DOI:
10.1039/C6RA17862F
(Paper)
RSC Adv., 2016,
6, 83312-83320
Colorimetric and fluorometric dual-mode detection of aniline pollutants based on spiropyran derivatives†
Received
13th July 2016
, Accepted 17th August 2016
First published on 18th August 2016
Abstract
The selective UV-vis absorption of spiropyran (SP) and the fluorescence quenching of a SP-cellulose material induced by aniline were observed in this study, which were exploited for rapid detection and accurate quantitative determination of aniline pollutants in the environment. The concentration of aniline pollutants can be rapidly evaluated by the naked eye in a colorimetric and fluorometric dual-mode by the SP-cellulose test paper. Furthermore, the aniline pollutants can also be accurately determined using the above materials with the aid of portable spectrophotometers. The detection processes are effective, low cost and easy to operate, and do not require personnel with expertise or cumbersome equipment, and are therefore especially suitable for real-time-field monitoring of aniline pollutants outdoors.
1. Introduction
As a prototypical aromatic amine, aniline is an essential chemical precursor and raw material widely applied in dyes,1 pharmaceuticals,2 rubber industries3 and so forth. However, due to the severe toxicity and suspected carcinogenic properties of aniline,4 any industrial leakage of aniline would jeopardize environmental security and cause serious social panic.5 Therefore, convenient and effective detection methods are needed for monitoring aniline pollutants in the environment. The current techniques available to analysts have a great variety of mechanisms and standardized procedures, for instance, coulometric titration,6 gas chromatography,7 high performance liquid chromatography (HPLC),8 super critical fluid chromatography,9 capillary electrophoresis,10 Raman scattering,11 UV-vis absorption and fluorescence spectrometry12–14 and electrochemical detection,14,15 etc. However, most of these approaches are either time consuming or highly dependent on clumsy equipment and professionals, which cannot fulfill the demands of real-time field monitoring. Most of direct electrochemical techniques need pre-separation protocol.15 The direct oxidation at bare electrode substrates tends to form polymeric by-products which could decrease its sensitivity.16 Fluorescence spectrometry has higher sensitivity, easier performance and faster response that are capable for real-time monitoring than other methods.17 Is there a convenient method like pH determination that pH values can be roughly estimated by pH indicator paper and the precise pH values can also be easily obtained via a portable pH meter? Our recent experimental results show the great possibility of utilizing the spiropyran derivative to detect the aniline pollutant in a similar way of pH test.18
As a typical photochromic molecular, spiropyran (SP) has been extensively investigated for decades since the 1950s.19 Benefit from the excellent reversible properties of its bi-stable molecular system, particularly the photoswitchable zwitterionic characteristic of its ring-open isomer merocyanine (MC), spiropyran has been widely used as a sort of chemical sensor or probe to detect metal ions and some extremely poisonous anion, e.g. Mg2+, Zn2+, Ni2+, Cu2+, Hg2+, Pd2+, and CN−, etc.,20 while the detection of organic pollutants is rarely reported maybe because of the weak interaction between the zwitterionic MC and the organic analytes. In our previous investigation of the solvent effect on photo-, solvato-chromism of spiropyran,21 an unconventional absorption of spiropyran was observed in comparison with other common organic solvents (see the normalized spectra in Fig. 1). Such a selective absorption of spiropyran implies a potentiality of spiropyran to be used as a chemical sensor for aniline detection via a colorimetric analysis, and the precise analysis can be achieved with the aid of spectrophotometers, for instance, UV-vis spectrometer. The results of UV-vis measurement, however, are often disturbed inevitably by the suspension in solutions due to scattering, especially without filtration facilities in the field monitoring. Moreover, the accuracy depends on the concentration of the solutions.22 The fluorometric analysis may dodge these disadvantages since only the emissive signals are detected. Therefore, the high sensitivity and selectivity could be obtained. Although nearly no emission is observed from the spiropyran solutions, an excellent spiropyran derived fluorescent cellulose paper has been successfully fabricated with a great enhancement of the fluorescence intensity in our previous work.23 It's widely accepted that the sensor that detects analyte interacting with molecules on the surface will increase with increasing surface area per unit mass.24 Given a porous structure and large specific surface area, cellulose paper with high quality is used extensively in filtration, absorption or enrichment of specific matters. Hence, the aforementioned fluorescent cellulose paper could serve as an ideal medium for detecting aniline.
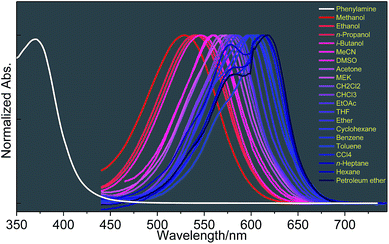 |
| Fig. 1 Absorption spectra of the SP dissolved in different solvents with distinct polarity. | |
Herein, we would like to explore the possibility of a colorimetric and fluorometric dual-mode detection of aniline pollutant based on spiropyran derivatives. Our aim is to develop a simple method to achieve a rapid and accurate detection of aniline even by non-professionals without large complex equipment, similar to that of pH measurement.
2. Experimental section
In this study, all the reagents and solvents were purchased commercially and used without further purification. All the solvents were chromatographically pure. The synthesis of 3-(3′,3′-dimethyl-6-nitrospiro[chromene-2,2′-indolin]-1′-yl) propranoic acid (SPCOOH, without special denotation, the spiropyran or SP vide infra is assigned to SPCOOH) as well as the preparation of the SP-cellulose test paper was in full accordance with our previous study.23 So it was only briefly descripted as below. 8.50 g, 27.0 mmol 1-(2-carboxyethyl)-2,3,3-trimethyl-3H-indolium bromide and 2.32 g, 21.0 mmol piperidine was dissolved in 100 mL round-bottom flask with about 20 mL distilled 2-butanone (methyl-ethyl-ketone) and then stirred for 10 min. And then 4.51 g, 27 mmol 2-hydroxy-5-nitrobenzaldehyde was added in the solution. The mixture was refluxed for 3 h in nitrogen atmosphere. The reaction solution was then placed overnight to cool down to ambient temperature. The precipitate was obtained by filtration and after dehydration crude powder (3.370 g, 33%) was obtained. The powder was further purified by silica gel column (volume ratio, acetone/chloroform, 1/1). Finally, the green-yellow powder (2.53 g, 75%) of the product was procured. The preparation of the SP-cellulose paper was illustrated in Fig. 2. It contained three main steps in sequence: preparation of cellulose pulp, synthesis of SP-cellulose pulp, and preparation of the SP-cellulose paper. 10 g scraps of high-quality filter paper were immersed in THF (300 mL) and broken into paper pulp with a high-speed blender. 5 mmol, 1.90 g SPCOOH and 1.25 mmol, 0.1527 g 4-dimethylamiopryidine (DMAP) was dissolved in 25 mL THF. Then the solution was added in the prepared cellulose pulp mixture. The mixture was stirred until it was cooled down to zero centigrade by the ice-salt bath. 5.5 mmol, 1.135 g dicyclohexylcarbodiimide (DCC) was dissolved in 20 mL THF and then was dropped into above mixture slowly with a dropping funnel. The mixture was stirred for 2 h while the temperature remained below zero centigrade. After withdrawing the ice-salt bath, the mixture was stirred for 24 h. When the reaction finished, the mixture was filtered and the precipitate was washed by THF, ethanol, and water in order to remove the residual unreacted reagents. Finally, the precipitate was distributed into 500 mL deionized water, and then it was blended into pulp again. The unreacted SPCOOH was separated from the filtrate and 0.381 g SPCOOH was recycled. The content of the SP in the SP-cellulose is about 13% (mass fraction) (see ESI†). The paper sample was prepared from the pulp via the conventional method. The procedure refers to http://www.wikihow.com/Make-Paper.
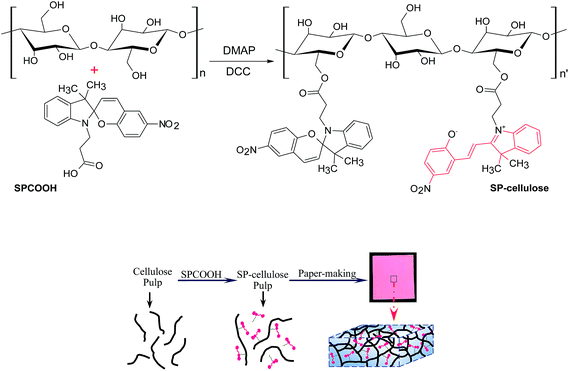 |
| Fig. 2 Schematic procedure to prepare the SP-cellulose test paper. | |
For characterization and measurements, 1HNMR spectra were recorded on a 400 MHz spectrometer (Bruker Advance 400). 13C NMR spectra were recorded on a 600 MHz spectrometer (Bruker Advance 600). UV-vis absorption spectra were recorded with a U-3010 spectrophotometer (Hitachi). The fluorescence (FL) spectra were obtained using a Fluorolog 3-P spectrofluorometer (Jobin Yvon). FTIR spectra were accomplished by Bruker Tensor 27 spectrometer. MS spectra were recorded with a LCMS-2010 mass spectrometer (Shimadzu Corporation). The thin layer chromatography (TLC) was employed to analyze the chemical constitution of the reaction solutions.
3. Results and discussion
3.1 Rapid evaluation of aniline pollutant from naked eye
As illustrated by the normalized spectra in Fig. 1, spiropyran shows nearly no absorption band in the visible region in aniline, which is quite different from the feature in other common organic solvents. Such big difference indicates that a specific interaction exists between the spiropyran and aniline molecules, and apparently, spiropyran is spectroscopically sensitive and selective to aniline, which is the foundation of quantitative determination for aniline. Generally, the variation in absorption band (intensity and λmax) in visible region denotes the color alternations. When an equal amount of spiropyran was added into a series of aniline/methanol solutions with the aniline concentration ranging from 10−7 M to 1 M, a gradual color change from heavy pink to green-yellow (Fig. 3, up photos) was obviously observed along with the increment of aniline concentration. Note that the absorption band (Fig. 1, λmax at 529 nm) in the visible region corresponds to the ring-open MC form which is more stable when spiropyran dissolves in methanol, a polar solvent.21 The trend of color change implies that the amount of MC form in solution decreases with the increment of aniline concentration from 10−7 M to 1 M.
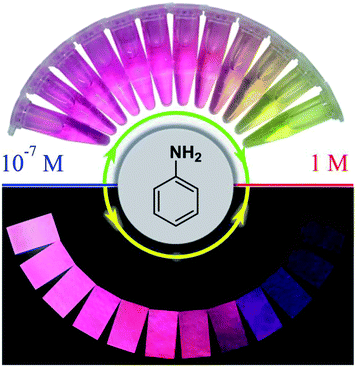 |
| Fig. 3 Photo-responses of spiropyrans to the concentration gradient of aniline at 25 °C: visible absorption change (up, SP dissolved in methanol); fluorescent intensity variation (down, the SP-cellulose test paper sample after the immersion into aniline/methanol solution for 3 min and then dried by vacuum). The concentration of aniline ranges from 10−7 M to 1 M. | |
Considering methanol is a good solvent of aniline while the actual condition is aqueous solution of aniline, in order to stimulate such circumstance, the SP-cellulose test papers or strips were immersed in the aniline/water solutions with different aniline concentration, as seen in Fig. 4. A similar color alternation from scarlet to grey was observed with increasing the aniline concentration. In addition, after the SP-cellulose test papers were immersed into a series of aniline/methanol solutions for 3 min then dried by vacuum, as seen from Fig. 3 (down fluorescent photos), the emission of the SP-cellulose test papers was quenched gradationally with increasing the concentration of aniline in methanol solutions. Similar to above absorption behavior, the fluorescence intensity of the emission band at 620 nm that is ascribed to the conjugated MC form23 declines with rising the aniline concentration from 10−7 M to 1 M.
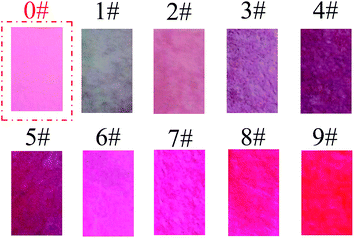 |
| Fig. 4 Color-response of the SP-cellulose test paper after the immersion into aniline/water solution at 25 °C with different concentration for 5 min: 0#, blank sample; 1#, 1 M; 2#, 0.6 M; 3#, 0.1 M; 4#, 0.01 M; 5#, 0.001 M; 6#, 10−4 M; 7#, 10−5 M; 8#, 10−6 M; 9#, 10−7 M. | |
The dependence of the color and fluorescence intensity of the SP-cellulose test papers upon the aniline concentration in Fig. 3 and 4 suggests that a colorimetric and fluorometric dual-mode analytic method could be established for rapidly and simply evaluating the concentration of aniline pollutant with the naked-eye. The standardized procedure is as follows. Firstly, the aniline standard solutions ranging from 10−7 M to 1 M are prepared. Secondly, the colorimetric or fluorometric standard cards are enacted by immersing the test papers into the standard solutions for a certain period (e.g. 3 minutes) then followed by vacuum drying process. Thirdly, the blank test paper is immersed in the unknown solution and treated with exactly the same procedure. Finally, the concentration of the unknown solution can be rapidly evaluated via simply comparing the color or emission grade with the colorimetric or fluorometric standard cards. Such rapid detection process has been principally illustrated in Fig. 5 (left panel).
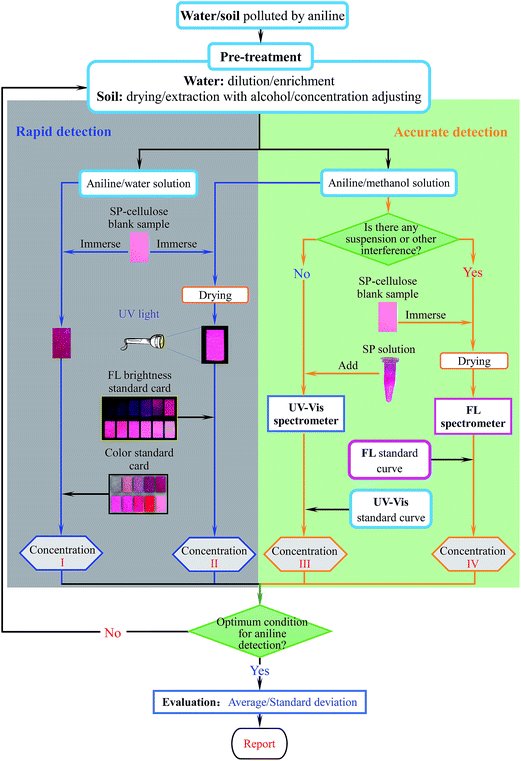 |
| Fig. 5 Flowchart for rapid and accurate detection of aniline pollutant. | |
3.2 Accurate determination of aniline with the aid of spectrometers
The method accounted before is limited by the resolution capability of the naked-eye, the accurate aniline concentration nevertheless can be determined by the colorimetric or fluorometric method with the aid of portable spectrometers. As shown in Fig. 6a, when adding an equal amount of the SP into a series of aniline/methanol solutions, two related but utterly inverse absorption variations are obtained. Specifically, the absorption at 534 nm regularly decreases while the absorption at 335 nm increases with raising the aniline concentration. Accordingly, a similar trend exists in the fluorometric method, as shown in Fig. 7a. The typical emission band of the conjugated MC form at 620 nm diminishes with the growth of the aniline concentration. It is clear that whether the colorimetric or the fluorometric method, the nonlinear fitting results indicate that all the spectra variations rigorously conform to a tri-exponential equation (eqn (1)) as bellow, even at a rather low aniline concentration (see the enlarged insets in Fig. 6b, c and 7b). |
y = y0 + A1 exp(−x/t1) + A2 exp(−x/t2) + A3 exp(−x/t3)
| (1) |
where y is the abs. or the FL intensity, x is the aniline concentration, and y0, A1, t1, A2, t2, A3, t3 are pre-exponential parameters. As listed in Table 1, the correlation efficiency (R-square) up to unity suggests that the nonlinear fitting is perfect and the aniline concentration can be accurately determined in a wide range from 10−7 M to 1 M on the basis of eqn (1), which is applicable for both the colorimetric and the fluorometric detection. Besides, there always is a Stocks shift between excitation and emission wavelength and only the emissive signals are recorded. Consequently, the scattering interference of the suspension can be completely excluded. Therefore, the fluorometric method is more appropriate for monitoring aniline pollutant in field, especially without the filtration facilities.
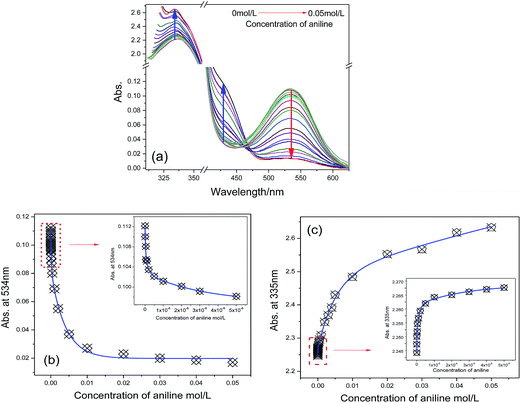 |
| Fig. 6 Absorption alterations of spiropyran in aniline/methanol solutions of different concentration at 25 °C: (a) absorption spectra; (b) absorption alteration at 534 nm; (c) absorption alteration at 335 nm. And the insets are the enlarged images of the related parts of (b) and (c). | |
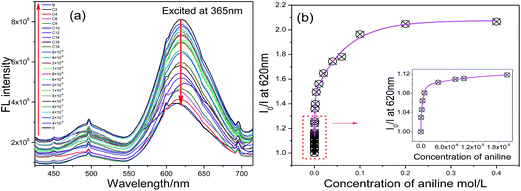 |
| Fig. 7 Fluorescence variations of the SP-cellulose test paper sample excited at 365 nm (a) and the Stern–Volmer curve of the SP-cellulose test sample with different quencher concentration (b). The inset is the enlarged image of the related part of (b). | |
Table 1 Parameters in the tri-exponential functions between aniline concentrations and the absorbance of spiropyran or the fluorescence intensity of the SP-cellulose paper
y = y0 + A1 exp(−x/t1) + A2 exp(−x/t2) + A3 exp(−x/t3) |
Parameters |
Colorimetric detection with UV-vis spectrometer |
Fluorometric detection with FL spectrometer (Stern–Volmer curve) |
534 nm |
335 nm |
y0 |
1.98300 × 10−02 |
3.25484 |
2.07528 |
A1 |
1.76800 × 10−02 |
−2.17590 × 10−01 |
−2.11015 × 10−5 |
t1 |
2.39740 × 10−04 |
5.11000 × 10−03 |
0.00111 |
A2 |
1.13000 × 10−02 |
−7.69070 × 10−01 |
−1.41852 × 109 |
t2 |
1.76872 × 10−07 |
2.31080 × 10−01 |
9.0048 × 10−7 |
A3 |
6.17200 × 10−02 |
−1.78200 × 10−02 |
−0.60356 |
t3 |
4.00000 × 10−03 |
1.62900 × 10−06 |
0.06811 |
R-Square |
0.99716 |
0.99804 |
0.99672 |
The standardized quantitative determination process is constituted as below. Firstly, the aniline standard solutions with different aniline concentration ranging from 10−7 M to 1 M are prepared. Secondly, the colorimetric or fluorometric calibration plots are established on the basis of the spectra variation in the different aniline standard solutions by UV-vis or FL measurements, in details, the absorption intensity against the aniline concentration for colorimetric method and the Stern–Volmer plot for the fluorometric method. Thirdly, the absorption or the FL intensity of the solution under test is measured. Eventually, the precise aniline concentration of the solution under test is determined according to its abs. or FL intensity values by referring to the colorimetric or fluorometric calibration curves. Such accurate determination process is principally depicted in Fig. 5 (right panel).
3.3 Error analysis on the colorimetric and fluorometric methods
As shown in Fig. 8a and b, all above colorimetric and fluorometric dual-mode detection of aniline pollutant is carried out repeatedly and assessed with error analysis. Detailed data for the error analysis can be found in Tables S1–S4 (see ESI†). As seen from Fig. 8a, for the rapid methods, the deviation of the plots from the diagonal line (y = x) for the colorimetric method is comparable to that of the fluorometric method. The minor fluctuation of the data happens at the direction of high aniline concentration (above 10−3 M). It is obvious that the precision of the rapid methods crucially depends on the resolution capability of the naked eye and it is also greatly influenced by the subjective judgment of the operator. The stability and accuracy of the data can be significantly improved with the aid of spectrometer in the cases of the accurate quantitative determination of aniline. As seen in Fig. 8b, the predicted data almost coincide with the true values on the diagonal line when the aniline concentration ranging from 10−5 M to 10−3 M. Compared with the accurate approaches, the results of the rapid detection are relatively rough. However, this method can be accomplished just as easy as the pH test and does not rely on the expertise and the cumbersome equipment, which is very crucial for the aniline monitoring and the preliminary hazard assessment in field. As the supplementary means, the methods with spectrometers can give a more precise result for the final outcome, especially the fluorometric method in which the moderate suspension can be tolerated without any damage to the ultimate results.
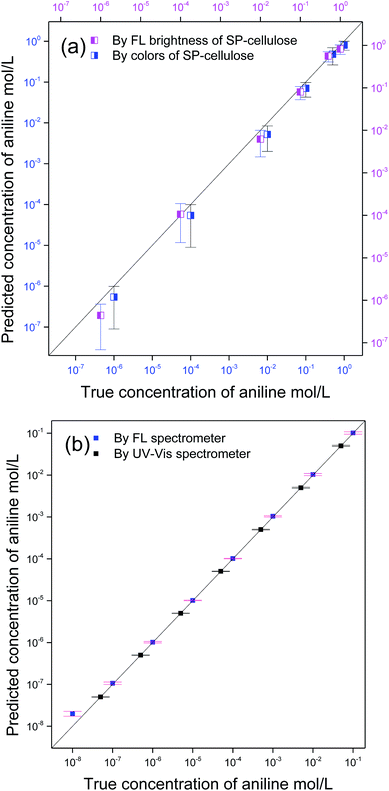 |
| Fig. 8 Comparison between the true and the predicted concentrations of aniline under different detection methods: (a), colorimetric and fluorometric methods referred to the SP-cellulose color standard card and fluorescent brightness standard card with naked eyes. (b) Detections with UV-vis and fluorescence spectrometers. The error bars denote the standard derivation and the solid diagonal line represents the reference plot of x = y. | |
3.4 Sensitivity and selectivity of the SP-cellulose for aniline detection
The results shown in Fig. 3–8 give a detection limit as low as 10−7 M and a detection range as wide as 10−7 M to 1 M. It is necessary to compare these results with those appeared in the literature. They are summarized in Table 2. As seen from Table 2, most of detections from electrochemical method give detection limit within micromolar concentration. However, by applying some special techniques such as electrochemiluminescence quenching14 and Raman spectrometer11 the detection limit can be as low as nanomolar concentration. With regard to the detection range, the highest aniline concentration is no more than 1 mM. In the case of the methods presented in this work, the detection limit of 10−7 M is almost comparable to those of lowest values and the detection range of 10−7 M to 1 M is the widest. Note that in our case besides portable spectrophotometers no other large-scale complex equipment such as electrochemical workstation or Raman spectrometer is needed. This suggests that the SP molecules are sensitive enough to aniline and the derived methods are applicable for real-time-field monitoring of aniline pollutant outdoors.
Table 2 Summary of aniline detection from references
Anilines |
Method |
Detection range |
Detection limit |
Ref. |
Aniline; no data for other anilines |
Electrochemical method |
2–40 μM |
1.4 μM |
15 |
Totally 7 aniline compounds |
Electrochemical method |
50–1 mM |
10 μM |
33 |
Aniline; no data for other anilines |
Electrochemical method |
1−140 μM |
1 μM |
34 |
Aniline and chlorinated anilines |
Fluorescence method |
Micromolar concentration range |
5 μM |
35 |
Totally 5 aniline derivatives |
Electrochemiluminescence quenching |
0.01–10 μM |
5.0 nM |
14 |
Nitroaniline |
Fluorescence titrations |
10 μM to 1 mM |
Low detection limit |
36 |
Aniline and phenol derivatives |
Raman spectrometer |
1 nM to 1 μM |
0.42–0.52 nM |
11 |
Aniline; no data for other anilines |
Quartz crystal microbalance technique |
3–50 ppm |
— |
37 |
Select aniline vapor from other anilines |
Optical and electrochemical method |
1–860 ppm |
∼1 ppm |
13 |
Aniline |
Naked eye observation |
0.1 μM to 1 M |
0.1 μM |
This work |
Aniline |
Portable spectrometers |
0.1 μM to 1 M |
0.1 μM |
This work |
The selectivity of the SP-cellulose test papers for aniline detection is also conducted in this study. As we know, only amino and phenyl are involved in aniline. The key interaction between the SP and aniline molecules, as expected, might be related to nitrogen. Thus, some nitrogen-containing organic compounds are tried. The results show that some nitrogen-containing compounds such as phenylhydrazine, p-nitroaniline, m-toluidine, and piperidine are responsive to some extent to the SP while others of pyridine, 8-hydroxyquinoline, toluene, and N,N-dimethylformamide are absolutely non-responsive. This suggests that the interaction mechanism between the SP and nitrogen-containing molecules is rather complicated and further insight research is needed that is currently being conducted in our lab. In fact, such poor selectivity for aniline detection is no surprise since only gaseous aniline can be well recognized exclusively from other aniline derivatives in the literature, as shown in Table 2.
3.5 Fluorescence quenching mechanism of spiropyran derivatives in aniline
With respect to different types of fluorescent system, there are various quenching mechanism, such as dynamic quenching, static quenching,25 charge transfer,26 fluorescence resonance energy transfer (FRET),27 etc. Aniline is one of many popular fluorescence quenchers for some specific fluorescent species, such as carboxamide,28 anthracene,29 coumarin derivative,30 and so forth. Generally, the exponential decay of the fluorescence in the presence of the quencher obeys the well-known Stern–Volmer equation.31 However, as shown in Fig. 7b, the significant negative deviation of the nonlinear Stern–Volmer curve was obtained in our experiment and it indicates that the static quenching mechanism other than the dynamic mechanism dominates the fluorescence decrease of spiropyran derivatives in the presence of aniline. And the negative deviation always involves two or more different fluorophores with different accessibility to the quencher,32 herein, in accordance with the two different isomeric forms of spiropyran species, spiropyran (SP form) and merocyanine (MC). And the reactions between photoactive molecules and quencher also can give the observed negative deviation from the Stern–Volmer law.25 Two solid evidences support such conclusion as follows. First, the appearance of the second band absorption (Fig. 6a) at lower wavelength (335 nm) and nearly no changes in emission spectra confirm the formation of non-fluorescent complex between aniline and the spiropyran species in the ground state. The TLC analysis and 1H NMR result (Fig. 9) also imply that a further reaction occurs and a new photoactive complex is generated during the quenching process. This reaction has been shown in Scheme 1. Several characterization methods of 1H NMR, 13C NMR, FTIR and ESI-MS are applied to identify the formed new substance and the results are shown in Fig. 10. Data from these methods are as below: 1H NMR (400 MHz, CDCl3) δ 8.72 (s, 1H), 8.40 (d, J = 2.7 Hz, 1H), 8.28 (dd, J = 9.2, 2.7 Hz, 1H), 7.48 (t, J = 7.7 Hz, 2H), 7.42–7.29 (m, 3H), 7.11 (d, J = 9.2 Hz, 1H), 7.26 (CDCl3); 13C NMR (600 MHz, CDCl3) δ 118.07, 118.31, 121.21, 128.04, 128.37, 129.69, 139.95, 146.72, 160.56, 166.76; FTIR (KBr, cm−1): Ar–H, 3090–3010; Ar–C, 1617, 1481; –NO2, 1533, 1290; C–N, 1344; 1,2,4-substituted aromatic ring, 831, 785; 1-substituted aromatic ring, 765, 748; –OH, 3422; ESI-MS m/z (relative intensity) 241 (M − H+, 100).
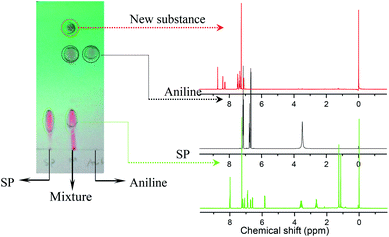 |
| Fig. 9 TLC (left) and 1H NMR (right) experimental results for separating the mixture of SP and aniline. | |
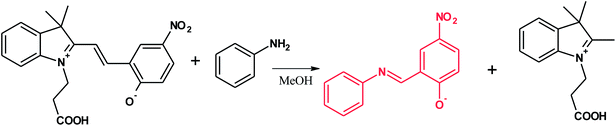 |
| Scheme 1 The reaction mechanism between the spiropyran derivatives and aniline. | |
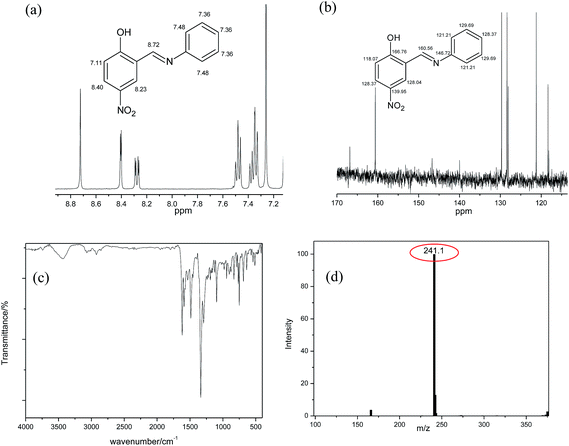 |
| Fig. 10 Characterizations of the new substance obtained from SPCOOH and aniline: (a) 1H NMR spectrum (400 MHz, CDCl3); (b) 13C NMR spectrum (600 MHz, CDCl3); (c) FTIR spectrum (KBr); (d) ESI-MS spectrum. | |
4. Conclusion
With the increase of the industrial pollutions, especially the water pollution, it need develop the chemical sensors for the specific pollutants to meet the great demand in environment monitoring urgently. In this study, we found that aniline can induce a selective absorption of spiropyran species in comparison with other common organic solvents and it also can quench the fluorescence emission of the SP-cellulose paper. Herein, a whole package of aniline determination methods including the colorimetric and the fluorometric methods was established on the basis of the specific intermolecular interactions between spiropyran and aniline. The concentration of aniline ranging from 10−7 M to 1 M can be roughly but conveniently estimated via the rapid colorimetric or fluorometric method. As the supplementary, the accurate concentration values of aniline also can be determined with the aid of spectrometers. The error analysis indicates that both the colorimetric method and the fluorometric method can give a reliable prediction for the concentration of aniline pollutant. Moreover, the colorimetric and fluorometric dual-mode methods possess the advantage that the colorimetric and the fluorometric results can be verified from each other, which can promote the validity of the determination conclusion. These detection processes are effective, low cost and easy to operate, and particularly independent to the personnel with expertise and some cumbersome equipment, especially suitable for real-time-field monitoring of aniline pollutant outdoors.
Acknowledgements
This work is financially supported by the Natural Science Foundation of Shandong Province of China (ZR2013EMM017).
References
- A. Grirrane, A. Corma and H. García, Science, 2008, 322, 1661–1664 CrossRef CAS PubMed.
- V. Alagarsamy, V. Raja Solomon and K. Dhanabal, Bioorg. Med. Chem., 2007, 15, 235–241 CrossRef CAS PubMed.
- B. G. Soares, G. S. Amorim, F. G. Souza, M. G. Oliveira and J. E. P. D. Silva, Synth. Met., 2006, 156, 91–98 CrossRef CAS.
- P. L. Skipper, M. H. Kim, H. L. Sun, G. N. Wogan and S. R. Tannenbaum, Carcinogenesis, 2009, 31, 50–58 CrossRef PubMed.
- Xinhua, Detail report on N China chemical leak, http://en.people.cn/90882/8081246.html.
- T. Kanyanee, P. Fuekhad and K. Grudpan, Talanta, 2013, 115, 258–262 CrossRef CAS PubMed.
- Y. Y. Han, L. Y. Wang, Y. Y. Zhao, Y. Q. Li and L. Y. Liu, Chromatographia, 2013, 76, 1747–1753 CAS.
- A. Sarafraz-Yazdi and Z. Es'Haghi, Chromatographia, 2006, 63, 563–569 CAS.
- T. A. Berger and W. H. Wilson, J. Chromatogr. Sci., 1993, 31, 127–132 CAS.
- S. Liu, W. Wang, J. Chen and J. Sun, Int. J. Mol. Sci., 2012, 13, 6863–6872 CrossRef CAS PubMed.
- D. Li, D. W. Li, J. S. Fossey and Y. T. Long, Anal. Chem., 2010, 82, 9299–9305 CrossRef CAS PubMed.
- C. S. Weiss, J. S. Hazlett, M. H. Datta and M. H. Danzer, J. Chromatogr. A, 1992, 608, 325–332 CrossRef CAS.
- L. Shi, C. He, D. Zhu, Q. He, Y. Li, Y. Chen, Y. Sun, Y. Fu, D. Wen, H. Cao and J. Cheng, J. Mater. Chem., 2012, 22, 11629–11635 RSC.
- X. Wang, Y. Yang and H. Gao, J. Lumin., 2014, 156, 229–234 CrossRef CAS.
- E. H. Seymour, N. S. Lawrence, E. L. Beckett, J. Davis and R. G. Compton, Talanta, 2002, 57, 233–242 CrossRef.
- E. T. Kang, K. G. Neoh and K. L. Tan, Prog. Polym. Sci., 1998, 23, 277–324 CrossRef CAS.
- S. W. Thomas, G. D. Joly and T. M. Swager, Chem. Rev., 2007, 107, 1339–1386 CrossRef CAS PubMed.
- J. T. Tian, W. G. Tian and P. Z. Gong, Peop. Rep. China Patent No. CN 104142310, Jul 18, 2014.
- Y. Hirshberg, C. R. Hebd. Seances Acad. Sci., 1950, 231, 903–904 CAS.
- M. Natali and S. Giordani, Chem. Soc. Rev., 2012, 41, 4010–4029 RSC.
- W. Tian and J. Tian, Dyes Pigm., 2014, 105, 66–74 CrossRef CAS.
- G. H. Ayres, Anal. Chem., 2002, 21, 652–657 CrossRef.
- W. G. Tian and J. T. Tian, Langmuir, 2014, 30, 3223–3227 CrossRef CAS PubMed.
- X. Wang, C. Drew, S. Lee, K. J. Senecal, J. Kumar and L. A. Samuelson, Nano Lett., 2002, 2, 1273–1275 CrossRef CAS.
- L. K. Fraiji, D. M. Hayes and T. C. Werner, J. Chem. Educ., 1992, 69, 424–428 CrossRef CAS.
- H. Knibbe, K. Röllig, F. P. Schäfer and A. Weller, J. Chem. Phys., 1967, 47(3), 1184–1185 CrossRef CAS.
- I. L. Medintz, H. T. Uyeda, E. R. Goldman and H. Mattoussi, Nat. Mater., 2005, 4, 435–446 CrossRef CAS PubMed.
- N. R. Patil, R. M. Melavanki, S. B. Kapatkar, K. Chandrashekhar, H. D. Patil and U. Siva, Spectrochim. Acta, Part A, 2011, 79, 1985–1991 CrossRef CAS PubMed.
- A. Reddy, J. Thipperudrappa, D. S. Biradar, M. T. Lagare and S. M. Hanagodimath, Indian J. Pure Appl. Phys., 2004, 42(9), 648–652 CAS.
- D. Nagaraja, R. M. Melavanki and N. R. Patil, Can. J. Phys., 2013, 91(11), 966–970 CrossRef CAS.
- H. Boaz and G. K. Rollefson, J. Am. Chem. Soc., 1950, 72(8), 3435–3443 CrossRef CAS.
- J. R. Mannekutla, B. G. Mulimani, M. I. Savadatti and S. R. Inamdar, Spectrosc. Lett., 2006, 39(4), 321–335 CrossRef CAS.
- S. Y. Ai, M. N. Gao, W. Zhang, Q. J. Wang, Y. F. Xie and L. T. Jin, Talanta, 2004, 62, 445–450 CrossRef CAS PubMed.
- T. Spătaru, N. Spătaru and K. FujishimaA, Talanta, 2007, 73, 404–406 CrossRef PubMed.
- A. S. Vangnai, N. Kataoka, T. Tajima, J. Kato, S. Soonglerdsongpha and C. Kalambaheti, J. Ind. Microbiol. Biotechnol., 2012, 39, 1801–1810 CrossRef CAS PubMed.
- Z. C. Yu, F. Q. Wang, X. Y. Lin, C. M. Wang, Y. Y. Fu, X. J. Wang, Y. N. Zhao and G. D. Li, J. Solid State Chem., 2015, 232, 96–101 CrossRef CAS.
- Y. Kosaki, H. Izawa, S. Ishihara, K. Kawakami, M. Sumita, Y. Tateyama, Q. Ji, V. Krishnan, S. Hishita, Y. Yamau chi, J. P. Hill, A. Vinu, S. Shiratori and K. Ariga, ACS Appl. Mater. Interfaces, 2013, 5, 2930–2934 CAS.
Footnotes |
† Electronic supplementary information (ESI) available: Data for error analysis, synthetic process, and characterizations. See DOI: 10.1039/c6ra17862f |
‡ These authors contributed equally to this work. |
|
This journal is © The Royal Society of Chemistry 2016 |
Click here to see how this site uses Cookies. View our privacy policy here.