DOI:
10.1039/C6RA14404G
(Paper)
RSC Adv., 2016,
6, 75144-75151
[2.2]Paracyclophane-based carbene–copper catalyst tuned by transannular electronic effects for asymmetric boration†
Received
3rd June 2016
, Accepted 3rd August 2016
First published on 3rd August 2016
Abstract
A series of planar chiral carbene–copper complexes based on the [2.2]paracyclophane backbone with a pseudo-ortho substitution pattern have been synthesized and applied to asymmetric β-boration of α,β-unsaturated esters. As a result, transannular electronic effects of the substituent of the chiral catalyst have significant influence on the catalytic performance. A variety of chiral β-hydroxyl esters were obtained in excellent enantioselectivities (up to 97% ee) and yields (up to 99%).
Introduction
Organoboranes are versatile synthetic intermediates for the preparation of a wide range of organic molecules.1 Catalytic boration of C
C bonds with diboron reagents is a promising strategy for synthesis of organoboron derivatives.2 After the seminal report of Miyaura and Suzuki,3 several catalysts for the addition of diboron reagents to various unsaturated compounds including asymmetric variants were developed.4 In particular, copper-catalyzed β-boration of α,β-unsaturated carbonyl compounds with diborons has become the most straightforward approach to access structurally diverse carbonyl compounds containing a C–B bond. The copper-catalyzed asymmetric β-boration of α,β-unsaturated carbonyl compounds was first reported by Yun et al.5 Subsequently, carbene–copper catalysts for the enantioselective boration of α,β-unsaturated esters were devised by Fernaández and co-workers.6 Since then, catalysis mediated by N-heterocyclic carbenes (NHCs) as well as their metal complexes has been developed as one of the most efficient methods for synthesis of organoboranes.7 However, in pioneering studies on the use of NHCs as chiral ligands, substantial efforts was directed toward the control of their steric properties. To date, the consequences of an electronic tuning of the chiral carbene center on the catalytic performance of relevant complexes has not been examined. The development of chiral carbene–metal complexes, the catalytic performance of which can be remotely controlled by an electronic effect, represents a challenging conceptual advance in modern asymmetric transition metal catalysis.
Cyclophanes are a special group of aromatic compounds which consist of two phenyl rings locked face-to-face by aliphatic chains.8 The [2.2]paracyclophane core holds the two rings together with a well-defined orientation and facilitates strong through-space electronic interactions between the upper and lower rings in addition to the through-bond interactions normally associated with π-conjugated skeletons. Besides the unique electronic properties, [2.2]paracyclophane backbone as a planar chiral source has inspired great interest from researchers in asymmetric catalysis.9 Recently, our group also reported a series of planar chiral NHC precursors derived from [2.2]paracyclophane and employed them in asymmetric transition metal catalysis.10 On the basis of current knowledge, we anticipated that simple introduction of a range of substituents in the pseudo-ortho position of the [2.2]paracyclophane backbone exhibiting different transannular electronic effects might allow a rapid and effective fine-tuning of the electronic properties of the carbene center without change in the ligand's steric properties. Beyond, we also intended to examine the effects of such a modulation on the catalytic behavior of relevant complex. Herein, we report that the catalytic performance of [2.2]paracyclophane-based NHC–copper complexes in the asymmetric boration can be controlled by the electronic tuning of carbene ligands.
Results and discussion
Planar chiral imidazolium salts based on the pseudo-ortho-substituted [2.2]paracyclophane backbone were selected as the precursors of a series of target ligands showing almost the same steric hindrance (Fig. 1). We conceived that remote electronic effects should provide a handle to control the reactivity of metal–NHC–based catalysts.11 Therefore, the energies of the orbital occupied by the carbene lone pair of electrons (Eσ) and of the lowest unoccupied orbital at the carbene center (Eπ) were determined by density functional theory (DFT) calculations at the B3LYP/6-31G (d,p) level (Table 1). And a set of planar chiral NHC–copper complexes was prepared and applied to the asymmetric β-boration of α,β-unsaturated esters (Table 1). At 5 mol% catalyst loading in THF solvent at room temperature, all of these catalysts facilitated addition of bis(pinacolato)diboron (B2Pin2) to ethyl cinnamate, and after oxidative workup, the derived hydroxyl compound was isolated with significant levels of enantioenrichment (Table 1, entries 1–4). In line with our expectations, these NHC–copper complexes showed strikingly different behavior in the asymmetric boration. Thus, methoxyl-substituted (RP)-L1-CuCl, which contains the strongest electron donor of all ligands in Table 1 (Eσ = −5.33), gave the boration product 2c with 65% yield and 22% ee. By contrast, fluorine-substituted (SP)-L4-CuCl, which appears as the poorest electron donor of the whole series (Eσ = −5.64), furnished 87% yield and 80% ee. Furthermore, a comparative kinetic study of these ligands shows that a slight difference in the substitution pattern of the pseudo-ortho-substituted [2.2]paracyclophanyl NHC dramatically alters the activity of the resulting copper catalyst (Fig. 2). Based on these observations, we can conclude that, at least in the present case, the transannular electronic effects of the substituent on the pseudo-ortho-substituted [2.2]paracyclophanyl NHC has significant influence on the catalytic performances. Moreover, NHC–copper complexes with more electron deficient ligands give higher yields and selectivities.
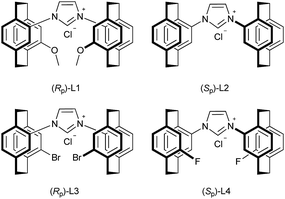 |
| Fig. 1 Various planar chiral carbene precursor. | |
Table 1 Investigation of transannular electronic effectsa
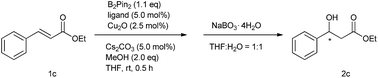
|
Entry |
Ligand |
Yieldb (%) |
eec (%) |
Eσd (eV) |
Eπ (eV) |
The reaction was carried out with ligand (5.0 mol%), Cu2O (2.5 mol%), Cs2CO3 (5.0 mol%), (B2Pin2) (0.19 mmol), 1c (0.17 mmol) and MeOH (0.34 mmol) in THF (0.5 mL) at room temperature. Yield of product after oxidated by NaBO3·4H2O. Determined by HPLC analysis using a chiral stationary phase (Chiralpak IA column). All calculations were performed by using the DFT method with B3LYP function implemented in Gaussian03 program package. Geometry optimizations were performed using the 6–31 G (d,p) basis set. |
1 |
(RP)-L1 |
65 |
22 (R) |
−5.33 |
−0.64 |
2 |
(SP)-L2 |
82 |
72 (S) |
−5.62 |
−0.54 |
3 |
(RP)-L3 |
89 |
64 (R) |
−5.57 |
−0.68 |
4 |
(SP)-L4 |
87 |
80 (S) |
−5.64 |
−0.54 |
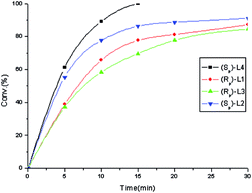 |
| Fig. 2 Comparison of the kinetics of the ligands in the Cu-catalyzed asymmetric boration of ethyl cinnamate. | |
With imidazolium salt (SP)-L4 as the optimal ligand, we screened the reaction conditions to optimize the yield and selectivity of the boration product. As shown in Table 2, strong bases such as KOtBu and NaOtBu did not improve the yields and enantioselectivities. Among the various nonprotic solvents tested (THF, dioxane, DME, Et2O, CH2Cl2, CHCl3, DCE, toluene), toluene was identified to afford the highest enantioselectivity (82% ee), so it was chosen as the optimal solvent (Table 2, entries 4–10). Interestingly, reaction could be performed at temperature between 0 °C and 40 °C with little erosion of enantioselectivity, although the reaction rate dropped sharply at 0 °C (Table 2, entries 11–12).
Table 2 Screening of the reaction conditionsa
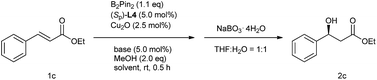
|
Entry |
Solvent |
T (°C) |
Base |
Yieldb (%) |
eec (%) |
The reaction was carried out with (Sp)-L4 (5.0 mol%), Cu2O (2.5 mol%), (B2Pin2) (0.19 mmol), 1c (0.17 mmol) and MeOH (0.34 mmol) in solvent (0.5 mL). Yield of product after oxidated by NaBO3·4H2O. Determined by HPLC analysis using a chiral stationary phase (Chiralpak IA column). The reaction was carried out in 5 h. The reaction was performed with the in situ formed catalyst system. |
1 |
THF |
25 |
Cs2CO3 |
87 |
80 |
2 |
THF |
25 |
NaOtBu |
74 |
77 |
3 |
THF |
25 |
KOtBu |
85 |
78 |
4 |
Dioxane |
25 |
Cs2CO3 |
84 |
78 |
5 |
DME |
25 |
Cs2CO3 |
80 |
70 |
6 |
Et2O |
25 |
Cs2CO3 |
83 |
71 |
7 |
CH2Cl2 |
25 |
Cs2CO3 |
87 |
81 |
8 |
CHCl3 |
25 |
Cs2CO3 |
73 |
68 |
9 |
DCE |
25 |
Cs2CO3 |
87 |
70 |
10 |
Toluene |
25 |
Cs2CO3 |
85 |
82 |
11d |
Toluene |
0 |
Cs2CO3 |
57 |
82 |
12 |
Toluene |
40 |
Cs2CO3 |
83 |
81 |
13e |
THF |
25 |
Cs2CO3 |
<5 |
— |
Under these optimized reaction conditions, we explored the scope of β-boration with various α,β-unsaturated esters containing diverse steric and electronic properties. The results were summarized in Table 3. First, the reaction scope was probed by changing the nature of the cinnamate partner. Methyl and benzylic cinnamates led to slightly lower selectivities (Table 3, entries 1–2). In contrast to our previously published results,10d increasing the size of the ester moiety of the substrate had a beneficial impact on the overall selectivity (Table 3, entries 3–6). Among the cinnamate partners screened, t-butyl cinnamate gave the best enantioselectivity (95% ee). Inspired by the exciting result, we continued to evaluate various β-substituted unsaturated t-butyl esters in order to investigate the substituent effect. It appears that substituents on the aromatic rings of the α,β-unsaturated esters have little effect on the catalytic activity and enantioselectivity (Table 3, entry 7–20), no matter whether it is a electron-withdrawing substituent or a electron-donating one. Besides, the substrate bearing a furyl ring proceeded smoothly to give the desired product 2u in good yield (96%) and enantiomeric excess (92% ee). Furthermore, the cyclohexyl-substituted α,β-unsaturated ester was a suitable substrate under the modified conditions, affording the boration product in good yield (87%) and stereoselectivity (93% ee). However, iso-propyl and tert-butyl substituted acrylates yielded the corresponding products with 84% and 86% enantiomeric excesses, respectively.
Table 3 Investigating the substrate scope of the reactiona
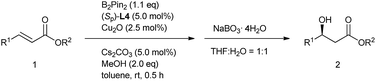
|
Entry |
R1 |
R2 |
Yieldb (%) |
eec (%) |
The reaction was carried out with (Sp)-L4 (5.0 mol%), Cu2O (2.5 mol%), Cs2CO3 (5.0 mol%), (B2Pin2) (0.165 mmol), substrate (0.15 mmol) and MeOH (0.30 mmol) in toluene (0.5 mL) at room temperature. Yield of product after oxidated by NaBO3·4H2O. Determined by HPLC analysis using a chiral stationary phase (Chiralpak IA or IB column). The absolute configuration was established by comparison of the optical rotation with literature values. The enantiomeric excess value was determined as benzoylated compound. |
1 |
Ph |
Me |
91 (2a) |
76 (S)d |
2 |
Ph |
Bn |
90 (2b) |
80 (S) |
3 |
Ph |
Et |
85 (2c) |
82 (S) |
4 |
Ph |
iPr |
87 (2d) |
87 (S) |
5 |
Ph |
iBu |
82 (2e) |
70 (S) |
6 |
Ph |
tBu |
86 (2f) |
95 (S) |
7 |
2-MeC6H4 |
tBu |
84 (2g) |
93 (S) |
8 |
3-MeC6H4 |
tBu |
84 (2h) |
90 (S) |
9 |
4-MeC6H4 |
tBu |
86 (2i) |
97 (S) |
10 |
2-ClC6H4 |
tBu |
99 (2j) |
91 (S) |
11 |
3-ClC6H4 |
tBu |
99 (2k) |
92 (S) |
12 |
4-ClC6H4 |
tBu |
97 (2l) |
93 (S) |
13 |
2-MeOC6H4 |
tBu |
86 (2m) |
90 (S) |
14 |
3-MeOC6H4 |
tBu |
83 (2n) |
94 (S) |
15 |
4-MeOC6H4 |
tBu |
85 (2o) |
95 (S) |
16 |
2-CF3C6H4 |
tBu |
91 (2p) |
90 (S) |
17 |
3-CF3C6H4 |
tBu |
83 (2q) |
95 (S) |
18 |
4-CF3C6H4 |
tBu |
88 (2r) |
95 (S) |
19 |
1-Naphthyl |
tBu |
99 (2s) |
91 (S) |
20 |
2-Naphthyl |
tBu |
94 (2t) |
95 (S) |
21 |
2-Furyl |
tBu |
96 (2u) |
92 (S) |
22e |
Cyclohexyl |
tBu |
87 (2v) |
93 (S) |
23e |
Isopropyl |
tBu |
81 (2w) |
84 (S) |
24e |
tBu |
tBu |
85 (2x) |
86 (S) |
To further demonstrate the applicability of our catalytic process, a larger scale reaction was attempted with only 0.5 mol% of (Sp)-L4 at room temperature. Under these conditions, the reaction could also complete in half an hour and gave the chiral β-hydroxyl esters 2f in 96% yield and 92% enantioselectivity (Scheme 1). This result indicated that the catalysis could also keep efficient with much less catalyst loadings (10% of the amount used before) when the substrates were broadened to a larger scale.
 |
| Scheme 1 Asymmetric β-boration of 1f on a larger scale. | |
Conclusions
In conclusion, we synthesized a series of planar chiral imidazolium salts derived from the pseudo-ortho-substituted [2.2]paracyclophane skeleton and evaluated their transannular electronic effects and chiral discrimination ability based on the Cu-catalyzed asymmetric β-boration of α,β-unsaturated esters. Our efforts led to the development of the fluorine-substituted catalyst (Sp)-L4-CuCl, which exhibited an exceptionally high reactivity and enantioselectivity in the asymmetric boration reaction by virtue of transannular electronic effects. To the best of our knowledge, this is the first successful example of an electronic tuning of the chiral carbene center on the catalytic performance of relevant complexes, which might provide a new direction to the design of the chiral carbene backbone.
Experimental
Imidazolium triflates of (RP)-L1,10g (SP)-L2 (ref. 10f) and (RP)-L3 (ref. 10f) were synthesized following a reported procedure. The corresponding imidazolium chlorides were obtained easily by anion exchange of their imidazolium triflates analogue with an ion-exchange resin (chloride form) according the same method as described by McQuade.12
Imidazolium chloride (RP)-L1
White solid: 126.4 mg, 65% yield, mp > 225 °C (dec); [α]25D = −101.0 (c 0.4, CHCl3); 1H NMR (300 MHz, CDCl3) δ 9.82 (s, 1H), 8.34 (s, 2H), 7.09–6.95 (m, 2H), 6.87–6.75 (m, 2H), 6.72–6.58 (m, 4H), 6.54–6.43 (m, 2H), 6.20–6.00 (m, 2H), 3.86 (s, 6H), 3.42 (ddd, J = 13.3, 9.2, 4.4 Hz, 2H), 3.36–3.20 (m, 4H), 3.20–3.02 (m, 4H), 3.02–2.89 (m, 4H), 2.82 (ddd, J = 13.4, 9.5, 5.5 Hz, 2H). 13C{1H}NMR (75 MHz, CDCl3) δ 158.3, 142.7, 142.2, 136.8, 135.9, 135.4, 134.7, 133.9, 132.3, 130.0, 128.2, 124.7, 123.4, 119.9, 60.2, 34.5, 33.7, 31.6, 30.3. HRMS (ESI-TOF) m/z [M − Cl]+ calcd for C37H37N2O2 541.2855, found 541.2858.
Imidazolium chloride (SP)-L2
White solid: 60.7 mg, 62% yield, mp > 250 °C (dec); [α]25D = +73.5 (c 0.4, CHCl3); 1H NMR (300 MHz, CDCl3) δ 10.90 (s, 1H), 8.04 (s, 2H), 7.09 (d, J = 6.5 Hz, 4H), 6.81–6.47 (m, 10H), 3.49–3.18 (m, 6H), 3.18–2.96 (m, 8H), 2.70 (dd, J = 15.6, 6.6 Hz, 2H). 13C{1H}NMR (75 MHz, CDCl3) δ 143.5, 140.4, 140.3, 138.3, 137.4, 135.9, 135.3, 133.7, 133.2, 133.1, 131.8, 128.5, 127.1, 123.6, 35.0, 34.9, 34.6, 32.9. HRMS (ESI-TOF) m/z [M − Cl]+ calcd for C35H33N2 481.2644, found 481.2643.
Imidazolium chloride (RP)-L3
White solid: 138.3 mg, 87% yield, mp > 250 °C (dec); [α]25D = −57.5 (c 0.3, CHCl3); 1H NMR (300 MHz, CDCl3) δ 11.21 (s, 1H), 8.38 (s, 2H), 7.24–7.17 (m, 2H), 6.93–6.84 (m, 2H), 6.77–6.66 (m, 6H), 6.65–6.57 (m, 2H), 3.64–3.44 (m, 4H), 3.31–3.13 (m, 6H), 3.07–2.88 (m, 4H), 2.71–2.55 (m, 2H). 13C{1H}NMR (75 MHz, CDCl3) δ 142.0, 141.5, 138.9, 138.0, 137.7, 135.9, 135.6, 133.4, 133.3, 132.7, 132.3, 126.1, 122.7, 122.5, 35.5, 33.9, 32.9, 32.3. HRMS (ESI-TOF) m/z [M − Cl]+ calcd for C35H31Br2N2 639.0834, found 639.0833.
General procedure for the synthesis of (Sp)-4-amino-12-fluoro[2.2]paracyclophane
(Sp)-4-Amino-12-fluoro[2.2]paracyclophane was synthesized following a reported procedure13 using the known enantiomer (Sp)-4-bromo-12-fluoro[2.2]paracyclophane14 as the starting material.
(Sp)-4-Amino-12-fluoro[2.2]paracyclophane ((Sp)-3)
White solid: 347.5 mg, 88% yield, mp 178–180 °C; [α]25D = −167.7 (c 0.13, CH2Cl2); 1H NMR (300 MHz, CDCl3) δ 6.49–6.34 (m, 2H), 6.34–6.28 (m, 1H), 6.27–6.19 (m, 1H), 6.17–6.02 (m, 1H), 5.82–5.70 (m, 1H), 3.73–3.52 (m, 1H), 3.40–3.19 (m, 1H), 3.06–2.94 (m, 3H), 2.94–2.86 (m, 1H), 2.86–2.72 (m, 2H), 2.72–0.44 (m, 2H). 13C{1H}NMR (75 MHz, CDCl3) δ 160.6 (d, 1JC–F = 237.8 Hz), 145.1, 141.6, 141.5, 140.1, 134.9, 134.8, 134.7, 128.8, 128.8, 124.5, 124.2, 123.5, 123.4, 121.4, 119.8, 119.5, 34.6, 34.5, 30.8, 26.1, 26.0. HRMS (ESI-TOF) m/z [M + H]+ calcd for C16H17FN 242.1345, found 242.1345.
General procedure for the synthesis of imidazolium chloride (SP)-L4
40% glyoxal (0.14 mL, 1.17 mmol) was added into the solution of (Sp)-4-amino-12-fluoro[2.2]paracyclophane (238.0 mg, 0.78 mmol) in THF (1.0 mL) and the mixture stirred at room temperature for 4 h before a yellow precipitate separated out. After completion of the reaction, as indicated by TLC, the yellow precipitate diimine was filtered, dried and prepared for the next step.
To a suspension of AgOTf (200.5 mg, 0.78 mmol) in THF (1.0 mL) was added chloromethyl pivalate (0.10 mL, 0.70 mmol) and the resulting suspension was sealed and stirred for 30 min in dark. Then the above diimine in CH2Cl2 (1.0 mL) was added and the mixture continued to stir in the sealed tube in dark at 40 °C for another 24 h. When the reaction was over, the suspension was filtered and solvent was removed under vacuum. The residue was purified by chromatographed on silica gel (CH2Cl2/ethanol = 30/1) to give the imidazolium triflate as a white solid. The corresponding imidazolium chloride (SP)-L4 was obtained easily by anion exchange of its imidazolium triflate analogue with an ion-exchange resin (chloride form) according the same method as described by McQuade.12
Imidazolium chloride (SP)-L4
White solid: 203.0 mg, 78% yield, mp > 250 °C; [α]25D = −88.7 (c 0.5, CHCl3); 1H NMR (300 MHz, CDCl3) δ 10.73 (s, 1H), 8.02 (s, 2H), 7.21–7.01 (m, 2H), 7.00–6.77 (m, 2H), 6.76–6.66 (m, 2H), 6.66–6.44 (m, 4H), 6.27–5.95 (m, 2H), 3.68–3.07 (m, 10H), 3.01 (ddd, J = 12.8, 9.3, 3.4 Hz, 2H), 2.85 (dt, J = 14.6, 5.5 Hz, 4H). 13C{1H}NMR (75 MHz, CDCl3) δ 160.6 (d, 1JC–F = 242.3 Hz), 143.7, 143.7, 143.7, 142.0, 137.6, 137.0, 137.0, 137.0, 136.3, 135.4, 135.4, 135.4, 134.1, 133.4, 129.4, 126.0, 125.8, 125.7, 123.5, 123.3, 117.4, 117.4, 117.1, 117.1, 34.4, 33.5, 31.6, 29.0. HRMS (ESI-TOF) m/z [M − Cl]+ calcd for C35H31F2N2 517.2450, found 517.2449.
General procedure for the copper-catalyzed β-boration of α,β-unsaturated esters
Imidazolium chloride (SP)-L4 (4.15 mg, 7.5 × 10−3 mmol), Cu2O (0.54 mg, 3.75 × 10−3 mmol) were added to 1.0 mL anhydrous CH2Cl2 in an oven dried Schlenk flask under an argon atmosphere. The mixture was stirred at 40 °C for 72 h before solvent was evaporated under argon at 60 °C and 0.5 mL anhydrous toluene was added at room temperature. Cs2CO3 (2.44 mg, 7.5 × 10−3 mmol) and bis(pinacolato)diboron (41.9 mg, 0.165 mmol) were added consecutively. The mixture was stirred at room temperature for 10 minutes before α,β-unsaturated esters (0.15 mmol) and MeOH (13.0 μL, 0.30 mmol) were added simultaneously. After being stirred for 0.5 h, the solvent was removed under vacuum and the crude product was subjected to the oxidation with sodium peroxoborate (115.4 mg, 5.0 eq.) in THF (1.0 mL) and H2O (1.0 mL) at room temperature for 3 h. The reaction mixture was concentrated in vacuum and the residue was purified by column chromatography on silica gel (n-hexane/ethyl acetate = 30/1–10/1), giving the corresponding alcohol 2.
(S)-Methyl 3-hydroxy-3-phenylpropanoate 2a
Colorless oil: 24.6 mg, 91% yield, 76% ee, [α]25D = −23.7 (c 0.17, CHCl3); [lit.15a [α]16D − 52.9 (c 1.00, CHCl3) for 95% ee (S)]. HPLC (Chiralpak IA column, n-hexane/iPrOH 75
:
1, 220 nm, 1.0 mL min−1), retention time: 45.4 min (minor), 50.5 min (major); 1H NMR (300 MHz, CDCl3) δ 7.50–7.27 (m, 5H), 5.15 (dd, J = 8.5, 4.3 Hz, 1H), 3.73 (s, 3H), 3.18 (s, 1H), 2.88–2.65 (m, 2H). 13C{1H}NMR (75 MHz, CDCl3) δ 172.9, 142.5, 128.7, 127.9, 125.7, 70.4, 52.0, 43.2, 29.8.
(S)-Benzyl 3-hydroxy-3-phenylpropanoate 2b
Colorless oil: 34.6 mg, 90% yield, 80% ee, [α]25D = −17.6 (c 0.15, CHCl3); [lit.15a [α]26D − 34.8 (c 1.12, CHCl3) for 91% ee (S)]. HPLC (Chiralpak IA column, n-hexane/EtOH 50
:
1, 220 nm, 1.0 mL min−1), retention time: 41.9 min (minor), 54.5 min (major); 1H NMR (300 MHz, CDCl3) δ 7.53–7.22 (m, 10H), 5.14 (s, 3H), 3.23 (d, J = 2.4 Hz, 1H), 2.92–2.67 (m, 2H). 13C{1H}NMR (75 MHz, CDCl3) δ 172.2, 142.5, 135.6, 128.7, 128.6, 128.6, 128.4, 128.4, 128.3, 128.2, 127.9, 127.8, 125.9, 125.7, 83.0, 72.9, 70.4, 66.7, 66.4, 44.0, 43.4, 24.6, 24.5.
(S)-Ethyl 3-hydroxy-3-phenylpropanoate 2c
Colorless oil: 24.8 mg, 85% yield, 82% ee, [α]25D = −35.4 (c 0.30, CHCl3); [lit.15a [α]26D − 46.5 (c 1.04, CHCl3) for 97% ee (S)]. HPLC (Chiralpak IA column, n-hexane/iPrOH 100
:
1, 220 nm, 1.0 mL min−1), retention time: 46.4 min (minor), 49.5 min (major); 1H NMR (300 MHz, CDCl3) δ 7.53–7.21 (m, 5H), 5.13 (dd, J = 8.4, 4.5 Hz, 1H), 4.18 (q, J = 7.1 Hz, 2H), 3.29 (s, 1H), 2.87–2.62 (m, 2H), 1.26 (t, J = 7.1 Hz, 3H). 13C{1H}NMR (75 MHz, CDCl3) δ 172.4, 142.5, 128.6, 127.8, 125.7, 70.3, 60.9, 43.3, 14.2.
(S)-Isopropyl 3-hydroxy-3-phenylpropanoate 2d
Colorless oil: 27.2 mg, 87% yield, 87% ee, [α]25D = −31.3 (c 0.20, CHCl3); [lit.15a [α]24D − 39.6 (c 0.94, CHCl3) for 92% ee (S)]. HPLC (Chiralpak IA column, n-hexane/EtOH 50
:
1, 220 nm, 1.0 mL min−1), retention time: 19.9 min (minor), 21.6 min (major); 1H NMR (300 MHz, CDCl3) δ 7.58–7.22 (m, 5H), 5.26–4.98 (m, 2H), 3.34 (s, 1H), 2.83–2.60 (m, 2H), 1.24 (dd, J = 6.3, 1.6 Hz, 6H). 13C{1H}NMR (75 MHz, CDCl3) δ 172.0, 142.5, 128.5, 127.8, 125.7, 70.3, 68.4, 43.6, 21.8.
(S)-iso-Butyl 3-hydroxy-3-phenylpropanoate 2e
Colorless oil: 27.3 mg, 82% yield, 70% ee, [α]25D = −33.6 (c 0.25, CHCl3); [lit.10d [α]25D − 40.5 (c 0.1, CHCl3) for 96% ee (S)]. HPLC (Chiralpak IA column, n-hexane/EtOH 50
:
1, 220 nm, 0.5 mL min−1), retention time: 38.0 min (minor), 41.8 min (major); 1H NMR (300 MHz, CDCl3) δ 7.48–7.08 (m, 5H), 5.04 (dd, J = 8.4, 4.5 Hz, 1H), 3.81 (d, J = 6.7 Hz, 2H), 3.29 (s, 1H), 2.80–2.52 (m, 2H), 1.83 (dp, J = 13.4, 6.7 Hz, 1H), 0.83 (d, J = 6.7 Hz, 6H). 13C{1H}NMR (75 MHz, CDCl3) δ 172.5, 142.5, 128.6, 127.8, 125.7, 71.0, 70.3, 43.3, 27.7, 19.0.
(S)-tert-Butyl 3-hydroxy-3-phenylpropanoate 2f
Colorless oil: 36.7 mg, 86% yield, 95% ee, [α]25D = −40.7 (c 0.30, CHCl3); [lit.15a [α]24D − 37.7 (c 1.20, CHCl3) for 88% ee (S)]. HPLC (Chiralpak IA column, n-hexane/iPrOH 75
:
1, 220 nm, 1.0 mL min−1), retention time: 22.9 min (minor), 25.0 min (major); 1H NMR (300 MHz, CDCl3) δ 7.54–7.11 (m, 5H), 5.04 (dd, J = 8.3, 4.6 Hz, 1H), 3.53 (s, 1H), 2.79–2.49 (m, 2H), 1.42 (s, 9H). 13C{1H}NMR (75 MHz, CDCl3) δ 172.2, 142.9, 128.8, 128.0, 126.0, 81.9, 70.7, 44.6, 28.4.
(S)-tert-Butyl 3-hydroxy-3-(2-methylphenyl)propanoate (2g)
Colorless oil 29.7 mg, 84% yield, 93% ee, [α]25D = −56.6 (c 0.2, CHCl3); HPLC (Chiralpak IA column, n-hexane/iPrOH 200
:
1, 220 nm, 1.0 mL min−1), retention time: 50.9 min (major), 60.8 min (minor); 1H NMR (300 MHz, CDCl3) δ 7.55–7.44 (m, 1H), 7.25–7.08 (m, 3H), 5.30 (dd, J = 8.1, 4.6 Hz, 1H), 3.33 (s, 1H), 2.77–2.49 (m, 2H), 2.35 (s, 3H), 1.47 (s, 9H). 13C{1H}NMR (75 MHz, CDCl3) δ 172.1, 140.5, 134.3, 130.4, 127.5, 126.3, 125.3, 81.5, 67.1, 43.0, 28.1, 19.0. HRMS (ESI-TOF) m/z [M + Na]+ calcd for C14H20NaO3 259.1310, found 259.1300.
(S)-tert-Butyl 3-hydroxy-3-(3-methylphenyl)propanoate (2h)
Colorless oil: 29.7 mg, 84% yield, 90% ee, [α]25D = −57.0 (c 0.15, CHCl3); HPLC (Chiralpak IA column, n-hexane/iPrOH 100
:
1, 220 nm, 1.0 mL min−1), retention time: 28.5 min (minor), 32.7 min (major); 1H NMR (300 MHz, CDCl3) δ 7.25–7.12 (m, 3H), 7.09 (d, J = 7.4 Hz, 1H), 5.05 (dd, J = 8.1, 4.6 Hz, 1H), 3.39 (s, 1H), 2.75–2.53 (m, 2H), 2.35 (s, 3H), 1.45 (s, 9H). 13C{1H}NMR (75 MHz, CDCl3) δ 172.0, 142.5, 138.1, 128.4, 128.4, 126.4, 122.8, 81.5, 70.4, 44.3, 28.1, 21.4. HRMS (ESI-TOF) m/z [M + Na]+ calcd for C14H20NaO3 259.1310, found 259.1308.
(S)-tert-Butyl 3-hydroxy-3-(4-methylphenyl)propanoate (2i)
Colorless oil: 30.3 mg, 86% yield, 97% ee, [α]25D = −33.8 (c 0.2, CHCl3); HPLC (Chiralpak IA column, n-hexane/iPrOH 100
:
1, 220 nm, 1.0 mL min−1), retention time: 42.0 min (minor), 45.2 min (major); 1H NMR (300 MHz, CDCl3) δ 7.27 (s, 1H), 7.24 (s, 1H), 7.15 (d, J = 7.9 Hz, 2H), 5.05 (dd, J = 8.4, 4.4 Hz, 1H), 3.34 (s, 1H), 2.82–2.51 (m, 2H), 2.34 (s, 3H), 1.45 (s, 9H). 13C{1H}NMR (75 MHz, CDCl3) δ 172.0, 139.7, 137.3, 129.1, 125.7, 81.45, 70.3, 44.3, 28.1, 21.1. HRMS (ESI-TOF) m/z [M + Na]+ calcd for C14H20NaO3 259.1310, found 259.1310.
(S)-tert-Butyl 3-hydroxy-3-(2-chlorophenyl)propanoate (2j)
Colorless oil: 38.1 mg, 99% yield, 91% ee, [α]25D = −45.0 (c 0.2, CHCl3); HPLC (Chiralpak IA column, n-hexane/iPrOH 75
:
1, 220 nm, 1.0 mL min−1), retention time: 14.8 min (major), 15.9 min (minor); 1H NMR (300 MHz, CDCl3) δ 7.68–7.55 (m, 1H), 7.38–7.27 (m, 2H), 7.25–7.15 (m, 1H), 5.44 (dd, J = 9.5, 2.7 Hz, 1H), 3.72 (s, 1H), 2.78 (dd, J = 16.6, 2.8 Hz, 1H), 2.51 (dd, J = 16.6, 9.5 Hz, 1H), 1.47 (s, 9H). 13C{1H}NMR (75 MHz, CDCl3) δ 172.0, 140.0, 131.4, 129.3, 128.6, 127.1, 81.7, 67.2, 42.3, 28.1. HRMS (ESI-TOF) m/z [M + Na]+ calcd for C13H17ClNaO3 279.0764, found 279.0732.
(S)-tert-Butyl 3-hydroxy-3-(3-chlorophenyl)propanoate (2k)
Colorless oil: 38.2 mg, 99% yield, 92% ee, [α]25D = −16.0 (c 0.2, CHCl3); HPLC (Chiralpak IA column, n-hexane/iPrOH 100
:
1, 220 nm, 0.5 mL min−1), retention time: 26.5 min (minor), 41.3 min (major); 1H NMR (300 MHz, CDCl3) δ 7.39 (d, J = 2.0 Hz, 1H), 7.27–7.18 (m, 3H), 5.05 (t, J = 6.3 Hz, 1H), 3.57 (s, 1H), 2.63 (d, J = 6.2 Hz, 2H), 1.45 (s, 9H). 13C{1H}NMR (75 MHz, CDCl3) δ 171.7, 144.6, 134.4, 129.7, 127.8, 126.0, 123.8, 81.8, 69.7, 44.0, 28.1. HRMS (ESI-TOF) m/z [M + Na]+ calcd for C13H17ClNaO3 279.0764, found 279.0758.
(S)-tert-Butyl 3-hydroxy-3-(4-chlorophenyl)propanoate (2l)
White solid: 37.3 mg, 97% yield, 93% ee, [α]25D = −48.8 (c 0.2, CHCl3); [lit.15b [α]20D + 13.8 (c 0.18, CHCl3) for 98% ee (R)]. Mp 48–50 °C; HPLC (Chiralpak IA column, n-hexane/iPrOH 30
:
1, 220 nm, 1.0 mL min−1), retention time: 15.4 min (major), 16.3 min (minor); 1H NMR (300 MHz, CDCl3) δ 7.54–7.28 (m, 4H), 5.19–4.96 (m, 1H), 3.57 (s, 1H), 2.76–2.49 (m, 2H), 1.45 (s, 9H). 13C{1H}NMR (75 MHz, CDCl3) δ 171.8, 141.1, 133.3, 128.6, 127.1, 81.8, 69.7, 44.1, 28.1. HRMS (ESI-TOF) m/z [M + Na]+ calcd for C13H17ClNaO3 279.0764, found 279.0763.
(S)-tert-Butyl 3-hydroxy-3-(2-methoxyphenyl)propanoate (2m)
White solid: 32.3 mg, 86% yield, 90% ee, [α]25D = −30.3 (c 0.15, CHCl3); mp 57–58 °C; HPLC (Chiralpak IA column, n-hexane/iPrOH 100
:
1, 220 nm, 1.0 mL min−1), retention time: 31.9 min (major), 33.6 min (minor); 1H NMR (300 MHz, CDCl3) δ 7.55–7.34 (m, 1H), 7.31–7.18 (m, 1H), 7.10–6.91 (m, 1H), 6.90–6.77 (m, 1H), 5.31 (dd, J = 9.0, 3.7 Hz, 1H), 3.85 (s, 3H), 3.55 (s, 1H), 2.82–2.52 (m, 2H), 1.45 (s, 9H). 13C{1H}NMR (75 MHz, CDCl3) δ 172.1, 156.1, 130.6, 128.5, 126.7, 120.7, 110.3, 81.1, 66.6, 55.3, 42.7, 28.1. HRMS (ESI-TOF) m/z [M + Na]+ calcd for C14H20NaO4 275.1259, found 275.1257.
(S)-tert-Butyl 3-hydroxy-3-(3-methoxyphenyl)propanoate (2n)
Colorless oil: 31.1 mg, 83% yield, 94% ee, [α]25D = −53.2 (c 0.15, CHCl3); HPLC (Chiralpak IB column, n-hexane/iPrOH 75
:
1, 220 nm, 1.0 mL min−1), retention time: 18.3 min (major), 20.5 min (minor); 1H NMR (300 MHz, CDCl3) δ 7.32–7.16 (m, 1H), 6.94 (d, J = 7.6 Hz, 2H), 6.86–6.72 (m, 1H), 5.06 (dd, J = 7.4, 5.3 Hz, 1H), 3.81 (s, 3H), 3.43 (s, 1H), 2.79–2.49 (m, 2H), 1.47 (d, J = 7.1 Hz, 9H). 13C{1H}NMR (75 MHz, CDCl3) δ 171.9, 159.8, 144.3, 129.5, 118.0, 113.3, 111.1, 81.6, 70.3, 55.2, 44.2, 28.1. HRMS (ESI-TOF) m/z [M + Na]+ calcd for C14H20NaO4 275.1259, found 275.1259.
(S)-tert-Butyl 3-hydroxy-3-(4-methoxyphenyl)propanoate (2o)
Colorless oil: 32.1 mg, 85% yield, 95% ee, [α]25D = −50.9 (c 0.18, CHCl3); [lit.15b [α]20D + 28.0 (c 0.15, CHCl3) for 97% ee (R)]. HPLC (Chiralpak IA column, n-hexane/EtOH 100
:
1, 220 nm, 1.0 mL min−1), retention time: 40.3 min (major), 44.5 min (minor); 1H NMR (300 MHz, CDCl3) δ 7.34–7.24 (m, 2H), 7.00–6.80 (m, 2H), 5.03 (dd, J = 8.7, 4.1 Hz, 1H), 3.80 (s, 3H), 3.35 (s, 1H), 2.64 (dd, J = 8.6, 6.4 Hz, 2H), 1.45 (s, 9H). 13C{1H}NMR (75 MHz, CDCl3) δ 171.9, 159.1, 134.8, 127.0, 113.8, 81.4, 70.0, 55.3, 44.3, 28.1. HRMS (ESI-TOF) m/z [M + Na]+ calcd for C14H20NaO4 275.1259, found 275.1257.
(S)-tert-Butyl 3-hydroxy-3-(2-trifluoromethylphenyl)propanoate (2p)
Colorless oil: 39.6 mg, 91% yield, 90% ee, [α]25D = −57.6 (c 0.2, CHCl3); HPLC (Chiralpak IA column, n-hexane/iPrOH 75
:
1, 254 nm, 1.0 mL min−1), retention time: 13.4 min (major), 16.0 min (minor); 1H NMR (300 MHz, CDCl3) δ 7.93–7.76 (m, 1H), 7.75–7.50 (m, 2H), 7.48–7.32 (m, 1H), 5.49 (dd, J = 8.9, 2.1 Hz, 1H), 3.68 (s, 1H), 2.80–2.43 (m, 2H), 1.48 (s, 9H). 13C{1H}NMR (75 MHz, CDCl3) δ 171.8, 141.5, 132.3, 127.7, 125.6, 125.5, 125.4, 124.3 (q, 1JC–F = 272.3 Hz), 81.8, 66.0, 44.3, 28.1. HRMS (ESI-TOF) m/z [M + Na]+ calcd for C14H17F3NaO3 313.1027, found 313.1022.
(S)-tert-Butyl 3-hydroxy-3-(3-trifluoromethylphenyl)propanoate (2q)
Colorless oil: 36.1 mg, 83% yield, 95% ee, [α]25D = −47.4 (c 0.15, CHCl3); HPLC (Chiralpak IA column, n-hexane/EtOH 100
:
1, 220 nm, 1.0 mL min−1), retention time: 13.0 min (minor), 13.9 min (major); 1H NMR (300 MHz, CDCl3) δ 7.66 (s, 1H), 7.60–7.51 (m, 2H), 7.51–7.43 (m, 1H), 5.14 (t, J = 6.3 Hz, 1H), 3.70 (s, 1H), 2.76–2.57 (m, 2H), 1.45 (s, 9H). 13C{1H}NMR (75 MHz, CDCl3) δ 171.7, 143.5, 129.1, 128.9, 124.5, 124.4, 124.1 (q, 1JC–F = 270.8 Hz), 122.6, 122.5, 82.0, 69.8, 44.0, 28.0. HRMS (ESI-TOF) m/z [M + Na]+ calcd for C14H17F3NaO3 313.1027, found 313.1059.
(S)-tert-Butyl 3-hydroxy-3-(4-trifluoromethylphenyl)propanoate (2r)
White solid: 38.3 mg, 88% yield, 95% ee, [α]25D = −50.0 (c 0.15, CHCl3); mp 50–51 °C; HPLC (Chiralpak IA column, n-hexane/iPrOH 100
:
1, 220 nm, 1.0 mL min−1), retention time: 28.3 min (major), 30.2 min (minor); 1H NMR (300 MHz, CDCl3) δ 7.61 (d, J = 8.2 Hz, 2H), 7.50 (d, J = 8.1 Hz, 2H), 5.21–5.07 (m, 1H), 3.66 (s, 1H), 2.91–2.45 (m, 2H), 1.46 (s, 9H). 13C{1H}NMR (75 MHz, CDCl3) δ 171.7, 146.5, 130.0, 126.0, 125.5, 125.4, 125.4, 125.3, 124.1 (q, 1JC–F = 270.0 Hz), 82.0, 69.7, 44.0, 28.0. HRMS (ESI-TOF) m/z [M + Na]+ calcd for C14H17F3NaO3 313.1027, found 313.1014.
(S)-tert-Butyl 3-hydroxy-3-(naphthalen-1-yl)propanoate (2s)
Colorless oil: 40.4 mg, 99% yield, 91% ee, [α]25D = −46.7 (c 0.2, CHCl3); [lit.15c [α]24D + 28.2 (c 2.20, EtOH) for 94% ee (R)]. HPLC (Chiralpak IA column, n-hexane/iPrOH 50
:
1, 254 nm, 1.0 mL min−1), retention time: 22.9 min (major), 26.2 min (minor); 1H NMR (300 MHz, CDCl3) δ 8.17–8.01 (m, 1H), 7.93–7.84 (m, 1H), 7.83–7.74 (m, 1H), 7.74–7.65 (m, 1H), 7.59–7.42 (m, 3H), 5.87 (dd, J = 9.2, 3.3 Hz, 1H), 3.51 (s, 1H), 2.80 (qd, J = 16.6, 6.3 Hz, 2H), 1.49 (s, 9H). 13C{1H}NMR (75 MHz, CDCl3) δ 172.2, 138.1, 133.8, 130.1, 129.0, 128.2, 126.2, 125.5, 125.5, 123.0, 122.9, 81.6, 67.4, 43.6, 28.1. HRMS (ESI-TOF) m/z [M + Na]+ calcd for C17H20NaO3 295.1310, found 295.1305.
(S)-tert-Butyl 3-hydroxy-3-(naphthalen-2-yl)propanoate (2t)
White solid: 38.4 mg, 94% yield, 95% ee, [α]25D = −53.6 (c 0.15, CHCl3); [lit.15d [α]20D − 36.9 (c 0.42, CHCl3) for 96% ee (S)]. Mp 65–66 °C; HPLC (Chiralpak IA column, n-hexane/iPrOH 30
:
1, 220 nm, 1.0 mL min−1), retention time: 21.2 min (major), 22.3 min (minor); 1H NMR (300 MHz, CDCl3) δ 8.02–7.67 (m, 4H), 7.63–7.33 (m, 3H), 5.36–5.15 (m, 1H), 3.54 (s, 1H), 2.85–2.58 (m, 2H), 1.46 (s, 9H). 13C{1H}NMR (75 MHz, CDCl3) δ 171.9, 140.0, 133.3, 133.0, 128.3, 128.0, 127.7, 126.2, 125.9, 124.5, 123.9, 81.6, 70.5, 44.2, 28.1. HRMS (ESI-TOF) m/z [M + Na]+ calcd for C17H20NaO3 295.1310, found 295.1299.
(S)-tert-Butyl 3-hydroxy-3-(furan-2-yl)propanoate (2u)
Colorless oil: 30.7 mg, 96% yield, 92% ee, [α]25D = −22.5 (c 0.2, CHCl3); [lit.15e [α]25D + 22.2 (c 1.5, CHCl3) for 90% ee (R)]. HPLC (Chiralpak IA column, n-hexane/iPrOH 25
:
1, 220 nm, 0.5 mL min−1), retention time: 28.5 min (major), 31.1 min (minor); 1H NMR (300 MHz, CDCl3) δ 7.37 (dd, J = 1.8, 0.8 Hz, 1H), 6.33 (dd, J = 3.2, 1.8 Hz, 1H), 6.27 (d, J = 3.2 Hz, 1H), 5.08 (dd, J = 7.7, 4.5 Hz, 1H), 3.40 (s, 1H), 2.92–2.68 (m, 2H), 1.46 (s, 9H). 13C{1H}NMR (75 MHz, CDCl3) δ 171.4, 154.9, 142.1, 110.2, 106.2, 81.7, 64.3, 40.7, 28.1. HRMS (ESI-TOF) m/z [M + Na]+ calcd for C11H16NaO4 235.0946, found 235.0934.
(S)-tert-Butyl 3-cyclohexyl-3-hydroxypropanoate (2v)
Colorless oil: 29.7 mg, 87% yield, 93% ee, [α]25D = −43.2 (c 0.2, CHCl3); [lit.15e [α]25D + 26.8 (c 1.1, CHCl3) for 92% ee (R)]. HPLC (benzoylated, Chiralpak IA column, n-hexane/iPrOH 75
:
1, 220 nm, 1.0 mL min−1), retention time: 8.0 min (minor), 31.0 min (major); 1H NMR (300 MHz, CDCl3) δ 3.73 (ddd, J = 9.1, 6.0, 3.0 Hz, 1H), 3.00 (s, 1H), 2.57–2.23 (m, 2H), 1.96–1.81 (m, 1H), 1.81–1.71 (m, 2H), 1.71–1.60 (m, 2H), 1.47 (s, 9H), 1.42–1.28 (m, 2H), 1.23–1.13 (m, 2H), 1.13–0.96 (m, 2H). 13C{1H}NMR (75 MHz, CDCl3) δ 173.1, 81.2, 72.2, 43.0, 39.5, 28.8, 28.3, 28.1, 26.5, 26.2, 26.1, 23.0. HRMS (ESI-TOF) m/z [M + Na]+ calcd for C13H24NaO3 251.1623, found 251.1615.
The synthesis of (S)-3-(tert-butoxy)-1-cyclohexyl-3-oxopropyl benzoate (2v′)
To the oxidized product (0.19 mmol) in dichloromethane (1.0 mL) were added pyridine (75.2 mg, 0.95 mmol) and benzoyl chloride (53.6 mg, 0.38 mmol). After the reaction mixture was stirred for 1 h at 0 °C, the resulting mixture was quenched with 1.0 mL of water. The obtained organic layer was dried over anhydrous Na2SO4. After removal of solvent, the residue was purified by preparative TLC (n-hexane/ethyl acetate = 25/1) to afford benzoylated product as a colorless oil: 47.0 mg, 74% yield; [α]25D = −54.6 (c 0.13, CHCl3); 1H NMR (300 MHz, CDCl3) δ 8.17–7.96 (m, 2H), 7.60–7.50 (m, 1H), 7.49–7.36 (m, 2H), 5.45–5.31 (m, 1H), 2.75–2.52 (m, 2H), 1.97–1.53 (m, 7H), 1.35 (s, 9H), 1.23–1.06 (m, 4H). 13C{1H}NMR (75 MHz, CDCl3) δ 170.1, 165.8, 132.8, 130.5, 129.6, 128.3, 80.8, 75.0, 41.6, 38.3, 28.8, 28.2, 27.9, 26.3, 26.0, 26.0. HRMS (ESI-TOF) m/z [M + Na]+ calcd for C20H28NaO4 355.1885, found 355.1878.
(S)-tert-Butyl 3-hydroxy-4-methylpentanoate (2w)
Colorless oil: 22.9 mg, 81% yield, 84% ee, HPLC (benzoylated, Chiralpak IA column, n-hexane/iPrOH 75
:
1, 220 nm, 1.0 mL min−1), retention time: 7.1 min (minor), 17.2 min (major); [α]25D = −37.5 (c 0.2, CHCl3); [lit.15e [α]25D + 35.0 (c 1.1, CHCl3) for 95% ee (R)]. 1H NMR (300 MHz, CDCl3) δ 3.73 (s, 1H), 3.07 (s, 1H), 2.37 (qd, J = 16.2, 6.2 Hz, 2H), 1.82–1.61 (m, 1H), 1.47 (s, 9H), 0.93 (dd, J = 8.6, 6.9 Hz, 6H). 13C{1H}NMR (75 MHz, CDCl3) δ 173.0, 81.2, 72.8, 39.4, 33.1, 28.1, 18.3, 17.8. HRMS (ESI-TOF) m/z [M + Na]+ calcd for C10H20NaO3 211.1310, found 211.1303.
(S)-1-(tert-Butoxy)-4-methyl-1-oxopentan-3-yl benzoate (2w′)
Colorless oil: 52.6 mg, 84% yield; [α]25D = −24.5 (c 0.18, CHCl3); 1H NMR (300 MHz, CDCl3) δ 8.21–7.92 (m, 2H), 7.61–7.50 (m, 1H), 7.49–7.35 (m, 2H), 5.38 (dt, J = 8.2, 5.2 Hz, 1H), 2.74–2.51 (m, 2H), 2.15–1.94 (m, 1H), 1.35 (s, 9H), 1.00 (dd, J = 6.8, 3.4 Hz, 6H). 13C{1H}NMR (75 MHz, CDCl3) δ 167.0, 165.8, 132.8, 130.5, 129.6, 128.3, 80.8, 75.4, 38.1, 31.7, 27.9, 18.3, 17.7. HRMS (ESI-TOF) m/z [M + Na]+ calcd for C17H24NaO4 315.1572, found 315.1572.
(S)-tert-Butyl 3-hydroxy-4,4-dimethylpentanoate (2x)
Colorless oil: 34.3 mg, 85% yield, 86% ee, [α]25D = −33.3 (c 0.15, CHCl3); HPLC (benzoylated, Chiralpak IA column, n-hexane/iPrOH 50
:
1, 220 nm, 1.0 mL min−1), retention time: 6.0 min (minor), 9.7 min (major).
(S)-1-(tert-Butoxy)-4,4-dimethyl-1-oxopentan-3-yl benzoate (2x′)
Colorless oil: 32.2 mg, 88% yield; [α]25D = −20.8 (c 0.12, CHCl3); 1H NMR (300 MHz, CDCl3) δ 8.10–8.00 (m, 2H), 7.59–7.50 (m, 1H), 7.49–7.38 (m, 2H), 5.40 (dd, J = 9.3, 3.9 Hz, 1H), 2.58 (qd, J = 15.0, 6.6 Hz, 2H), 1.32 (s, 9H), 1.00 (s, 9H). 13C{1H}NMR (75 MHz, CDCl3) δ 170.3, 165.7, 132.7, 130.6, 129.6, 128.3, 80.9, 37.4, 34.9, 27.8, 25.9. HRMS (ESI-TOF) m/z [M + Na]+ calcd for C18H26NaO4 329.1729, found 329.1732.
Acknowledgements
Financial support from the National Natural Science Foundation of China (Grant No. 21372144 and 81473085) and Department of Science and Technology of Shandong Province is gratefully acknowledged.
Notes and references
-
(a) D. G. Hall, Boronic Acids: Preparation and Applications in Organic Synthesis, Medicine and Materials, Wiley-VCH GmbH & Co., Weinheim, 2nd edn, 2011 Search PubMed;
(b) H. K. Scott and V. K. Aggarwal, Chem.–Eur. J., 2011, 17, 13124 CrossRef CAS PubMed;
(c) B. W. Glasspoole and C. M. Crudden, Nat. Chem., 2011, 3, 912 CrossRef CAS PubMed;
(d) D. Leonori and V. K. Aggarwal, Angew. Chem., Int. Ed., 2015, 54, 1082 CrossRef CAS PubMed.
-
(a) G. J. Irvine, M. J. G. Lesley, T. B. Marder, N. C. Norman, C. R. Rice, E. G. Robins, W. R. Roper, G. R. Whittell and L. J. Wright, Chem. Rev., 1998, 98, 2685 CrossRef CAS PubMed;
(b) T. B. Marder and N. C. Norman, Top. Catal., 1998, 5, 63 CrossRef CAS;
(c) K. Takahashi, T. Ishiyama and N. Miyaura, Chem. Lett., 2000, 982 CrossRef CAS;
(d) I. Beletskaya and C. Moberg, Chem. Rev., 2006, 106, 2320 CrossRef CAS PubMed.
- T. Ishiyama, N. Matsuda, N. Miyaura and A. Suzuki, J. Am. Chem. Soc., 1993, 115, 11018 CrossRef CAS.
-
(a) C. N. Iverson and M. R. Smith III, Organometallics, 1997, 16, 2757 CrossRef CAS;
(b) T. B. Marder, N. C. Norman and C. R. Rice, Tetrahedron Lett., 1998, 39, 155 CrossRef CAS;
(c) C. Dai, E. G. Robins, A. J. Scott, W. Clegg, D. S. Yufit, J. A. K. Howard and T. B. Marder, Chem. Commun., 1998, 1983 RSC;
(d) P. Nguyen, R. B. Coapes, A. D. Woodward, N. J. Taylor, J. M. Burke, J. A. K. Howard and T. B. Marder, J. Organomet. Chem., 2002, 652, 77 CrossRef CAS;
(e) J. B. Morgan, S. P. Miller and J. P. Morken, J. Am. Chem. Soc., 2003, 125, 8702 CrossRef CAS PubMed;
(f) N. F. Pelz, A. R. Woodward, H. E. Burks, J. D. Sieber and J. P. Morken, J. Am. Chem. Soc., 2004, 126, 16328 CrossRef CAS PubMed;
(g) J. Ramírez, R. Corberán, M. Sanaú, E. Peris and E. Fernandez, Chem. Commun., 2005, 3056 RSC;
(h) P. Liu, Y. Fukui, P. Tian, Z. He, C. Sun, N. Wu and G. Lin, J. Am. Chem. Soc., 2013, 135, 11700 CrossRef CAS PubMed.
- J. E. Lee and J. Yun, Angew. Chem., Int. Ed., 2008, 47, 145 CrossRef CAS PubMed.
- V. Lillo, A. Prieto, A. Bonet, M. M. Díaz-Requejo, J. Ramírez, P. J. Pérez and E. Fernández, Organometallics, 2009, 28, 659 CrossRef CAS.
-
(a) J. M. O'Brien, K. S. Lee and A. H. Hoveyda, J. Am. Chem. Soc., 2010, 132, 10630 CrossRef PubMed;
(b) J. K. Park, H. H. Lackey, M. D. Rexford, K. Kovnir, M. Shatruk and D. T. McQuade, Org. Lett., 2010, 12, 5008 CrossRef CAS PubMed;
(c) D. H. Weil, K. A. Abboud and S. Hong, Chem. Commun., 2010, 46, 7525 RSC;
(d) H. Wu, S. Radomkit, J. M. O'Brien and A. H. Hoveyda, J. Am. Chem. Soc., 2012, 134, 8277 CrossRef CAS PubMed.
-
(a) H. M. Muchall, in Modern Cyclophane Chemistry, ed. R. Gleiter and H. Hopf, Wiley-VCH, Verlag GmbH & Co. KGaA, 2004, p. 259 Search PubMed;
(b) C. J. Brown and A. C. Farthing, Nature, 1949, 164, 915 CrossRef CAS;
(c) A. A. Aly and A. B. Brown, Tetrahedron, 2009, 65, 8055 CrossRef CAS.
-
(a) P. J. Pye, K. Rossen, R. A. Reamer, N. N. Tsou, R. P. Volante and P. J. Reider, J. Am. Chem. Soc., 1997, 119, 6207 CrossRef CAS;
(b) N. Hermanns, S. Dahmen, C. Bolm and S. Bräse, Angew. Chem., Int. Ed., 2002, 41, 3692 CrossRef CAS;
(c) S. Dahmen and S. Bräse, J. Am. Chem. Soc., 2002, 124, 5940 CrossRef CAS PubMed;
(d) S. E. Gibson and J. D. Knight, Org. Biomol. Chem., 2003, 1, 1256 RSC;
(e) C. Bolm, T. Focken and G. Raabe, Tetrahedron: Asymmetry, 2003, 14, 1733 CrossRef CAS;
(f) X. W. Wu, T. Z. Zhang and X. L. Hou, Tetrahedron: Asymmetry, 2004, 15, 2357 CrossRef CAS;
(g) S. Bräse and S. Höfener, Angew. Chem., Int. Ed., 2005, 44, 7879 CrossRef PubMed;
(h) D. K. Whelligan and C. Bolm, J. Org. Chem., 2006, 71, 4609 CrossRef CAS PubMed;
(i) A. Fürstner, M. Alcarazo, H. Krause and C. W. Lehmann, J. Am. Chem. Soc., 2007, 129, 12676 CrossRef PubMed;
(j) J. F. Schneider, F. C. Falk, R. Fröhlich and J. Paradies, Eur. J. Org. Chem., 2010, 75, 2265 CrossRef;
(k) J. Paradies, Synthesis, 2011, 23, 3749 CrossRef;
(l) S. Kitagaki, T. Ueda and C. Mukai, Chem. Commun., 2013, 49, 4030 RSC;
(m) B. Jiang, Y. Lei and X. L. Zhao, J. Org. Chem., 2008, 73, 7833 CrossRef CAS PubMed;
(n) V. Rozenberg, E. Sergeeva and H. Hopf, in Modern Cyclophane Chemistry, Wily-VCH, Weinheim, Germany, 2004, p. 435 Search PubMed.
-
(a) B. Hong, Y. Ma, L. Zhao, W. Duan, F. He and C. Song, Tetrahedron: Asymmetry, 2011, 22, 1055 CrossRef CAS;
(b) L. Zhao, Y. Ma, W. Duan, F. He, J. Chen and C. Song, Org. Lett., 2012, 14, 5780 CrossRef CAS PubMed;
(c) L. Zhao, Y. Ma, W. Duan, F. He, J. Chen and C. Song, J. Org. Chem., 2013, 78, 1677 CrossRef CAS PubMed;
(d) Z. Niu, J. Chen, Z. Chen, M. Ma, C. Song and Y. Ma, J. Org. Chem., 2015, 80, 602 CrossRef CAS PubMed;
(e) L. Wang, Z. Chen, M. Ma, W. Duan, C. Song and Y. Ma, Org. Biomol. Chem., 2015, 13, 10691 RSC;
(f) Q. Ma, Y. Ma, X. Liu, W. Duan, B. Qu and C. Song, Tetrahedron: Asymmetry, 2010, 21, 292 CrossRef CAS;
(g) W. Duan, Y. Ma, B. Qu, L. Zhao, J. Chen and C. Song, Tetrahedron: Asymmetry, 2012, 23, 1369 CrossRef CAS.
- M. Alcarazo, T. Stork, A. Anoop, W. Thiel and A. Fürstner, Angew. Chem., Int. Ed., 2010, 49, 2542 CrossRef CAS PubMed.
- S. M. Opalka, J. K. Park, A. R. Longstreet and D. T. McQuade, Org. Lett., 2013, 15, 996 CrossRef CAS PubMed.
- B. Qu, Y. Ma, Q. Ma, X. Liu, F. He and C. Song, J. Org. Chem., 2009, 74, 6867 CrossRef CAS PubMed.
- F. He, Y. Ma, L. Zhao, W. Duan, J. Chen and C. Song, Org. Lett., 2012, 14, 5436 CrossRef CAS PubMed.
-
(a) K. Toribatake, L. Zhou, A. Tsuruta and H. Nishiyama, Tetrahedron, 2013, 69, 3551 CrossRef CAS;
(b) E. T. Choi, M. H. Lee, Y. Kim and Y. S. Park, Tetrahedron, 2008, 64, 1515 CrossRef CAS;
(c) S. E. Denmark, G. L. Beutner, T. Wynn and M. D. Eastgate, J. Am. Chem. Soc., 2005, 127, 3777 Search PubMed;
(d) A. E. Díaz-Álvarez, L. Mesas-Sánchez and P. Dinér, Molecules, 2014, 19, 14273 CrossRef PubMed;
(e) V. R. O. Duthaler, P. Herold, W. Lottenbach, K. Oertle and M. Riediker, Angew. Chem., 1989, 101, 490 CrossRef.
Footnote |
† Electronic supplementary information (ESI) available: Experimental procedures, full compound characterization, detailed spectral data and HPLC chromatograms for products. See DOI: 10.1039/c6ra14404g |
|
This journal is © The Royal Society of Chemistry 2016 |