DOI:
10.1039/C6RA16031J
(Communication)
RSC Adv., 2016,
6, 73450-73453
Tandem cycloaddition–decarboxylation of α-keto acid and isocyanide under oxidant-free conditions towards monosubstituted oxazoles†
Received
21st June 2016
, Accepted 20th July 2016
First published on 26th July 2016
Abstract
An efficient method, tandem [3 + 2] cycloaddition–decarboxylation of α-keto acid and isocyanide promoted by copper salt, has been developed. Under oxidant-free conditions, a series monosubstituted oxazoles have been constructed. Different from the traditional application of α-oxo acids as acyl surrogates, the elegant approach herein ingeniously avoids consuming excess oxidants.
Oxazoles are very useful building blocks in organic chemistry because they are essential moieties in many synthetic materials, natural products and medicines.1 For example, VX-497 and texaline which contain oxazole rings, are used as conventional immunosuppressants1d and antimycobacterial drugs respectively. As a consequence, increasing attention has been paid to the synthesis of oxazoles and their derivatives.2 One of the most important strategy towards oxazoles is [3 + 2] cycloaddition reactions of methyl isocyanides with electrophilic acyl compounds.3,4 Differently, Lei's group recently developed a silver promoted novel [3 + 2] cycloaddition route of methyl isocyanides and α-oxocarboxylates, where α-oxocarboxylates acted as nucleophiles rather than an electrophilic acyl source.5 The α-keto acids and α-oxocarboxylates have received extensive attention in organic chemistry because they could be used as highly effective acyl surrogates for C–Z (Z = C, N, S) bond formation via decarboxylation reactions.6–16 In 2014, Lei described a synthetic method of 6-acyl phenanthridines through silver catalyzed oxidative decarboxylation–cyclization of α-oxocarboxylates and isocyanides in the presence of excess Na2S2O8.11 The same year, Duan et al. reported a decarboxylative acylfluorination of styrenes with α-oxocarboxylic acids using Selectfluor as oxidant.12 Mechanically, it was thought that initial decarboxylation of α-keto acids to form “acyl” is crucial among these examples. In addition, it should be noted that the decarboxylation catalyzed by transition metal is a fast oxidative process, and thus excessive oxidants such as persulfate and Selectfluor were necessary, which add the complication and difficulty in separation of products.
In recent years, we have focused on the research of organic reactions catalyzed by transition metal organic complexes17 and the synthesis of heterocycle compounds using methyl isocyanides as N-donor.18 On the ongoing interests for construction of heterocycle by using isocyanides, we questioned whether we could find an ideal system, in which α-oxocarboxylic acids or α-oxocarboxylates will be directly involved in reaction as acyl reagents before the release of CO2. At the same time, we hope that the decarboxylation will be completed automatically, so that the use of oxidants could be avoided. Herein, we disclose our results on tandem [3 + 2] cycloaddition–decarboxylation of isocyanide and α-keto acid in one pot for the synthesis of monosubstituted oxazoles without using any oxidant. This novel method provides a convenient access to 5-aryl oxazoles promoted by copper salt.
Seeking to find a novel efficient, cheap and concise method accords with the concept of green chemistry. Base on this point, inexpensive copper salts were chosen as catalysts for optimizing the reaction conditions. Originally, our investigation started with α-oxo-2-phenylacetic acid 1a and TosMIC 2 to give the corresponding 5-phenyloxazole 3a as the model reaction. Under air atmosphere, the product 3a can be monitored by GC in 60% yield in the presence of (1 mol%) Cu(NO3)2 and 1.1 equiv. of KOH at 80 °C in DMF for 12 h (Table 1, entry 1). Then, it was found that low-valent copper salt was more conductive to the tandem reaction (entries 2, 3). In comparison, the yields of 3a were basically the same with CuI or CuCl as catalyst. In addition, copper salt was helpful to the transformation (entry 4, 5). Taken together, CuCl was more ideal because of lower cost. With 1,10-phen as an additive, the yield increased dramatically (entry 6). If the temperature continued to rise, the yields decreased rapidly (entry 7, 8). In addition, both the usage of NaOH as base and the decreasing of temperature to 60 °C need a longer time to 12 hours for getting a good and medium yield, respectively (entries 9, 10). Increasing the amount of CuCl to 5 mol%, reaction time was shortened obviously (entry 11). Interestingly, the equivalent target product can be tested even in the nitrogen flow throughout (entry 12). That was to say, the process of cyclization–decarboxylation was no need for any oxidant. No significant difference was observed when α-oxocarboxylates as substrate in the absence of additional base (entry 13). Therefore, the optimal oxidant-free system under air for this reaction involves CuCl/1,10-phen (1 mol%) and KOH (1.1 eq.) in DMF at 80 °C.
Table 1 Optimization of reaction conditionsa
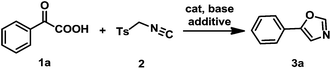
|
Entry |
Cat (1 mol%) |
Additive (1 mol%) |
Base (1.1 eq.) |
Temp. (°C) |
Time (h) |
Yieldb (%) |
Reaction conditions: 1a (0.55 mmol; 83.3 mg), 2 (0.5 mmol; 97.6 mg), catalyst (1 mol%), 1,10-phen (1 mol%, 0.9 mg), base (1.1 eq.), DMF (1 mL), air. Determined by GC. 5 mol% catalyst and 5 mol% ligand. N2 atmosphere. α-Oxocarboxylates as substrate. Yield determined by GC, the reactive mixture is difficult to be separated. |
1 |
Cu(NO3)2 |
— |
KOH |
80 |
12 |
60 |
2 |
CuI |
— |
KOH |
80 |
5 |
86 |
3 |
CuCl |
— |
KOH |
80 |
5 |
89 |
4 |
— |
— |
KOH |
80 |
5 |
42 |
5 |
— |
— |
K2CO3 |
80 |
12 |
26f |
6 |
CuCl |
1,10-Phen |
KOH |
80 |
5 |
96 |
7 |
CuCl |
1,10-Phen |
KOH |
120 |
12 |
30 |
8 |
CuCl |
1,10-Phen |
KOH |
160 |
12 |
15 |
9 |
CuCl |
1,10-Phen |
NaOH |
80 |
12 |
95 |
10 |
CuCl |
1,10-Phen |
KOH |
60 |
12 |
78 |
11c |
CuCl |
1,10-Phen |
KOH |
80 |
1 |
97 |
12d |
CuCl |
1,10-Phen |
KOH |
80 |
5 |
95 |
13e |
CuCl |
1,10-Phen |
— |
80 |
5 |
98 |
Subsequent work was to evaluate the scopes and limitations of cyclization–decarboxylation reaction of TosMIC 2 with a variety of substituted α-oxocarboxylic acids under the optimal condition (Table 1, entry 5). α-Oxo-2-phenylacetic acids with electron-donating groups in the meta and para position, such as both methyl and methoxy, performed well to the desired products in excellent yields (Table 2, entries 2–5). As well, the combination of CuCl and 1,10-phen was amenable to substrates bearing halogen groups (F, Cl, Br, I) and electron-deficient (–NO2), providing the satisfactory C-5 substituted oxazoles (3f–k) with good yields (entries 6–11). Furthermore, the space steric hindrance employing substituents at ortho position of α-oxo-2-phenylacetic acids showed a slight influence on the formation of corresponding oxazoles (3e, 3j). It is noteworthy that α-keto acid containing thienyl ring or naphthalene ring, due to its general reactivity, was monitored moderate yield of target product (3l, 3m) even lengthening the time to 12 hours and complicated mixture was detected by GC after a longer time (entries 12, 13). Whereas, aliphatic ketonic acid was inert to the conversion even extending the reaction to 24 h (Table 2, entry 14).
Table 2 Synthesis of monosubstituted oxazolesa

|
Entry |
R |
Time (h) |
Yieldb (%) |
Reaction conditions: 1 (0.55 mmol), 2 (0.5 mmol; 97.6 mg), CuCl (1 mol%; 1.3 mg), 1,10-phen (1 mol%, 0.9 mg), KOH (0.55 mmol; 25.3 mg), DMF (1 mL), 80 °C, air. Determined by GC; isolated yield in parenthese. |
1 |
1a |
C6H5– |
5 |
3a |
96 (91) |
2 |
1b |
4-CH3C6H4– |
8 |
3b |
98 (94) |
3 |
1c |
4-CH3OC6H4– |
10 |
3c |
98 (92) |
4 |
1d |
3-CH3OC6H4– |
10 |
3d |
96 (94) |
5 |
1e |
2-CH3OC6H4– |
10 |
3e |
71 (65) |
6 |
1f |
4-FC6H4– |
8 |
3f |
89 (82) |
7 |
1g |
4-BrC6H4– |
6 |
3g |
95 (88) |
8 |
1h |
4-IC6H4– |
8 |
3h |
82 (76) |
9 |
1i |
4-ClC6H4– |
4 |
3i |
99 (93) |
10 |
1j |
2-ClC6H4– |
12 |
3j |
97 (91) |
11 |
1k |
4-NO2C6H4– |
6 |
3k |
94 (83) |
12 |
1l |
1-Nathphyl |
12 |
3l |
53 (46) |
13 |
1m |
2-Thienyl |
12 |
3m |
50 (45) |
14 |
1n |
CH3 |
12 |
3n |
0 |
According to the reports of relative literatures, most of reactions with α-keto acids as acyl surrogates underwent through a radical process. To further verify the possibility, 2.0 equiv. of TEMPO (excellent free radical trapping species) was added into the mixture of 1a and 2 under standard condition and the formation of 3a wasn't obviously inhibited, supervised in 80% yield by GC (Scheme 1).
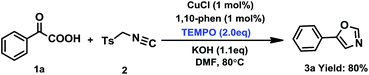 |
| Scheme 1 Control experiment. | |
The fact showed that our reaction hadn't experienced the radical mechanism. In addition, as noted during optimizing condition, the reaction didn't need any additional oxidant (Table 1, entry 9) and the copper catalyst played an indispensable role in reaction (Table 1, entry 4). All of which pointed out a novel reaction pathway involving Cu(I)-promoted might be involved. A tentative tandem reaction mechanism for the construction of monosubstituted oxazoles is described in Scheme 2. In the presence of CuCl/1,10-phen and KOH, α-oxocarboxylates II metalled by copper experience [3 + 2] cycloaddition with cuprioisocyanide III18d,19 activated similarly by copper to generate the intermediate IV. Subsequently, decarboxylation of IV followed by leaving of tosylate anion afford the aromatized oxazole products 3.
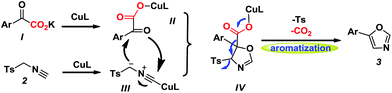 |
| Scheme 2 A plausible mechanism of the tandem cyclization–decarboxylation reaction. | |
In summary, we have disclosed an efficient and practical method for construction of monosubstituted oxazoles through tandem [3 + 2] cycloaddition–decarboxylation of isocyanide and α-keto acid reaction in one pot. Using inexpensive CuCl/1,10-phen as catalyst, we provide an elegant access to 5-aryl oxazoles under oxidant free mild condition. The method has found a novel application way for α-oxocarboxylic acids as acyl surrogates.
Experimental section
General procedure for the synthesis of 3a
To a 5 mL round bottom flask, the mixture of α-oxo-2-phenylacetic acid 1a (0.55 mmol, 83.3 mg) and KOH (0.55 mmol, 25.3 mg) in DMF (1 mL), was stirred at room temperature for 1 h. After 1a was consumed traced by TLC, TosMIC 2 (0.5 mmol, 97.6 mg) and CuCl (1 mol%, 1.3 mg)/1,10-phenanthroline (1 mol%, 0.9 mg) was added. The vial was stirring in oil bath at 80 °C for another 5 hours in air atmosphere, and monitored by TLC. Followed by TosMIC (2) was exhausted completely, the reaction was cooled down to room temperature and quenched in (10 mL) saturated NaCl solution then extracted with EtOAc (3 × 10 mL). Organic layers was combined and solvent was evaporated, then the residue was purified by flash column chromatography (petroleum ether
:
ethyl acetate = 9
:
1) to get 5-phenyloxazole 3a as yellow liquid.
Acknowledgements
Financial support for this research provided by the NSFC (Grant 21402112) is greatly acknowledged.
References
-
(a) Z. Jin, Nat. Prod. Rep., 2003, 20, 584 RSC;
(b) C.-M. Cheung, F. W. Goldberg, P. Magnus, C. J. Russell, R. Turnbull and V. Lynch, J. Am. Chem. Soc., 2007, 129, 12320 CrossRef CAS PubMed;
(c) R. A. Hughes and C. J. Moody, Angew. Chem., Int. Ed., 2007, 46, 7930–7954 CrossRef CAS PubMed;
(d) A. R. Looker, B. J. Littler, T. A. Blythe, J. R. Snoonian, G. K. Ansell, A. D. Jones, P. Nyce, M. Chen and B. J. Neubert, Org. Process Res. Dev., 2008, 12, 666–673 CrossRef CAS;
(e) Á. Pintér and G. Haberhauer, Eur. J. Org. Chem., 2008, 2008, 2375–2387 CrossRef;
(f) R. Kuang, H.-J. Shue, D. J. Blythin, N.-Y. Shih, D. Gu, X. Chen, J. Schwerdt, L. Lin, P. C. Ting, X. Zhu, R. Aslanian, J. J. Piwinski, L. Xiao, D. Prelusky, P. Wu, J. Zhang, X. Zhang, C. S. Celly, M. Minnicozzi, M. Billah and P. Wang, Bioorg. Med. Chem. Lett., 2007, 17, 5150–5154 CrossRef CAS PubMed.
-
(a) P. Querard, S. A. Girard, N. Uhlig and C.-J. Li, Chem. Sci., 2015, 6, 7332–7335 RSC;
(b) Y. Bai, W. Chen, Y. Chen, H. Huang, F. Xiao and G.-J. Deng, RSC Adv., 2015, 5, 8002–8005 RSC;
(c) W. Xu, U. Kloeckner and B. J. Nachtsheim, J. Org. Chem., 2013, 78, 6065–6074 CrossRef CAS PubMed;
(d) A. S. K. Hashmi, A. M. Schuster, M. Schmuck and F. Rominger, Eur. J. Org. Chem., 2011, 4595–4602 CrossRef CAS;
(e) J. P. Weyrauch, A. S. K. Hashmi, A. Schuster, T. Hengst, S. Schetter, A. Littmann, M. Rudolph, M. Hamzic, J. Visus, F. Rominger, W. Frey and J. W. Bats, Chem.–Eur. J., 2010, 16, 956–963 CrossRef CAS PubMed;
(f) A. S. K. Hashmi and A. Littmann, Chem.–Asian J., 2012, 7, 1435–1442 CrossRef CAS PubMed;
(g) A. S. K. Hashmi, J. P. Weyrauch, W. Frey and J. W. Bats, Org. Lett., 2004, 6, 4391–4394 CrossRef CAS PubMed;
(h) A. S. K. Hashmi, M. C. B. Jaimes, A. M. Schuster and F. Rominger, J. Org. Chem., 2012, 77, 6394–6408 CrossRef CAS PubMed;
(i) A. Stephen, K. Hashmi, M. Rudolph, S. Schymura, J. Visus and W. Frey, Eur. J. Org. Chem., 2006, 4905–4909 Search PubMed.
- A. V. Lygin and A. de Meijere, Angew. Chem., Int. Ed., 2010, 49, 9094–9124 CrossRef CAS PubMed.
-
(a) R. Heath, H. Muller-Bunz and M. Albrecht, Chem. Commun., 2015, 51, 8699–8701 RSC;
(b) D. Canseco-Gonzalez, A. Petronilho, H. Mueller-Bunz, K. Ohmatsu, T. Ooi and M. Albrecht, J. Am. Chem. Soc., 2013, 135, 13193–13203 CrossRef CAS PubMed;
(c) D. K. Tiwari, J. Pogula and D. K. Tiwari, RSC Adv., 2015, 5, 53111–53116 RSC;
(d) K. V. Kumar, T. Swaroop, N. Rajeev, A. Vinayaka, G. Lingaraju, K. Rangappa and M. Sadashiva, Synlett, 2016, 27, 1363–1366 CrossRef;
(e) D. Yamamuro, R. Uchida, M. Ohtawa, S. Arima, Y. Futamura, M. Katane, H. Homma, T. Nagamitsu, H. Osada and H. Tomoda, Bioorg. Med. Chem. Lett., 2015, 25, 313–316 CrossRef CAS PubMed.
- Y.-Y. Ma, Z.-Y. Yan, C.-L. Bian, K. Li, X.-W. Zhang, M.-F. Wang, X.-L. Gao, H. Zhang and A.-W. Lei, Chem. Commun., 2015, 51, 10524–10527 RSC.
- L. J. Gooßen, F. Rudolphi, C. Oppel and N. Rodríguez, Angew. Chem., Int. Ed., 2008, 47, 3043–3045 CrossRef PubMed.
- L.-J. Gu, C. Jin, J.-Y. Liu, H.-T. Zhang, M.-L. Yuan and G.-P. Li, Green Chem., 2016, 18, 1201–1205 RSC.
- H. Yang, L.-N. Guo and X.-H. Duan, RSC Adv., 2014, 4, 52986–52990 RSC.
- W.-M. Cheng, R. Shang, H.-Z. Yu and Y. Fu, Chem.–Eur. J., 2015, 21, 13191–13195 CrossRef CAS PubMed.
- J. Liu, Q. Liu, H. Yi, C. Qin, R.-P. Bai, X.-T. Qi, Y. Lan and A.-W. Lei, Angew. Chem., Int. Ed., 2014, 53, 502–506 CrossRef CAS PubMed.
- J. Liu, C. Fan, H.-Y. Yin, C. Qin, G.-T. Zhang, X. Zhang, H. Yi and A.-W. Lei, Chem. Commun., 2014, 50, 2145–2147 RSC.
- H. Wang, L.-N. Guo and X.-H. Duan, Chem. Commun., 2014, 50, 7382–7384 RSC.
- L. Yu, P.-H. Li and L. Wang, Chem. Commun., 2013, 49, 2368–2370 RSC.
- J. Park, M. Kim, S. Sharma, E. Park, A. Kim, S. H. Lee, J. H. Kwak, Y. H. Jung and I. S. Kim, Chem. Commun., 2013, 49, 1654–1656 RSC.
- M.-Z. Li, C. Wang, P. Fang and H.-B. Ge, Chem. Commun., 2011, 47, 6587–6589 RSC.
- M. Kim, J. Park, S. Sharma, A. Kim, E. Park, J. H. Kwak, Y. H. Jung and I. S. Kim, Chem. Commun., 2013, 49, 925–927 RSC.
-
(a) L.-J. Zhang, C.-Y. Han, Q.-Q. Dang, Y.-H. Wang and X.-M. Zhang, RSC Adv., 2015, 5, 24293–24298 RSC;
(b) Y.-H. Wang, M.-C. Xu, J. Liu, L.-J. Zhang and X.-M. Zhang, Tetrahedron, 2015, 71, 9598–9601 CrossRef CAS.
-
(a) L.-J. Zhang, X.-X. Xu, J. Tan, L. Pan, W.-M. Xia and Q. Liu, Chem. Commun., 2010, 46, 3357–3359 RSC;
(b) L.-J. Zhang, X.-X. Xu, W.-M. Xia and Q. Liu, Adv. Synth. Catal., 2011, 353, 2619–2623 CrossRef CAS;
(c) X.-X. Xu, L.-J. Zhang, X. Liu, L. Pan and Q. Liu, Angew. Chem., Int. Ed., 2013, 52, 9271–9274 CrossRef CAS PubMed;
(d) L.-J. Zhang, X.-X. Xu, Q.-R. Shao, L. Pan and Q. Liu, Org. Biomol. Chem., 2013, 11, 7393–7399 RSC;
(e) X. Liu, L.-J. Zhang, X.-X. Xu, S. Wang, L. Pan, Q. Zhang and Q. Liu, Chem. Commun., 2014, 50, 8764–8767 RSC.
-
(a) M. Gao, C. He, H.-Y. Chen, R.-P. Bai, B. Cheng and A.-W. Lei, Angew. Chem., Int. Ed., 2013, 52, 6958–6961 CrossRef CAS PubMed;
(b) P. Xiao, H.-Y. Yuan, J.-Q. Liu, Y.-Y. Zheng, X.-H. Bi and J.-P. Zhang, ACS Catal., 2015, 5, 6177–6184 CrossRef CAS;
(c) Q. Cai, F.-T. Zhou, T.-F. Xu, L.-B. Fu and K. Ding, Org. Lett., 2011, 13, 340–343 CrossRef CAS PubMed.
Footnote |
† Electronic supplementary information (ESI) available. See DOI: 10.1039/c6ra16031j |
|
This journal is © The Royal Society of Chemistry 2016 |
Click here to see how this site uses Cookies. View our privacy policy here.