 °C for 5 h with rate of 2 °C min−1 to remove the residual surfactant and obtain pure silica MCM-41. In continuation of this work, 4.8 g MCM-41 was mixed with 4.8 g (3-aminopropyl)-triethoxysilane (3-APTES) in n-hexane (96 mL) and heated at 80 °C for 24 hour under nitrogen atmosphere and reflux conditions. The resulting white solid of the functionalized MCM-41 (nPrNH2–MCM-41) was filtered, washed with n-hexane and dried under vacuum.
2.3. Preparation of heterogeneous catalyst Ni(II)–Schiff base complex grafted in mesoporous silica MCM-41
For grafting of the ligand to MCM-41–(SiCH2CH2CH2NH2)x, a mixture of dried nPrNH2–MCM-41 (1 mmol, 0.5 g) with vanillin (1.2 mmol, 0.182 g) in ethanol was refluxed under N2 atmosphere for 3 h. The resulting yellowish solid was filtered and washed with ethanol for several times and dried under vacuum. Finally, the mixture of yellowish precipitate (0.25 g) and Ni (NO3)2·6H2O (0.145 g. 0.5 mmol) was refluxed in ethanol at 80 °C for 20 h. The resulting catalyst was filtered, washed with ethanol and dried at room temperature (Scheme 1).
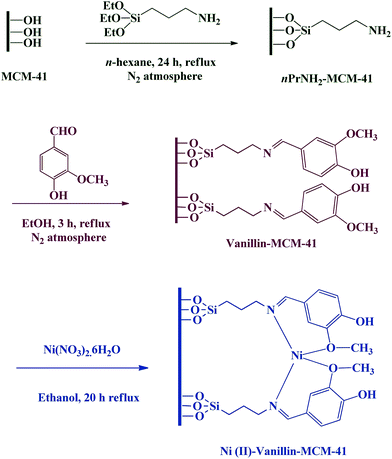 |
| Scheme 1 Synthesis of Ni–Vanilline–MCM-41 nanostructure. | |
2.4. General procedure for the synthesis of 2,3-dihydroquinazolin-4(1H)-one derivatives
A mixture of 2-aminobenzamide (1 mmol) and aldehyde (1 mmol) in the presence of Ni(II)–Vanillin–Schiff base–MCM-41 (0.187 mol%, 4 mg) as catalyst, was stirred in H2O as solvent at room temperature for an appropriate time. The progress of reaction was monitored by TLC (eluent; n-hexane
:
ethyl acetate, 8
:
2). After completion of the reaction, the catalyst was separated by filtration and then recrystallized from ethanol–water to obtain pure product. The solvent was evaporated and the product was obtained.
2.5. General procedure for the synthesis of polyhydroquinoline derivatives
Ni(II)–Vanillin–Schiff base–MCM-41 catalyst (35 mg, 0.93 mol%) was added to a mixture of aldehyde (1 mmol), dimedon (1 mmol), ethyl acetoacetate (1 mmol) and ammonium acetate (1.2 mmol) in water (2 mL), and the reaction mixture was stirred at 80 °C for an appropriate time. After completion of the reactions (monitored by TLC, eluent; n-hexane
:
ethyl acetate, 8
:
2) the product was filtered and then recrystallized from ethanol–water to obtain pure product.
2.6. General procedure for the synthesis of 2H-indazolo[2,1-b]phthalazine-triones derivatives
To a mixture of aldehyde (1 mmol), phthalhydrazide (1 mmol) and dimedon (1 mmol), Ni(II)–Vanillin–Schiff base–MCM-41 (10 mg, 0.267 mol%) was added and the mixture was stirred and heated in an oil bath at 100 °C for appropriate time. Completion of the reaction was indicated by TLC (n-hexane/ethylacetate 8
:
2). After the reaction was completed, the reaction mixture was cooled to room temperature. Then the solid residue was washed well with H2O
:
EtOH (90
:
10). The solid product was purified by recrystallization procedure from ethanol.
3 Results and discussion
In continuation of our previously investigations,26–28 herein we wish to report an efficient, facile, and environmentally friendly procedure for the synthesis of 2,3-dihydroquinazolin-4(1H)-ones, polyhydroquinoline and 1H-indazolo[1,2-b] phthalazine-triones under mild conditions in the presence of catalytic amount of Ni(II)–Vanillin–Schiff base–MCM-41. All the reactions were carried out in green solvent (H2O) and/or under solvent-free conditions. The prepared new heterogeneous catalyst was characterized by XRD, TGA, BET, EDS, SEM, TEM, ICP-OES and FT-IR spectroscopy.
3.1. X-ray diffraction
The XRD pattern of MCM-41 (Fig. 1(a)) shows 3 low angle reflection of (d100), (d110) and (d200) at 2θ = 2.77, 4.67 and 5.13 respectively, indexing to the hexagonal regularity of MCM-41 mesoporous structure. Those three reflection peaks were also observed in our study of the Ni(II)–Vanillin–Schiff base–MCM-41 sample (Fig. 1(b)). The XRD spectrum in this case has been showed a great reduction in the peak intensity, as shown in Fig. 1.29 When nickel loading is below 5 wt%, in higher angle range of XRD pattern no diffraction peak can be observed30 and with above 5 wt% nickel, diffraction peaks can be observed in higher angle.31 Here, no diffraction peak is observed at a high angle range for catalyst30 (Fig. 1(c)). These observations indicated that the nickel(II) metal is immobilized on the inner channel pores and providing evidence of functionalization occurring mainly inside of mesopore channels.32 The peak with a wide 2θ range (the broad peck appeared in around 2θ = 27) are related to amorphous silica.33
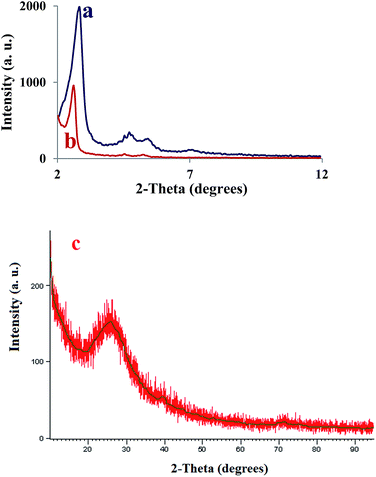 |
| Fig. 1 XRD patterns of: (a) MCM-41, (b) Ni(II)–Vanillin–Schiff base–MCM-41 and (c) high angle XRD patterns of Ni(II)–Vanillin–Schiff base–MCM-41. | |
3.2. FT-IR spectroscopy
The FT-IR spectra of MCM-41, nPrNH2-MCM-41, Vanillin–MCM-41 and Ni(II)–Vanillin–Schiff base–MCM-41 were shown in Fig. 2. MCM-41 sample shows characteristic peaks at 1223, 1059 and 790 cm−1 corresponding to stretching Si–O–Si and the peak at 454 cm−1 corresponding to bending Si–O–Si (Fig. 2(a)). The presence of the peak around 1600 cm−1 mainly from the NH2 symmetric bending vibration confirms the successful functionalization of the MCM-41 with 3-APTES (Fig. 2(b)). Signals at 1651 cm−1 (C
N bond) and 1520 cm−1 (C
C bond) for 4-hydroxy-3-methoxybenzaldehyde-functionalized on MCM-41 compared with the spectrum of the pure MCM-41 indicate that the vanillin have been attached successfully to the solid surface of mesoporous (Fig. 2(c)). The free ligand exhibits a ν(C
N) stretch at 1651 cm−1, while in the Ni(II)–Vanillin–Schiff base–MCM-41 this band is shifted to higher wave numbers and appears at 1664 cm−1, indicating the formation of Ni-ligand bond (Fig. 2(d)).
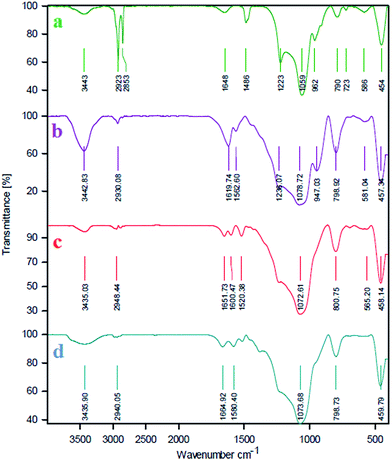 |
| Fig. 2 FT-IR spectra of (a) MCM-41(b) nPrNH2–MCM-41, (c) Vanillin–MCM-41 and (d) Ni(II)–Vanillin–Schiff base–MCM-41. | |
3.3. Scanning electron microscopy, inductively coupled plasma-optical emission spectrometry and energy dispersive spectrum
The morphology and size of the catalyst was evaluated using scanning electron microscopy (SEM). As can be seen in Fig. 3 the sample consists of uniform spherical particles with uniform nanometer size. To investigate the nickel(II) content in the anchored complex on MCM-41, the synthesized catalyst was analyzed using EDS. As shown in Fig. 4, the EDS spectrum of the catalyst showed the presence of Si (52.71%), C (7.52%), O (31.09%), N (4.66%), and Ni (4.02%) species in the catalyst (Fig. 4).
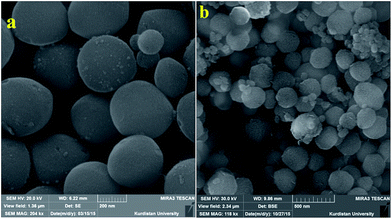 |
| Fig. 3 SEM images of (a) MCM-41 and (b) Ni(II)–Vanillin–Schiff base–MCM-41. | |
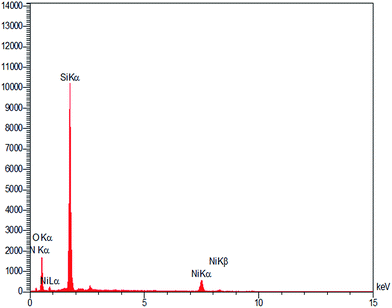 |
| Fig. 4 The EDS spectrum of Ni(II)–Vanillin–Schiff base–MCM-41. | |
Also, to extend the scope of catalyst characterization, we have determined the exact loading of Ni on MCM-41 by ICP-OES technique. The Ni amount of the immobilized catalyst on MCM-41 was found to be 2.6 × 10−4 mol g−1 or 15.26 mg g−1.
3.4. Transmission electron microscopy
The TEM micrographs of MCM-41 and Ni(II)–Vanillin–Schiff base–MCM-41 are shown in Fig. 5. Typical shapes of these materials with a regular hexagonal array of mesoporous channels can be clearly observed. The small nickel particles present in the catalyst cannot be located at the external surface of the support because there is not enough space for them. Therefore, this particle must be situated inside the pores of MCM-41.
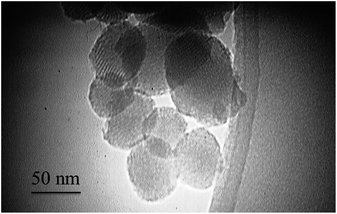 |
| Fig. 5 TEM micrographs of Ni(II)–Vanillin–Schiff base–MCM-41. | |
3.5. Thermogravimetric analysis
The TGA analysis data of MCM-41 and Ni(II)–Vanillin–Schiff base–MCM-41 catalyst are shown in Fig. 6. The curve of MCM-41 shows 4% weight loss which is due to desorption of water. In overall, the TGA curve of Ni(II)–Vanillin–Schiff base–MCM-41 shows three step weight losses. In the first step, Ni(II)–Vanillin–Schiff base–MCM-41 showed 10% weight loss between room temperature and 150 °C. This mass loss seems to be due to removal of adsorbed water, while the second mass loss at 150 to ∼250 °C is due to decomposition of the organically modified framework. Upon further heating, the TGA analysis data of Ni(II)–Vanillin–MCM-41 sample indicated the third weight loss (17%) at 280 to ∼500 °C is likely attributed to decomposition of silanol groups.27
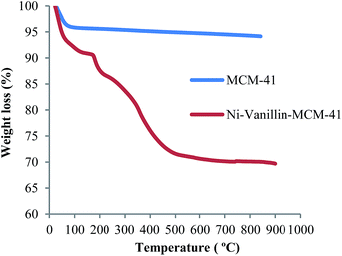 |
| Fig. 6 TGA curves of MCM-41 and Ni(II)–Vanillin–Schiff base–MCM-41. | |
3.6. N2 adsorption–desorption studies
The N2 adsorption and desorption isotherms of MCM-41 and Ni(II)–Vanillin–Schiff base–MCM-41 are shown in Fig. 7. The BET surface area of MCM-41 is 1372 m2 g−1 and the pore volume is 1.521 cm3 g−1. The average pore diameter is calculated to be 2.45 nm using BJH method. Ni(II)–Vanillin–Schiff base–MCM-41 shows smaller N2 uptake (BET surface area 265.117 m2 g−1), pore volume (0.744 cm3 g−1) and pore diameter (1.21 nm). We can conclude that Ni(II)–Vanillin–Schiff base–MCM-41 sample possess lower pore volume and pore size distributions compared to its parent material. A sharp inflection at about 0.3 relative pressures (P/P0), indicated a narrow pore size distribution for MCM-41 (Fig. 7(a)). With the isotherms of the mesoporous materials loaded with Ni(II) (Fig. 7(b)), the condensation step is shifted to lower P/P0 values while the total amount of N2 adsorbed decreases.23–25 These observations indicate that the immobilization of Ni complex has taken place substantially inside the pore channels of MCM-41 (Table 1).
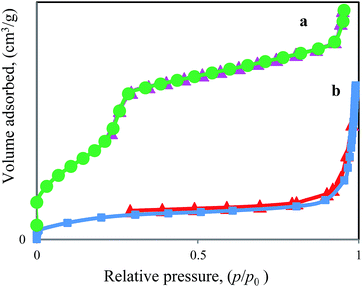 |
| Fig. 7 Adsorption/desorption isotherm for (a) bulk MCM-41, (b) Ni(II)–Vanillin–Schiff base–MCM-41. | |
Table 1 Surface properties of MCM-41 and Ni(II)–Vanillin–Schiff base–MCM-41
Sample |
SBET (m2 g−1) |
Pore diam. by BJH method (nm) |
Pore vol. (cm3 g−1) |
MCM-41 |
1372.275 |
2.45 |
1.521 |
Ni(II)–Vanillin–Schiff base–MCM-41 |
265.17 |
1.21 |
0.744 |
4 Evaluation of the catalytic activity of Ni(II)–Vanillin–Schiff base–MCM-41
4.1. Synthesis of 2,3-dihydroquinazolin-4(1H)-one derivatives
The heterogeneous Ni(II)–Vanillin–Schiff base–MCM-41 catalyst was applied from reaction of 4-chlorobenzaldehyde (1 mmol), and 2-aminobenzamide (1 mmol) as model reaction at the room temperature and the reaction parameters such as solvent and amount of catalyst were optimized. Initially, the model reaction was carried out in different solvents. Among them, H2O gave the best results (Table 2, entry 4). To find out the effect of the catalyst, blank experiment was carried out in the absence of catalyst at room temperature. It was observed that the reaction was very sluggish and only trace amount of product was formed after 5 h (Table 2, entry 8). However, when similar reaction was conducted in the presence of Ni(II)–Vanillin–MCM-41 (10 mg, 0.267 mol%), the reaction was completed yielding (94%) in 70 min (Table 2, entry 4).
Table 2 Optimization of the solvent, amount of catalyst for synthesis of 2,3-dihydroquinazolin-4(1H)-ones via the condensation of 4-chlorobenzaldehyde (1 mmol) with 2-aminobenzamide (1 mmol) as a model reaction at room temperature
Entry |
Solvent |
Catalyst (mg, mol%) |
Time (min) |
Yielda (%) |
Isolated yield. |
1 |
EtOH |
10, 0.267 |
70 |
57 |
2 |
DMF |
10, 0.267 |
70 |
50 |
3 |
1,4-Dioxane |
10, 0.267 |
70 |
35 |
4 |
H2O |
10, 0.267 |
70 |
94 |
5 |
H2O |
12, 0.320 |
70 |
94 |
6 |
H2O |
8, 0.213 |
70 |
84 |
7 |
H2O |
7, 0.187 |
70 |
70 |
8 |
H2O |
0 |
300 |
Trace |
On the bases of our optimized conditions we started our investigation with Ni(II)–Vanillin–Schiff base–MCM-41 catalyzed reaction of 2-aminobenzamide with various aldehydes and it was found that good to excellent yields of desired products were obtained (Table 3). The reaction was compatible with various functional groups such as electron donating groups (e.g. 4-methoxybenzaldehyde) (Table 3, entry 3) and electron withdrawing groups (e.g. 4-flouro benzaldehyde) (Table 3, entry 6) and all the reactions proceeded clean, to give corresponding 2,3-dihydroquinazolin-4(1H)-ones with no remarkable difference in their yields. In order to find out whether further surface modification of mesoporous MCM-41 by vanillin is required or not, the Ni(II) ions was directly immobilized onto nPrNH2–MCM-41 and used as catalyst (Ni(II)–MCM-41) in the synthesis of 2-(4-chlorophenyl)-2,3-dihydroquinazolin-4(1H)-one (instead of metal immobilized onto Vanillin–MCM-41). It was found that the reaction progress was very slow and after 24 h only 25% of desirable product was achieved (Table 3, entry 16).
Table 3 Synthesis of various 2,3-dihydroquinazolin-4(1H)-ones in the presence of Ni(II)–Vanillin–Schiff base–MCM-41 (10 mg, 0.267 mol%) in H2O at the room temperature
4.2. Synthesis of polyhydroquinoline derivatives
In this work, we also studied the synthesis of polyhydroquinoline derivatives from reaction of 4-chlorobenzaldehyde (1 mmol), dimedon (1 mmol), ethyl acetoacetate (1 mmol) and ammonium acetate (1.2 mmol) as a model reaction. Initially, the reaction was carried out in the absence of catalyst in water under heating condition for 25 h. However, reaction rate was very slow and product was obtained in lower yield (Table 4, entry 1). Hence, the reaction conditions for the one-pot synthesis of polyhydroquinoline derivatives was optimized for above mentioned reaction under the influence of different amounts of Ni(II)–Vanillin–MCM-41 as a reusable nanocatalyst in water at 80 °C. It was observed that the best amount of catalyst is 35 mg, (0.935 mol%) for this reaction (Table 4, entry 8). Moreover, this reaction was carried out in ethanol, DMF and 1,4 dioxane as solvents. As shown in Table 4, Ni(II)–Vanillin–Schiff base–MCM-41 is much more reactive in water compared with ethanol and other solvents (Table 4, entries 8–10). The reaction temperature was also optimized and 80 °C was chosen as reaction temperature (Table 4 entries 4, 8, 11, 12). Results of this study are summarized in Table 4.
Table 4 Optimization of amount of catalyst, solvent and temperature for the synthesis of polyhydroquinolines via the condensation of 4-chlorobenzaldehyde (1 mmol), dimedon (1 mmol), ethyl acetoacetate (1 mmol) and ammonium acetate (1.2 mmol) as a model reaction at 80 °C for 340 min
Entry |
Catalyst (mg, mol%) |
Solvent |
Temperature (°C) |
Time (min) |
Yielda (%) |
Isolated yield. |
1 |
None |
H2O |
80 |
1500 |
Trace |
2 |
5, 0.133 |
H2O |
80 |
340 |
30 |
3 |
10, 0.267 |
H2O |
80 |
340 |
45 |
4 |
15, 0.400 |
H2O |
80 |
340 |
53 |
5 |
20, 0.534 |
H2O |
80 |
340 |
68 |
6 |
25, 0.667 |
H2O |
80 |
340 |
75 |
7 |
30, 0.801 |
H2O |
80 |
340 |
84 |
8 |
35, 0.935 |
H2O |
80 |
340 |
93 |
9 |
35, 0.935 |
EtOH |
80 |
340 |
54 |
10 |
35, 0.935 |
EtOH(reflux) |
80 |
340 |
89 |
11 |
35, 0.935 |
H2O |
70 |
340 |
72 |
12 |
35, 0.935 |
H2O |
60 |
340 |
63 |
As shown in Table 5, a variety of aromatic and aliphatic aldehydes bearing electron-donating and electron-withdrawing substituents were successfully employed to prepare the corresponding polyhydroquinoline derivatives and it was observed that good to excellent yields of desired products were obtained. In this study, we also have used ethyl acetoacetate instead of dimedon for the synthesis of 1,4-dihydropyridines (Table 5, entries 13–15).
Table 5 Synthesis of various polyhydroquinolines in the presence of Ni(II)–Vanillin–Schiff base–MCM–41 (35 mg, 0.935 mol%) under the standard reaction conditions
4.3. Synthesis of 1H–indazolo[1,2-b]phthalazine-trione derivatives
In this research, we also report catalytic application of Ni(II)–Vanillin–Schiff base–MCM-41 as a green catalyst for the synthesis of 1H-indazolo[1,2-b]phthalazine-trione derivatives by a one-pot, three-component condensation of phthalhydrazide (1 mmol), para-ethoxy benzaldehyde (1 mmol), and dimedon (1 mmol) as a model reaction. In order to optimize the reaction conditions, we evaluated the influence of different amounts of catalyst at 100 °C in terms of product yield (Table 6). As shown in Table 6, when the catalytic amount of Ni(II)–Vanillin–Schiff base–MCM-41 was added, the yields of products were increased; however 10 mg of catalyst (0.267 mol%) was selected for the desired reaction (Table 6, entry 3). With respect to the solvent system, the best results were achieved under solvent-free conditions.
Table 6 Optimization of the conditions for three-component condensation of phthalhydrazide (1 mmol), para-ethoxy benzaldehyde (1 mmol), and dimedon (1 mmol) at 100 °C for 70 min
Entry |
Catalyst (mg, mol%) |
Solvent |
Time (min) |
Yielda (%) |
Isolated yield. |
1 |
None |
Solvent-free |
600 |
Trace |
2 |
8, 0.213 |
Solvent-free |
70 |
87 |
3 |
10, 0.267 |
Solvent-free |
70 |
94 |
4 |
12, 0.320 |
Solvent-free |
70 |
94 |
5 |
10, 0.267 |
H2O |
70 |
63 |
6 |
10, 0.267 |
EtOH |
70 |
50 |
In the following, we examined the generality of the reaction in the optimal reaction conditions with various aromatic and aliphatic aldehydes bearing either electron-donating or electron-withdrawing groups on the aromatic ring. In all cases, the reactions gave the corresponding products in short reaction times and good to excellent yields. The results are shown in Table 7.
Table 7 Synthesis of a series of 2H-indazolo[2,1-b]phthalazine-trione derivatives in the presence of Ni(II)–Vanillin–Schiff base–MCM-41 10 mg (0.267 mol%) under solvent-free conditions at 100 °C
The activity and recyclability of the prepared catalyst were tested. Fig. 8 shows the yield of five consecutive cycles for the preparation of 2,3-dihydroquinazolin-4(1H)-one (a), polyhydroquinolines (b) and 2H-indazolo [2,1-b]phthalazine-trione (c) with benzaldehyde. After completion of the reaction, the catalyst was separated by filtration, and then washed with EtOH and reused in the next run. The results showed that catalyst is recoverable and recycled for at least five times without significant loss of its catalytic activity (Fig. 8).
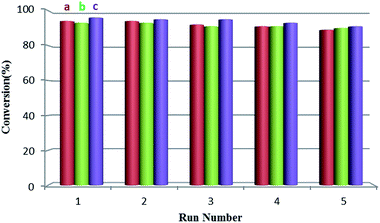 |
| Fig. 8 Reusability of catalyst in the preparation of 2,3-dihydroquinazolin-4(1H)-one (columns a), polyhydroquinolines (columns b) and 2H-indazolo[2,1-b]phthalazine-trione (columns c). | |
To test if metal was leached out from the solid catalyst during the reaction, hot filtration test was performed. For this aim, a mixture of 4-chlorobenzaldehyde (1 mmol), dimedone (1 mmol), ethylacetoacetate (1 mmol), ammonium acetate (1.2 mmol) and Ni(II)–Vanillin–Schiff base–MCM-41 in 3 mL of H2O was stirred at 80 °C. At this stage (in half time of the reaction), the catalyst was filtered off and the experiment was continued with the filtrate to complete reaction time. We found that the filtrate did not exhibit any detectable catalytic activity. In addition, Tech flame atomic absorption spectrometer (AAS) of the supernatant solution of the reaction mixture performed and results confirmed the absence of nickel ions in the liquid phase that indicated the prepared catalyst was truly act in heterogeneous manner.
5 Conclusions
In summary, we have demonstrated that Ni(II)–Vanillin–Schiff base-MCM-41 can be used as an inexpensive, green, non-hazardous, commercially available, efficient and reusable catalyst for the synthesis of a wide range of 2,3-dihydroquinazolin-4(1H)-one at room temperature and polyhydroquinoline derivatives at 80 °C in water as green solvent and 2H-indazolo[2,1-b]phthalazine-trione derivatives at 100 °C in solvent free conditions. This catalyst can be easily removed from the reaction mixture via simple filtration and reused several times with little loss of activity or metal leaching. The catalyst showed excellent stability and recyclability. The prepared complex was characterized by TGA, FTIR, BET, SEM, TEM, EDS, ICP-OES and XRD techniques.
Acknowledgements
This work was supported by the research facilities of Ilam University, Ilam, Iran.
References
- I. Mohammadpoor-Baltork, A. R. Khosropour, M. Moghadam, S. Tangestaninejad, V. Mirkhani, S. Baghersad and A. Mirjafari, C. R. Chim., 2011, 14, 1095 CrossRef.
- C. Gonzalez-Arellano, A. Corma, M. Iglesias and F. Sanchez, Adv. Synth. Catal., 2004, 346, 1758 CrossRef CAS.
- J. H. Clark and D. J. Macquarrie, in Handbook of Green Chemistry and Technology, Blackwell, Oxford, 2002 Search PubMed.
- P. T. Anastas, M. M. Kirchhoff and T. C. Williamson, Appl. Catal., A, 2001, 221, 3 CrossRef CAS.
-
(a) R. F. Heck, Org. React., 1982, 27, 345 CAS;
(b) A. F. Littke and G. C. Fu, Angew. Chem., Int. Ed., 2002, 41, 4176 CrossRef CAS.
- J. Beck, J. Vartuli, W. Roth, M. Leonowicz, C. Kresge, K. Schmitt, C. Chu, D. Olson, E. Sheppard, S. McCullen, J. Higgins and J. Schlenker, J. Am. Chem. Soc., 1992, 114, 10834 CrossRef CAS.
- N. Marın-Astorga, G. Pecchi, J. L. G. Fierro and P. Reyes, J. Mol. Catal. A: Chem., 2005, 231, 67 CrossRef.
- A. Ghorbani-Choghamarani, F. Nikpour, F. Ghorbani and F. Havasi, RSC Adv., 2015, 5, 33212 RSC.
- A. Ghorbani-Choghamarani, F. Nikpour, F. Ghorbani and F. Havasi, New J. Chem., 2015, 39, 6504 RSC.
- I. Ugi, B. Werner and A. Domling, Molecules, 2003, 8, 53 CrossRef.
- P. T. Anastas and J. C. Warner, Green Chemistry: Theory and Practice, Oxford University Press, New York, NY, USA, 1998 Search PubMed.
- J. Martínez, S. Romero-Vega, R. Abeja-Cruz, C. Álvarez-Toledano and R. Miranda, Int. J. Mol. Sci., 2013, 14, 2903 CrossRef PubMed.
- T. J. J. Müller Beilstein, J. Org. Chem., 2011, 7, 960 Search PubMed.
- M. Abdollahi-Alibeik and E. Shabani, Chin. Chem. Lett., 2011, 22, 1163 CAS.
- P. V. Murthy, D. Rambabu, G. R. Krishna, C. M. Reddy, K. R. S. Prasad, M. V. B. Rao and M. Pal, Tetrahedron Lett., 2012, 53, 863 CrossRef CAS.
- S. Rostamnia and F. Pourhassan, Chin. Chem. Lett., 2013, 24, 401 CrossRef CAS.
- Y. Chen, W. Shan, M. Lei and L. Hu, Tetrahedron Lett., 2012, 53, 5923 CrossRef CAS.
-
(a) V. Klusa, Bioenergetics, 1995, 20, 135 Search PubMed;
(b) A. Sausins and G. Duburs, Heterocycles, 1988, 27, 279 Search PubMed.
- B. V. S. Reddy, A. Venkateswarlu, C. Madan and A. Vinu, Tetrahedron Lett., 2011, 52, 1891 CrossRef.
- B. Atashkar, A. Rostami, H. Gholami and B. Tahmasbi, Res. Chem. Intermed., 2015, 41, 3675 CrossRef CAS.
- M. J. Hour, L. J. Huang, S. C. Kuo, Y. Xia, K. Bastow, Y. Nakanishi, E. Hamel and K. H. Lee, J. Med. Chem., 2000, 43, 4479 CrossRef CAS PubMed.
- S. Bhunia and S. Koner, Polyhedron, 2011, 30, 1857 CrossRef CAS.
- F. Havasi, A. Ghorbani-Choghamarani and F. Nikpour, Microporous Mesoporous Mater., 2016, 224, 26 CrossRef CAS.
- M. Nikoorazm, A. Ghorbani-Choghamarani, H. Mahdavi and S. M. Esmaeili, Microporous Mesoporous Mater., 2015, 211, 174 CrossRef CAS.
- Y. Gang, C. Xing, W. Xiaoli, X. Weihong and X. Nanping, Chin. J. Catal., 2013, 34, 1326 CrossRef.
- M. Nikoorazm, A. Ghorbani-Choghamarani and N. Noori, Appl. Organomet. Chem., 2015, 29, 328 CrossRef CAS.
- M. Nikoorazm, A. Ghorbani-Choghamarani and M. Khanmoradi, Appl. Organomet. Chem., 2016, 30, 236 CrossRef CAS.
- M. Nikoorazm, A. Ghorbani-Choghamarani, H. Mahdavi and Z. Karamshahi, J. Porous Mater., 2015, 22, 261 CrossRef CAS.
- Y. Gang, C. Xing, W. Xiaoli, X. Weihong and X. Nanping, Chin. J. Catal., 2013, 34, 1326 CrossRef.
- A. Ghorbani-Choghamarani, Z. Darvishnejad and B. Tahmasbi, Inorg. Chim. Acta, 2015, 435, 223 CrossRef CAS.
- D. J. Lensvld, J. G. Mesu, A. J. Dillen and K. P. Jong, Microporous Mesoporous Mater., 2001, 44, 401 CrossRef.
- D. Liu, X. Y. Quek, W. N. Evelyn Cheo, R. Lau, A. Borgna and Y. Yang, J. Catal., 2009, 266, 380 CrossRef CAS.
- X. Chen, J. Jiang, F. Yan, S. Tiana and K. Li, RSC Adv., 2014, 4, 8703 RSC.
- M. Hajjami and B. Tahmasbi, RSC Adv., 2015, 5, 59194 RSC.
- A. V. Dhanunjaya Rao, B. P. Vykunteswararao, T. Bhaskarkumar, N. R. jogdand, D. Kalita, J. K. D. Lililakar, V. Siddaiah, P. D. Sanasi and A. Raghunadh, Tetrahedron Lett., 2015, 56, 4714 CrossRef CAS.
- A. Ghorbani-Choghamarani and M. Norouzi, J. Mol. Catal. A: Chem., 2014, 395, 172 CrossRef CAS.
- A. Ghorbani-Choghamarani, Z. Darvishnejad and M. Norouzi, Appl. Organomet. Chem., 2015, 29, 707 CrossRef CAS.
- M. Tajbakhsh, H. Alinezhad, M. Norouzi, S. Baghery and M. Akbari, J. Mol. Liq., 2013, 177, 44 CrossRef CAS.
- A. Ghorbani-Choghamarani, M. A. Zolfigol, M. Hajjami, H. Goudarziafshar, M. Nikoorazm, S. Yousefi and B. Tahmasbi, J. Braz. Chem. Soc., 2011, 22, 525 CrossRef CAS.
- M. V. Reddy, G. C. S. Reddy and Y. T. Jeong, Tetrahedron, 2012, 68, 6820 CrossRef.
- R. Ghorbani-Vaghei, R. Karimi-Nami, Z. Toghraei-Semiromi, M. Amiri and M. Ghavidel, Tetrahedron, 2011, 67, 1930 CrossRef CAS.
- A. Rostami, B. Tahmasbi and A. Yari, Bull. Korean Chem. Soc., 2013, 34, 1521 CrossRef CAS.
- H. Shaterian, M. Ghashang and M. Feyzi, Appl. Catal., A, 2008, 345, 128 CrossRef CAS.
|
This journal is © The Royal Society of Chemistry 2016 |
Click here to see how this site uses Cookies. View our privacy policy here.