DOI:
10.1039/C6RA09877K
(Paper)
RSC Adv., 2016,
6, 67693-67700
Selective chromo-fluorogenic molecular sensor for dual channel recognition of Cu2+ and F−: effect of functional group on selectivity†
Received
16th April 2016
, Accepted 27th June 2016
First published on 29th June 2016
Abstract
The sensor HNHCB (3-hydroxy-naphthalene-2-carboxylic acid (4-cyano-benzylidene)-hydrazide) comprises a –CONH– group, –OH group, –CH
N– linkage and an electron withdrawing –CN group that can act as both an anion (fluoride) and a cation (copper) sensor by two distinct output modes. It can detect fluoride by naked eye color change and copper by fluorescence enhancement. Interestingly, the nature of the substituent in HNHCB has an effect on the selectivity. The detection limit can be as low as 160 nM for Cu2+ and 1.3 μM for F−. DFT calculations have been performed to demonstrate the structure of HNHCB and its copper complex. Biological applications of HNHCB have been evaluated in HEK 293 and it was found to exhibit membrane permeability for the detection of Cu2+. The sensor HNHCB is also sensitive towards fluoride in commercially available toothpaste.
1. Introduction
For recent years, the design of new molecular systems for the detection of biologically important cations, anions or neutral molecules has been the spotlight of modern research in the field of chemo and biosensors.1–3 To date, a huge number of single ion responsive sensors that are selective for cations or anions have been reported.4,5 At present, scientists are focused to develop single molecular systems that have multi-ion sensing ability with different output detection responses. In this regard, some molecular systems containing –OH, –NH–, –NH2, urea, thiourea and –CONH– centers have been reported, which act as sensors for both cations and anions, for example, Hg2+/ClO−,6 Hg2+/F−,7 and Cu2+/F−.8 In particular, the sensing of fluoride and copper ion has attracted growing attention due to their positive and negative impacts on human health and environment.
Fluoride is a very essential, as well as a toxic element for the human body. It can influence some medical and chemical processes such as dental care and osteoporosis treatment.9–11 On the contrary, a high intake of fluoride causes fluorosis, depression of thyroid activity, bone disorder, urolithiasis, stomach ulcers, or even cancer.12–14 On the other hand, copper is also a vital element in human physiology, but overdose or deficiency of it causes serious neurodegenerative diseases such as Menkes and Wilson diseases, Alzheimer's disease, and prion diseases.15,16 Thus, it is necessary to develop selective, sensitive and facile methods for the detection of both copper and fluoride in physiological conditions.
To date, some molecular probes have been reported as the sensor for both copper and fluoride ions. According to previous literature reports, most of the dual sensors detect both copper and fluoride ions by a single output mode. They detect copper and fluoride ions either by naked eye color change/UV-Vis spectral change or by fluorescence spectral changes. Recently, a research group have reported a 1,8-naphthalimide-based colorimetric sensor that detects Cu2+ by naked eye color change from red to colorless and F− from red to blue separately.17 Another group have reported a thiourea based sensor that detects fluoride by a color change from colorless to yellow/brown and copper from colorless to violet/bluish green.17 In addition, a julolidine moiety based sensor detects Cu2+ by a color change from colorless to yellow and F− by a color change from colorless to yellow.17 Therefore, in all the abovementioned cases, the sensors show a distinct color change when they are treated separately with copper and fluoride ions. In such cases, the problem is when both copper and fluoride ions are present together, there will be no way to separately distinguish between each ion within the mixture. There are also some fluorimetric sensors in the literature, which detect Cu2+ and F− by changes of their own fluorescence profile. For example, a phenanthrene-based sensor detects both the Cu2+ and F− separately by fluorescence quenching.18 Again, a dansyl-antipyrine derived sensor detects Cu2+ by fluorescence quenching and F− by ratiometric fluoroscence quenching.18 Another group has reported a pyrene based sensor that detects both the Cu2+ and F− by enhancement of its own fluorescence intensity.18 In these cases, the sensors show either an increase or decrease of emission intensity in the presence of Cu2+ and F−. Again, the same problem arises, i.e., they will not be able to distinguish Cu2+ and F− separately in their mixture. Herein, we have reported an easily synthesized molecular probe (HNHCB) having a dual mode of output signals for F− and Cu2+ in semi-aqueous mediums. The sensor detects Cu2+ by fluorescence enhancement and F− by a color change from colorless to yellow. Most interestingly, like other literature reports,8 our sensor can detect copper ion selectively by fluorimetric experiment in presence of all cations and anions mixture (including copper and fluoride ion), where F− does not interfere and it can also detect F− selectively by naked-eye experiment in the mixture ions wherein Cu2+ does not interfere. In addition, we have shown the effect of the functional group on the selectivity of the sensor towards cations and anions. It is noteworthy to mention that our sensor can detect Cu2+ in the living cell and it can detect F− in commercially available toothpaste as well.
2. Experimental
2.1. Instrumentation
Electronic absorption spectra and fluorescence spectra have been obtained by a Hitachi UV-Vis (Model U-3501) and Perkin Elmer LS-55 spectrofluorometer, respectively. IR spectra (KBr pellet, 4000–400 cm−1) have been obtained on a Parkin Elmer modal 883 infrared spectrophotometer. 1H NMR spectra have been obtained on a Bruker, Avance 300 spectrometer, wherein chemical shifts (δ in ppm) have been determined with respect to tetramethylsilane (TMS) as internal standards. Mass spectra have been obtained on Waters Xevo G2-S Q TOF mass spectrometer.
2.2. Reagents
All reagents and solvents have been used as received from commercial sources without further purification. All anions in the form of tetrabutylammonium salts and all cations in the form of perchlorate salts have been purchased from Sigma-Aldrich Chemical Company. All solvents used for the spectroscopic studies are spectroscopic grade. Herein, all the spectroscopic experiments have been performed in an acetonitrile–water mixture (CH3CN
:
H2O = 7
:
3, v/v, pH = 7.2). The pH of this mixture has been maintained using a Tris–HCl buffer.
2.3. Syntheses
3-Hydroxy-naphthalene-2-carboxylic acid hydrazide has been synthesized from 3-hydroxy-naphthalene-2-carboxylic acid ethyl ester according to the literature procedure.19,20
2.3.1. Compound 1.
2.3.1.1 Synthesis of 3-hydroxy-naphthalene-2-carboxylic acid(4-cyano-benzylidene)-hydrazide (HNHCB). Compound 3-hydroxy-naphthalene-2-carboxylic acid hydrazide (1.5 mmol, 0.30 g) has been treated with 4-formyl benzonitrile (1.5 mmol, 0.20 g) in methanol at room temperature for 30 minutes to form a very light yellow solid. The product thus obtained has been filtered and then dried under vacuum (yield: 0.35 g, 76%). 1H NMR in d6-DMSO, 300 MHz, δ (ppm): 12.15 (s, 1H, –CONH–), 11.25 (s, 1H, –OH), 8.51 (s, 1H, naph), 8.44 (s, 1H, –CH
N–), 7.96–7.93 (m, 6H), 7.78 (d, J = 7.5 Hz, 1H), 7.54–7.35 (m, 2H). 13C NMR (75.5 MHz, d6-DMSO, 20 °C) δ (ppm): 111, 120.77, 124.30, 126.24, 127.23, 128.74, 129.11, 129.33, 130.75, 134.50, 136.27, 149.08, 154.36, 164.27, 176. IR (KBr): 3254, 3067, 2913, 2224, 1664, 1648, 1627, 1542, 1503, 1449, 1358, 1325, 1215, 1176, 1070 cm−1. Mass: (TOF MS ES+) m/z: calcd for C19H13N3O2 [M + H]+, 316.11; found, 316.10.
3. Results and discussion
The compound HNHCB has been synthesized following two steps (Scheme S1†). In the first step, acid hydrazide has been prepared from the corresponding ethyl ester and hydrazine and then it has been condensed with 4-cyano benzaldehyde. The compound HNHCB has been characterized by 1H, 13C NMR, Mass and IR spectroscopic methods. The FTIR spectroscopy obtains information about the presence of two functional groups (amido (–CONH–) and imino (–CH
N–) group) by the absorption peaks at 1664 and 1627 cm−1, respectively.21 Compounds HNHCB shows strong absorption peaks at 2224 cm−1 corresponding to the –CN group. In the 1H-NMR spectrum, two characteristic peaks at 12.16 ppm and 8.44 ppm have been found, which are nothing but the peaks of (–CONH–) and imino (–CH
N–) groups, respectively.
3.1. Visual sensing of anions
Literature reports show that compounds having both the amide and phenolic –OH groups have a certain affinity towards anions.22 Therefore, the colorimetric sensing ability of compound HNHCB (10−5 M) towards anions has been investigated in acetonitrile water mixture (7
:
3, v/v). When a solution of F− ion is added to the solution of HNHCB, an intense yellow coloration occurs, which can be visualized by the naked eye (Fig. 1). Under similar experimental conditions, acetate ions can also exhibit a similar type of colour change, demonstrating that compound HNHCB can detect both fluoride and acetate ions by the naked eye. However, we can modulate the selectivity of compound HNHCB by slight modification of its structure by incorporating –H in place of –OH to form compound NHCB (ESI, Scheme S1†). Compound NHCB exhibits a yellow colour change only in the presence of fluoride ions, but not for acetate ions (ESI, Fig. S8†). Moreover, to understand the sensing mechanism, we have synthesized compound HNHB without a –CN group than that of HNHCB (ESI, Scheme S1†), which does not show any color change upon treatment of an acetonitrile solution of F− and OAc− ions compared to the aqueous acetonitrile solution of HNHB (1.0 × 10−5 M). Therefore, the colour change of compound HNHCB is the oblivious result of a subsequent intramolecular charge-transfer from the electron rich anion-bound naphthanol amide to the electron deficient cyanobenzene moiety due to hydrogen bonding interaction/deprotonation between the hydroxyl (–OH) group, amide group and anions.23–25 In the case of compound NHCB containing no –OH group, fluoride ions show color changes due to hydrogen bonding interactions/deprotonation with the amide group leading to the abovementioned intramolecular charge transfer process. However, acetate does not show any color change because the basicity of acetate is comparatively less than fluoride. Therefore, it may not be able to interact/deprotonate the amidic protons.
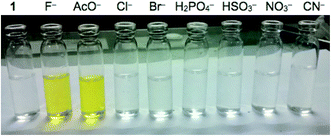 |
| Fig. 1 Visual color changes of HNHCB (1) (1.0 × 10−5 M) after addition of 2 equivalent of various anions in acetonitrile water mixture (CH3CN : H2O = 7 : 3, v/v). | |
Under the same experimental condition, other ions, such as H2PO4−, Cl−, Br−, HSO3−, NO3− and CN−, do not exhibit any visual color changes with any of the compounds. Based on the abovementioned observations, it can be said that compound HNHCB can detect both fluoride and acetate ions and NHCB can only detect fluoride ions selectively by the naked eye in acetonitrile water mixtures.
3.2. UV-Vis spectroscopic titration
The anion binding ability of compound HNHCB has also been monitored by UV-Visible spectroscopy in aqueous acetonitrile solutions. Fig. 2 shows that compound HNHCB exhibits three absorption bands at 226 nm, 319 nm and 376 nm with a shoulder at around 291 nm. Upon addition of increasing amount of F− ion into the aqueous acetonitrile solution of HNHCB (10−6 M), the bands at 226 nm and 319 nm gradually decrease with a red shift up to 239 nm and 346 nm, respectively. On the other hand, the band intensity at 376 nm gradually increases, indicating the formation of an H-bonded complex between the hydroxylic (–OH) and amide protons of the compound HNHCB and F− ion followed by deprotonation, leading to an intramolecular charge transfer from naphthanol amide moiety to the cyano-benzene ring. The abovementioned absorption changes occur with three clear isosbestic points at 233 nm, 270 nm and 335 nm. These spectral changes are accompanied by a visual color change from colorless to yellow. Under similar experimental conditions, a similar type of spectral changes occurs upon addition of AcO− ion, which indicates a like interaction with HNHCB (ESI, Fig. S9†).
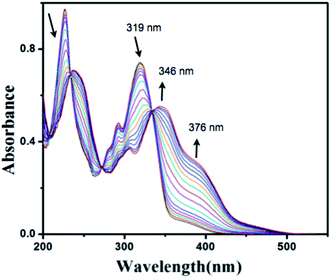 |
| Fig. 2 UV-Vis spectral changes of HNHCB (1.0 × 10−6 M) upon addition of F− ion (0–5 equiv.) in acetonitrile water mixture (CH3CN : H2O = 7 : 3, v/v). | |
Like the naked eye experiment, UV-Vis spectral studies have also been performed with compound NHCB in an aqueous acetonitrile solution. Compound NHCB shows two bands at 221 nm and 314 nm (ESI, Fig. S10†). As expected, compound NHCB exhibits a change in spectral properties with the addition of fluoride only, implying selectivity of NHCB towards the fluoride ion (ESI, Fig. S10 and S11†). Again, compound HNHB does not show any type of spectral modification in the presence of fluoride or acetate (ESI, Fig. S12†).
Under the same experimental conditions, the addition of other anions, such as CN−, H2PO4−, Cl−, Br−, NO3− and HSO3−, have observed no notable spectral or colour changes with HNHCB and NHCB, indicating that no interaction or complexation of these anions with the abovementioned compounds (ESI, Fig. S13†).
3.3. 1H NMR titration
To investigate the nature of binding between the compound HNHCB and fluoride, 1H NMR titration experiments have been performed in d6-DMSO. 1H NMR spectra of HNHCB (Fig. 3) shows the characteristic peak of amide proton (Hb), hydroxylic (Ha) proton and imine (Hd) proton at δ 12.16, 11.25 and 8.44 ppm, respectively. During titration, when 1 equivalent of TBAF has been added, the –NH peak and –OH peak become broader due to H-bonding interactions. On the other hand, the Hc and He protons of the naphthaline ring undergo an upfield shift. Upon further addition of 2 equivalents of F ions, both the –NH and –OH peaks disappear and all the aromatic peaks get separated and appear clearly. At 3 equivalent of F− ions, the Hc proton is shifted further upfield compared to the imine proton Hd. The upfield shift of Hc and He protons of the naphthaline ring may be explained in terms of the deprotonation of the amide and hydroxylic groups in the presence of the fluoride ion (ESI, Scheme SII†).26,27
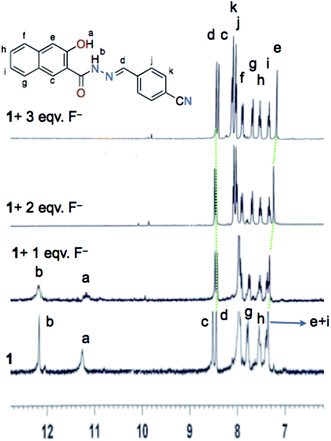 |
| Fig. 3 1H NMR spectra of HNHCB (1) in DMSO-d6 after addition of 0–3 equivalents of fluoride ion. | |
The abovementioned results of 1H NMR titration indicates that the binding mechanism of the compound with fluoride may be due to the H-bonding interaction with –NH– and –OH protons followed by deprotonation, which increases the electron density over both the naphthalene moiety and the nitrogen from the amide group. As a result, charge separation is introduced into the compound that triggers the intramolecular charge transfer (ICT) between the electron deficient –CN group and electron rich –N of the amide group, which shows as the UV-Vis and colorimetric changes.
3.4. Detection of copper
As our designed compound HNHCB contains –OH, imine and –CONH– groups as binding sites, it may also bind with cations. Taking this fact in mind, the binding abilities of compound HNHCB with various metal ions have also been investigated by naked eye, UV-Vis and fluoroscence spectroscopy. It does not show any visual color change in the presence of any cations. Therefore, we have performed UV-Vis and fluoroscence spectroscopic experiments in the presence of cations.
3.5. UV-Vis spectroscopic experiment
The UV-Vis spectral pattern of compound HNHCB has been described earlier. No such distinct spectral changes have been observed with the addition of metal ions (such as Fe3+, Cr3+, Co2+, Ni2+, Cu2+, Zn2+, Cd2+, Fe2+, Hg2+, Pb2+, Ca2+, Mg2+ and Mn2+) except Cu2+, which shows slight modification of the UV-Vis spectrum of the compound 1 (ESI, Fig S14†).
3.6. Spectrofluorometric experiment
The fluorescence properties of compound HNHCB have been investigated upon addition of various transition metal ions, such as Fe3+, Cr3+, Co2+, Ni2+, Cu2+, Zn2+, Cd2+, Fe2+, Hg2+, Pb2+, Ca2+, Mg2+ and Mn2+, in acetonitrile water mixture (CH3CN
:
H2O = 7
:
3, v/v). Upon excitation at 330 nm, HNHCB shows an emission band at 440 nm. When different metal ions have been added to HNHCB, fluorescence enhancement occurs only for Cu2+ (ESI, Fig. S15†). As shown in Fig. 4, upon addition of Cu2+ ion, the emission peak intensity gradually increases at 440 nm, which is attributed to the formation of a comparatively rigid structure imposed on the HNHCB by strong complexation with Cu2+ ion. The rigid structural architecture of HNHCB in the complex restricts the free motion of flexible bonds, thereby minimizing the radiationless transition, which consequently increases the fluorescence intensity.28,29 The Benesi–Hildebrand (B–H) plot of I/I0 vs. [Cu2+] for the fluorescence titration of HNHCB and Cu2+ ion provides a straight line, indicating a 1
:
1 complex formation with an association constant (K) 6.72 × 103 M−1 (ESI, Fig. S16†).
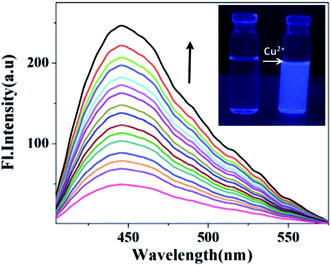 |
| Fig. 4 Fluorescence spectral changes of HNHCB (1.0 × 10−6 M) upon addition of Cu2+ ion (0–5 equiv.) in acetonitrile water mixture (CH3CN : H2O = 7 : 3, v/v). (Inset) The color change of HNHCB in acetonitrile water mixture (CH3CN : H2O = 7 : 3, v/v) without and with addition of Cu(ClO4)2 (1.0 × 10−4 M) under UV light. | |
The fluorescence intensity of HNHCB in the presence and absence of Cu2+ at various pH values has also been monitored. It has been found that in the absence of Cu2+, a change in pH hardly affects the fluorescence intensity of HNHCB. When Cu2+ has been added, HNHCB only shows a weak fluorescence response to Cu2+ in the acidic environment due to protonation of the donor sites of HNHCB. However, in the pH range from 5.5 to 7.7, it shows an appreciable fluorescence enhancement and a maximum fluorescence intensity is observed at pH = 7.2 (ESI, Fig. S17†).
To understand the sensing mechanism of HNHCB towards Cu2+, we have performed the abovementioned experiment under similar conditions with compounds NHCB and HNHB. Between NHCB and HNHB, only HNHB shows a response towards Cu2+ by fluorescence enhancement. Upon excitation at 300 nm, compound HNHB exhibits an emission band at 420 nm, which gradually increases with the increasing concentration of Cu2+ ion concentration. The presence of –OH group may be responsible for Cu2+ sensing (ESI, Fig. S18†). Interestingly, under UV light, the solution of HNHCB has no such noticeable fluorescence, whereas in the presence of Cu2+ a bluish white colored fluorescence has been observed in an acetonitrile water mixture (Fig. 4, inset). From the two aforesaid experiments, it can be said that senor HNHCB can detect Cu2+ in aqueous acetonitrile mixtures.
3.7. Fluorescence lifetime measurements
To investigate the local environment of the fluorophore and its excited state behaviour, fluorescence lifetime measurements of compound HNHCB have been performed in an acetonitrile water mixture at λex = 336 nm.30–32 The decay behavior of the bare fluorophore is found to be complicated and is best fitted to tri-exponential functions (Fig. 5, ESI Table S1†). With the addition of Cu2+ the relative abundance, as well as the life time of the first component (highest contribution) increases. For the second component, the lifetime increases but its relative abundance decreases and for the third component (lowest contribution), both the relative abundance as well as the lifetime decreases slightly. By averaging these values, it has been found that the average lifetime of HNHCB increases with the addition of Cu2+. This can be explained in terms of complexation of HNHCB with Cu2+ that gives more structural rigidity to the HNHCB, thereby minimizing the radiationless transition, which consequently increases the excited state lifetime of HNHCB. Increase of fluorescence intensity and the corresponding lifetime is quite obvious during complexation due to the lowering of non-radiative channels generated by the rigid structure of the complex than the comparatively more flexible structure of the bare ligand (flexible bonds promote non-radiative decay through low-frequency large-amplitude motion).
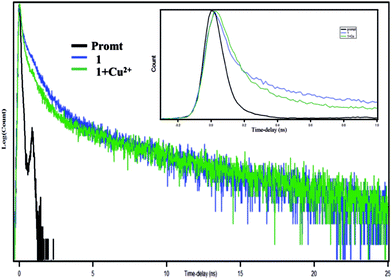 |
| Fig. 5 Fluorescence decay profile of HNHCB (1) and HNHCB (1) in presence of 2 equivalents of Cu2+, λex = 336 nm, λem(max) = 450 nm. (Inset) count vs. time–delay plot. | |
3.8. Kinetic study of HNHCB–Cu2+ association
The metal ligand interaction kinetics is considered to have crucial diagnostic significance. The kinetics of the HNHCB–Cu2+ association reaction has been studied by monitoring the enhancement of the fluorescence of HNHCB at 442 nm (λem) upon interaction with Cu2+ at 25 °C. The fluorescence trace has been fitted by an exponential increase function according to a pseudo-first order kinetics model wherein the concentration of Cu2+ is excess with respect to HNHCB.33 It has been found that the rate constant of association kinetics of HNHCB with Cu2+ is found to be 6 × 10−2 s−1 at 298 K. Furthermore, the association has been completed within 7 min (Fig. 6). Therefore, this system could be used for real-time tracking of Cu2+.
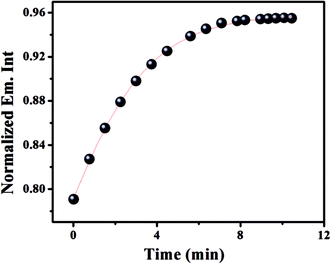 |
| Fig. 6 Reaction-time profile: changes of emission intensity of HNHCB (0.1 μM) at 442 nm in the presence of 4 equivalents of Cu2+ as a function of time (0.0–10.0 min). | |
3.9. Molecular simulation
To realize the nature of bonding interaction of HNHCB with Cu2+, theoretical structural calculations were preformed for compound HNHCB using Gaussian 03 software with a B3LYP-hybride functional and a 6-31++G(d,p) basis set and for Cu2+, a B3LYP with a LANL2DZ relativistic pseudopotential basis set at Density Functional Theory level.34 The optimized geometries of HNHCB and the [HNHCB–Cu2+] complex are shown in (ESI, Fig. S19†). The optimized global minima of compound HNHCB shows that both the naphthalene and benzene ring are in same plane and it exists in the open form wherein the phenolic –OH is not intramolecularly H-bonded with an imine nitrogen. In the complex, the Cu2+ ion binds to HNHCB very well through oxygen and nitrogen, and the whole molecular system forms a near planar structure. The optimized structure of the complex compound HNHCB is associated with two bonds (O–Cu and N–Cu) to the central ion with their distances being 1.70 and 1.65 Å, respectively (ESI Table S2†). The spatial distributions and orbital energies of the HOMO and LUMO of HNHCB and [HNHCB–Cu2+] complex have been also determined. The energy gaps between HOMO and LUMO in the compound HNHCB and the [HNHCB–Cu] complex are 2.41 eV and 1.856 eV, respectively, which clearly shows that the [HNHCB–Cu] complex has sufficient stability compared to the bare ligand (Fig. 7).
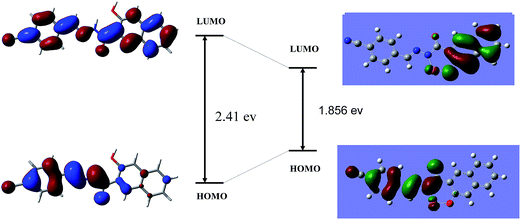 |
| Fig. 7 HOMO and LUMO distributions of HNHCB and HNHCB–Cu2+ complex. | |
3.10. Selectivity and sensitivity of the senor HNHCB towards Cu2+, F− and OAc−
From the abovementioned experimental analysis, it has been found that compound HNHCB can detect Cu2+ by fluorescence enhancement and F−/OAc− by visual color change. Moreover, to find out the selectivity of HNHCB towards Cu2+, an emission study has been performed in an aqueous acetonitrile mixture (acetonitrile
:
water = 7
:
3) in presence of other competing metal ions as well as an ion mixture (mixture of cations and anions including Cu2+). It has been found that the fluorescence intensity of the complex is hardly affected by other coexistent metal ions (ESI Fig. S20†). Again, the emission spectra show that HNHCB in the presence of an ion mixture still exhibits an emission band at 440 nm (ESI Fig. S21†), indicating the interaction of HNHCB with Cu2+. It is noteworthy to mention that the fluorescence intensity of HNHCB in the presence of an ion mixture is relatively lower than the fluorescence intensity of HNHCB in the presence of Cu2+ only. The selectivity of HNHCB towards fluoride has again been investigated in presence of other relevant anions and also in ion mixtures. As displayed in Fig. S22,† all the relevant anions tested have virtually no influence on the naked-eye detection of F− except OAc−. In the presence of ion mixtures, the existence of the 380 nm band in the UV-spectra indicates the selectivity of sensor HNHCB towards F− ion in presence of copper (ESI Fig. S23†). On the other hand, compound HNHCB is inefficient to detect OAc− ion in presence of Cu2+ in an aqueous acetonitrile (ESI Fig. S24†). Therefore, compound HNHCB can be used as a selective dual sensor for both Cu2+ and F− in a semi-aqueous medium. The detection limit of sensor HNHCB for both Cu2+ and F− has been calculated using fluorescence and UV-Visible titration data, respectively (ESI Fig. S25 and S26†).35 Sensor HNHCB has been found to have a detection limit of 160 nM for Cu2+ ion and 1.3 μM for F− in a semi aqueous solvent system.
3.11. The preliminary application of HNHCB
3.11.1. Biological studies of HNHCB in presence of Cu2+. The potential sensing ability of compound HNHCB towards Cu2+ ion by emission intensity enhancement in the visible region insists us for its practical bio-imaging application. The viability of HEK 293 cells has not been influenced by the amount of solvent (5% DMSO), which has been used for the experiment. Confocal microscopic studies revealed that compound HNHCB shows negligible fluorescence in HEK 293 cells (Fig. 8). Upon incubation with Cu2+ followed by compound HNHCB, fluorescence enhancement has been observed inside HEK 293 cells, indicating the formation of HNHCB–Cu2+ complex that collaborates well with the solution phase study. The confocal microscopic analysis suggests that compound HNHCB can rapidly sense intracellular Cu2+ by readily crossing the membrane barrier of the HEK 293 cells. Thus, HNHCB can be a suitable fluorescence chemosensor for Cu2+ detection in biological systems.
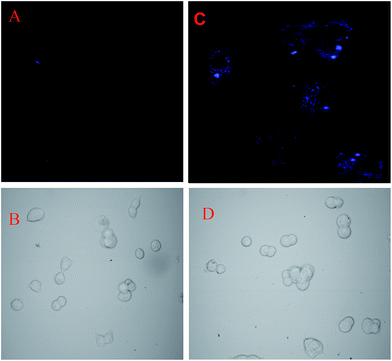 |
| Fig. 8 Confocal microscopic images of HNHCB in HEK 293 cells pretreated with Cu2+: (A) HNHCB (1 × 10−6 M), (B) bright field image of HNHCB (1 × 10−6 M), (C) HNHCB (1 × 10−6 M) in presence of Cu2+ (1 × 10−4 M), and (D) bright field image of HNHCB (1 × 10−6 M) in presence of Cu2+ (1 × 10−4 M). | |
3.11.2. Detection of fluoride in toothpaste by the sensor (HNHCB). From the abovementioned experiments, it has been found that compound HNHCB can quantitatively detect F− in a very wide concentration range. In this connection, we want to check the sensitivity of HNHCB towards fluoride ions in commercially available toothpaste. For this purpose, an aqueous acetonitrile solution of commercially available toothpaste was prepared as sample. On addition of the toothpaste solution to the aqueous acetonitrile solution of compound HNHCB (1 × 10−4 M), a deep yellow color generates, which is comparable to the color of the receptor in presence of bare fluoride (ESI Fig. S27,† inset). The UV-Vis spectra of compound HNHCB (1 × 10−5 M) in the presence of the toothpaste solution shows a broad band at 400 nm, which is comparable to the UV-Vis spectra of sensor HNHCB in presence of bare fluoride (ESI Fig. S27†). Thus, sensor HNHCB can detect F− in commercially available toothpaste.
3.11.3. Visual color changes on test papers. By considering the abovementioned observations, we have tried to investigate another practical applications of compounds HNHCB as an anion sensor. To explore this, we have prepared test kits by coating a test paper (Whatman-40) with aqueous CH3CN solution of HNHCB (1 × 10−4 M) and then dried it in air. For the detection of an anion, the aqueous solution of anions (1 × 10−4 M) were added on to the test paper and dried in hot air. Interestingly, the spot that contains F− ion shows a bright yellow color and no color changes has been observed in other spots containing anions, such as Cl−, Br−, I−, HSO3− and H2PO4−, except OAc−, which shows a faint yellow spot on the test paper (ESI, Fig. S28†). This experiment shows that compounds HNHCB can selectively detect the F− ion in both the solution and solid state.
4. Conclusion
In summary, we have synthesized an Amido-Schiff base HNHCB, which can act as a highly sensitive and selective bi-functional probe for the individual detection of Cu2+ and F−. It exhibits a naked eye colour change from colourless to yellow in presence of fluoride and shows fluoroscence enhancement by the interaction with Cu2+. The biological applications of HNHCB have been evaluated in HEK 293 and it was found to exhibit good membrane permeability for the detection of Cu2+. The senor HNHCB can also detect fluoride in both commercially available toothpaste and the solid state. On the basis of the abovementioned results, we believe that compound HNHCB will offer an important guidance for the development of single receptors to recognize both anions and cations via two different output modes of detection.
Acknowledgements
SG, AG & AB would like to acknowledge UGC and CSIR for Fellowship.
References
- T. Mayr, I. Klimant, O. S. Wolfbeis and T. Werner, Anal. Chim. Acta, 2002, 462, 1–10 CrossRef CAS.
- V. K. Gupta, A. K. Jain, G. Maheshwari, H. Langb and Z. Ishtaiwi, Sens. Actuators, B, 2006, 117, 99–106 CrossRef CAS.
- H. S. Jung, X. Chen, J. S. Kim and J. Yoon, Chem. Soc. Rev., 2013, 42, 6019–6031 RSC.
- S. Ghosh, M. A. Alam, A. Ganguly and N. Guchhait, Spectrochim. Acta, Part A, 2015, 149, 869–874 CrossRef CAS PubMed.
- S. Dalapati, S. Jana, M. A. Alam and N. Guchhait, Sens. Actuators, B, 2011, 160, 1106–1111 CrossRef CAS.
- K. Chen, J. W. Bats and M. Schmittel, Inorg. Chem., 2013, 52, 12863–12865 CrossRef CAS PubMed.
-
(a) N. Kumari, N. Dey and S. Bhattacharya, Analyst, 2014, 139, 2370–2378 RSC;
(b) A. Balamurugan and H. Lee, Sens. Actuators, B, 2015, 216, 80–85 CrossRef CAS;
(c) J.-Y. Lee, B. A. Rao, J.-Y. Hwang and Y.-A. Son, Sens. Actuators, B, 2015, 220, 1070–1085 CrossRef CAS.
-
(a) Y. Peng, Y.-M. Dong, M. Dong and Y.-W. Wang, J. Org. Chem., 2012, 77, 9072–9080 CrossRef CAS PubMed;
(b) D. Udhayakumari, S. Velmathi, W.-C. Chen and S.-P. Wu, Sens. Actuators, B, 2014, 204, 375–381 CrossRef CAS;
(c) M. Gupta, A. Balamurugan and H. Lee, Sens. Actuators, B, 2015, 211, 531–536 CrossRef CAS;
(d) H. Yang, H. Song, Y. Zhu and S. Yang, Tetrahedron Lett., 2012, 53, 2026–2029 CrossRef CAS.
- E. D. Eanes, I. Zipkin, R. A. Harper and A. S. Posner, Arch. Oral Biol., 1965, 10, 161–173 CrossRef CAS PubMed.
- J. A. Cury, L. M. A. Tenuta, C. C. C. Ribeiro and A. F. P. Leme, Braz. Dent. J., 2004, 15, 167–174 Search PubMed.
- J. Ekstrand, C. J. Spak and G. Vogel, J. Dent. Res., 1990, 69, 556–557 CrossRef.
- E. D. Eanes and A. H. Reddi, Metab. Bone Dis. Relat. Res., 1979, 2, 3–10 CrossRef CAS.
- V. Baelum, S. Pongpaisal, W. Pithpornchaiyakul, S. Pisuithanakan, R. Teanpaisan, P. N. Papapanou, G. Dahlen and O. Fejerskov, Acta Odontol. Scand., 2002, 60, 80–86 CrossRef PubMed.
- K. H. W. Lau and D. J. Baylink, J. Bone Miner. Res., 1998, 13, 1660–1667 CrossRef CAS PubMed.
- V. Desai and S. G. Kaler, Am. J. Clin. Nutr., 2008, 88, 855S–858S CAS.
- M. M. O. Pena, J. Lee and D. J. Thiele, J. Nutr., 1999, 129, 1251–1260 CAS.
-
(a) C. Liu, J. Xu, F. Yang, W. Zhou, Z. Li, L. Wei and M. Yu, Sens. Actuators, B, 2015, 212, 364–370 CrossRef CAS;
(b) S. Devaraj, D. Saravanakumar and M. Kandaswamy, Sens. Actuators, B, 2009, 136, 13 CrossRef CAS;
(c) Y. W. Choi, J. J. Lee, G. R. You, S. Y. Lee and C. Kim, RSC Adv., 2015, 5, 86463–86472 RSC.
-
(a) O. Sahin and E. Akceylan, Tetrahedron, 2014, 70, 6944–6950 CrossRef CAS;
(b) V. Luxami, A. S. Gupta and K. Paul, New J. Chem., 2014, 38, 2841–2846 RSC;
(c) S. Goswami, S. Chakraborty, S. Paul, S. Halder, S. Panja and S. K. Mukhopadhyay, Org. Biomol. Chem., 2014, 12, 3037–3044 RSC.
- P. Patrycja, S. Janusz, J. Anna, T. Bartosz, N. Wojciech and H. Maciej, Polyhedron, 2015, 87, 226–232 CrossRef.
- D. Prasad, A. Aamir, D. Jyoti, S. K. Venkateswara, K. M. Ejazuddin, K. Madhukar, P. Subhash and S. Fazlul, Bioorg. Med. Chem. Lett., 2014, 24, 2900–2904 CrossRef PubMed.
- Z. Lin, Y. Zhao, C. Duan, B. Zhang and Z. Bai, Dalton Trans., 2006, 3678–3684 RSC.
-
(a) X. Bao, J. Yu and Y. Zhou, Sens. Actuators, B, 2009, 140, 467–472 CrossRef CAS;
(b) X. Bao and Y. Zhou, Sens. Actuators, B, 2010, 147, 434–441 CrossRef CAS;
(c) J. Hao, K. Hiratani, N. Kameta and T. Oba, Supramol. Chem., 2011, 23, 319–328 CrossRef CAS.
-
(a) D. A. Jose, D. K. Kumar, B. Ganguly and A. Das, Org. Lett., 2004, 6, 3445–4344 CrossRef CAS PubMed;
(b) S. Ghosh, M. A. Alam, A. Ganguly, S. Dalapati and N. Guchhait, Inorg. Chim. Acta, 2015, 429, 39–45 CrossRef CAS.
- M. Kigga and D. R. Trivedi, J. Fluorine Chem., 2014, 160, 1–7 CrossRef CAS.
- T. G. Jo, Y. J. Na, J. J. Lee, M. M. Lee, S. Y. Lee and C. Kim, New J. Chem., 2015, 39, 2580–2587 RSC.
- H. Miyaji, W. Sato and J. L. Sessler, Angew. Chem., Int. Ed., 2001, 40, 154–157 CrossRef CAS.
- H. H. Hammud, A. Ghannoum and M. S. Masoud, Spectrochim. Acta, Part A, 2006, 63, 255–265 CrossRef PubMed.
- M. Suresh, A. K. Mandal, S. Saha, E. Suresh, A. Mandoli, R. Di Liddo, P. P. Parnigotto and A. Das, Org. Lett., 2010, 12, 5406–5409 CrossRef CAS PubMed.
- K. Hiratami, M. Nomoto, H. Sugihara and T. Okada, Chem. Lett., 1990, 19, 43–50 CrossRef.
- J. Khan, W. Rehman, B. Muhammad, M. Danish, Q. Mahmood and N. Bukhari, World Appl. Sci. J., 2009, 6, 1563–1568 CAS.
- J. R. Lakowicz, Principles of fluorescence spectroscopy, Plennum Press, New York, 1999 Search PubMed.
- B. K. Paul, A. Samanta and N. Guchhait, J. Phys. Chem. B, 2010, 114, 6183–6196 CrossRef CAS PubMed.
- A. Kumar, V. Kumar, U. Diwan and K. K. Upadhyay, Sens. Actuators, B, 2013, 176, 420–427 CrossRef CAS.
- S. Dalapati, M. A. Alam, S. Jana and N. Guchhait, J. Fluorine Chem., 2011, 132, 536–540 CrossRef CAS.
- IUPAC, Spectrochim. Acta, 1978, 33, 241 CrossRef.
Footnote |
† Electronic supplementary information (ESI) available. See DOI: 10.1039/c6ra09877k |
|
This journal is © The Royal Society of Chemistry 2016 |
Click here to see how this site uses Cookies. View our privacy policy here.