DOI:
10.1039/C6RA20269A
(Communication)
RSC Adv., 2016,
6, 95165-95168
Nano-curcumin influences blue light photodynamic therapy for restraining glioblastoma stem cells growth†
Received
11th August 2016
, Accepted 22nd September 2016
First published on 22nd September 2016
Abstract
Very low doses of curcumin loaded BSA nanoparticles, following blue light mediated photodynamic therapy, provide improved cytotoxicity against glioblastoma stem cells.
Glioblastoma multiforme grade (IV) is a highly malignant brain tumour that arises from astrocytes. Approximately 15% of all primary brain tumours are GBM with an average median survival time not lasting more than 15 months; treatment results in barely 25% of patients reaching the two-year survival time.3,9 Surgery alone is not sufficient in glioblastoma treatment because of some limitations in total resection of the tumour.13,19,24 Years of refinement in the treatment procedures, which consist of surgical resection,31 followed by chemotherapy and radiotherapy, provided an overall survival benefit; however, long-term survivals are still a fairy tale. These therapies are still not able to provide complete protection from aggressive GBM tumours and ultimately result in treatment failure. Chemoresistance and radiotherapy resistance are the most common cause of such failures. Recently, cancer stem cell theory of carcinogenesis suggested the existence of a small subpopulation of glioma stem-like cells (GSCs) within these tumours. These cells contribute to tumour progression, treatment resistance, and relapse of the tumour post-treatment. The recapitulation of the tumour after aggressive surgical resection and chemo-radiotherapy suggest the presence and proliferation of cancer stem-like cells (CSCs) post-treatment.32 Concurrent radiotherapy (RT) and chemotherapy (CT) are usually not initiated until 2–4 weeks post-surgery to allow for recovery of the wound.5 Although this time gap or delay in therapy could not affect the overall survival, it can contribute to the tumour relapse and cancer progression post-surgery. The time difference between surgery and cancer radiotherapy allow CSCs to differentiate and cause relapse (Scheme 1A). Such local relapse affects 80% of cases.17,25 Collective data have established that GSCs are more resistant to conventional radiotherapy and chemotherapy than non-CSCs.18 Glioma xenografts subjected to radiation were enriched in cells expressing CD133, suggesting that radiotherapy enhances the GSCs population29 by switching the cellular hierarchy toward GSCs.20,35 Radiation and chemotherapy target highly proliferative cells and are thus ineffective on quiescent, slow-cycling GSCs. Current clinical treatments enrich the GSCs subpopulation, which over time recapitulates the tumour due to their self-renewal properties.4,15,22 A change is needed in the therapeutic approach to overcome the challenge of such self-renewal. Blue light therapy mediated by a small dose of curcumin can be a new weapon in the arsenal to overcome the barriers of radiotherapy. Although cancer cells of different origins have shown a variable response to blue light therapy,33 there is no report on its impact on cancer stem cells so far. For the first time, we report the impact of blue light therapy in combination with curcumin on GSCs (Scheme 1B). The cancer stem cells exhibit drug resistance and radioresistance through some of the very well defined mechanisms such as ATP-binding cassette (ABC) transporters.9,26 Different phytochemicals can overcome this medication resistance. In the present study, we have hypothesized and developed a novel combination therapy to prevent glioblastoma using nano-curcumin and blue light chemo-phototherapy to prevent growth of C6 cells.The majority of the population of the C6 cell line are stem cells.28 Studies on C6 glioma stem cells suggest that they proliferate in a serum-free medium, indicating that they are very difficult to control.5 Curcumin is an effective phytochemical that has shown the potential to target cancer stem cells and simultaneously provide neuroprotection. It has proved to reduce the viability of several glioma cell lines over time at concentrations as low as 10–15 μM.5 At the same time, curcumin was also shown to be non-toxic to astrocytes and cortical neurones at low levels. It sensitises radioresistant human glioma cells to radiation-induced cytotoxicity; the same effect was observed with chemotherapeutic drugs. Dietary consumption of curcumin hardly allows reaching effective concentrations in the blood. When orally administered, 8 g of curcumin per day produced 2 μM of curcumin in patients' blood,10 which is not enough to make any visible changes. Encapsulation of curcumin in BSA nanoparticles of limited dimensions provided a better formulation, which also showed better pharmacokinetics than curcumin administered as a free drug. Several nanoformulations of curcumin comprised metal oxides,1,32 liposomes,34 artificial liposomes,23 dendrimers,30 polymers,12,36 and cyclodextrins21 are available but most of them suffer from complex synthesis and toxicity constraints and cannot be used in critical biological organs such as the brain after surgery. In the current study, bovine serum albumin was used to synthesize curcumin-loaded BSA nanoparticles, which are considered as highly biocompatible and non-toxic. BSA is also utilized in incorporation of photosensitizers using a heat denaturation method;2 however, the current method is based on glutaraldehyde mediated protein crosslinking, which allows better packaging of curcumin inside BSANPs. To estimate the best combination of drug dose and blue light exposure time, cells were treated with different concentrations of the drug in combination with blue light. It was found that 2.5 min of exposure to blue light with a mean wavelength of 450 nm is sufficient in combination with a very low dose of 6 μM of curcumin to kill the majority of the glioma C6 cancer stem cells (Fig. S6†). PDT is a growing field with several limitations of therapy and procedures. Delivering light to deep tissues such as the brain is one of the major challenges in PDT, but implantable optical probes have changed the scenario for light mediated therapy in recent years. It is now possible to deliver different wavelengths of light, even to specific regions of the brain, without affecting brain functionality.8 Curcumin PDT has been effectively used in microorganisms7,14,16,27 but nano-curcumin PDT in cancer stem cells has received little attention. We have found that nano-curcumin mediation improved solubility, bioavailability and release of curcumin. In combination with blue light PDT therapy, nano-curcumin showed a promising efficacy to inhibit glioblastoma C6 stem cells, which could be difficult to achieve by conventional treatments.
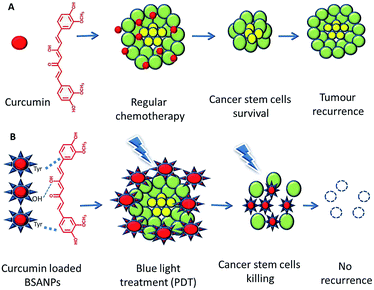 |
| Scheme 1 Comparative representation of regular chemotherapy (A) and PDT based combination therapy for glioblastoma (B). | |
Drug-loaded BSA nanoparticles (DLBSANPs) were synthesised after co-precipitation of curcumin with BSA using ethanol as a dissolving agent. Rotary evaporation was used to remove ethanol from the formulation, which allowed better entrapment of curcumin in BSA nanoparticles. For comparison purposes, blank BSA nanoparticles and a physical mixture of blank BSANPs and curcumin (PM) were also prepared and analysed.
The X-ray diffraction patterns of BSANPs, curcumin, curcumin loaded BSANPs, curcumin and PM were compared. Curcumin showed diffraction peaks at 2θ of 16.82°, 17.72°, 20.78°, 24.07° and 24.22°; these peaks were also observed in the PM sample (Fig. S4†). However, DLBSANPs did not have the same diffraction pattern, which indicated that curcumin was entirely entrapped inside the BSANPs.
Field Emission Scanning Electron Microscopy (FESEM) revealed the nearly spherical nature of BSANPs and DLBSANPs, in the range of 90–100 nm (Fig. 1A and B). Dynamic light scattering measurements showed a mean size of 106 nm (PDI = 0.058) and 126 nm (PDI = 0.149) for BSANPs and DLBSANPs, respectively (Table S1†).
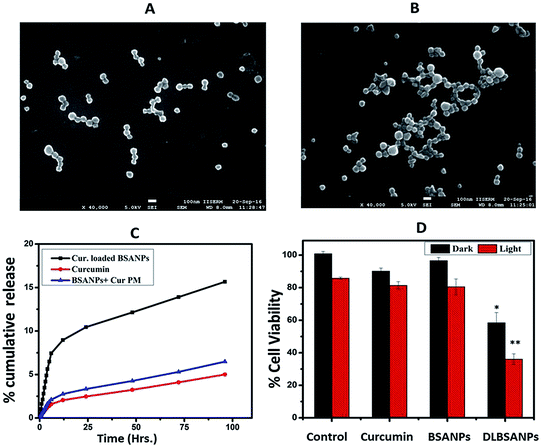 |
| Fig. 1 FESEM image of BSANPs (A), DLBSANPs (B), in vitro drug release profile (C), MTT assay at 24 h comparing control, curcumin, BSANPs, and DLBSANPs in presence and absence of blue light (D). | |
Fourier transformation infrared (FTIR) analysis was performed to study the interaction between BSANPs and curcumin and simultaneously to explore the presence of the drug inside the nanoformulation. From the analysis point of view, only the region of interest is shown (Fig. S2†). BSANPs exhibited a peak at 1656 cm−1, which was a characteristic of an amide I bond, present in all three samples (BSANPs, DLBSNPs, and PM). Curcumin displayed characteristic peaks at 3507 cm−1 (–OH vibrations), 1628 cm−1 (–C
O), 1604 cm−1 (aromatic C
C), 1427 cm−1 (phenol C–O), and 1281 cm−1 (enol C–O), which were also present in all three samples. In addition to these, some additional peaks were also present in DLBSANPs: a broad peak at 3283 cm−1 (intermolecular hydrogen bonding) and a small and sharp peak at 3175 cm−1, indicating some interaction between curcumin and BSANPs. Overall, this pattern was attributed to the hydrophobic interaction between phenolic groups in curcumin and tryptophan residues of BSA, in addition to some intermolecular hydrogen bonding interactions. Moreover, since DLBSANPs contained most of the characteristic peaks of curcumin and only some of the characteristic peaks of BSANPs, a change in structure of BSA during formation of NPs and after drug loading was inferred. Alteration in the secondary structure of BSA during synthesis of nanoparticles was examined using CD spectroscopy. The structural changes from alpha helix towards beta sheet were mediated by drug interaction and nanoformulation of the protein (Fig. S3†).
The solubility of curcumin was determined by preparing saturated solutions of CUR, DLBSANPs and PM in PBS at pH 7.2 to reach the equilibrium concentration. Curcumin solubility was increased nearly by two-fold via DLBSANPs (Fig. S5†), an observation that was attributed to interaction between curcumin and BSA. This increased solubility was also seen in the in vitro release profile performed in PBS 7.2 as a dissolution medium. Photodynamic therapy was performed using blue LED light with an average light wavelength of 450–480 nm. Rat glioma C6 cells were seeded in a 24 well plate at the density of 1.5 × 104 on the day prior to the experiment. Once the cells formed a monolayer, they were treated with different concentrations of the drug loaded nanoparticles, placebo nanoparticles, and drug for six h using lower serum media. After treatment, cells were washed thrice with chilled PBS at pH 7.2 and the incomplete medium was added. After removing the media, blue light was applied at a fixed distance of 17 mm for 2.5 min to make an overall light dose of 1000 J cm−2. After exposure, cells were supplemented with 2% FBS DMEM media and incubated overnight. MTT assay was performed at 24 h, along with a control that was untreated with blue light PDT (Fig. 1D).
Cell cytotoxicity studies using MTT assay on C6 cancer stem cells were conducted in the presence and absence of blue light. BSANPs showed non-significant cell toxicity in comparison to the untreated control in dark conditions after 6 h incubation with the cells. These results confirmed earlier reports of BSA nanoparticles as a better and more cell friendly drug carrier system.10 Drug loaded BSANPs treated cells showed higher cytotoxicity compared to the bare drug at the same concentration of 6 μM in PBS 7.2 because of higher surface to mass ratio of drug provided by nanodimensions. Cytotoxicity of DLBSANPs significantly increased after treatment with blue light PDT because of the better distribution of nano photosensitizer (curcumin) inside the cells.
The superior cytotoxicity of DLBSANPs could be attributed to the enhanced and sustained release of curcumin, as demonstrated from the in vitro dissolution studies (Fig. S5†) and in vitro drug release studies (Fig. 1C).
Spectroscopic properties of BSA and curcumin interaction indicated a strong interaction (Fig. S1†), which allowed the sustained release of the drug and a prolonged period of drug exposure. Curcumin-loaded BSANPs significantly inhibited the growth of C6 cancer stem cells at a very low dose of curcumin (6 μM), following blue light PDT, which is strikingly considered as non-lethal for healthy cell growth.6 The combination of chemotherapy with phototherapy induced more cell death in comparison to the chemotherapy alone (Fig. S6†). Time and distance of exposure of blue light are the crucial factors that form the perfect deadly combination for C6 glioma cancer stem cells in the current experiment. The molecular mechanisms are yet to be established.
Curcumin is a multi-action drug that targets different pathways inside the body. Mitochondria proteolytic based apoptotic pathways11 are activated by curcumin, whereas phototherapy starts an ROS-mediated response that guides cancer stem cells towards autophagy, apoptosis or programmed necrosis. Increased toxicity of curcumin loaded BSANPs was conclusively due to their increased endocytosis, which may be the result of better intracellular distribution of the drug. The current formulation was tried for the C6 glioma cancer stem cells only, but could be easily applied to other types of cancer stem cells.
Conclusions
In summary, we designed a protocol that can be very useful following tumour resection in glioblastoma. Curcumin-loaded BSANPs formulation, in combination with blue light mediated phototherapy, can provide prolonged prevention towards recurrence of the tumour through diminishing tumour stem cells and may hasten the wound healing process following craniotomy.
Acknowledgements
This study is partially supported by the University Grant Commission JRF in Science Fellowship (Sr. No. 2121330542 Ref no. 22/12/2013 (ii) EU-V) and the Science and Engineering Research Board (SERB) grants: YSS/2015/001706 and ECR/2016/000633/LS. We also thank Mr Inderjit Singh (IISER, Mohali) for capturing FESEM images of the samples.
References
- A. k. M. Prasanna Lakshmi Abbaraju, S. Jambhrunkar, J. Zhang, C. Xu, A. Popat and C. Yu, J. Mater. Chem. B, 2014, 2, 8298–8302 RSC
. - A. R. S. Marcilene, M. A. Rodrigues, F. L. Primo, M. P. Siqueira-Moura, P. C. Morais and A. C. Tedesco, J. Magn. Magn. Mater., 2009, 1600–1603, DOI:10.1016/j.jmmm.2009.02.093
. - American Brain Tumor Association, http://www.abta.org/braintumor-information/types-of-tumors/glioblastoma.html.
- B. Campos, Z. Gal, A. Baader, T. Schneider, C. Sliwinski, K. Gassel, J. Bageritz, N. Grabe, A. von Deimling, P. Beckhove, C. Mogler, V. Goidts, A. Unterberg, V. Eckstein and C. Herold-Mende, J. Pathol., 2014, 234, 23–33 CrossRef PubMed
. - C. M. Andrea Pirzkall, S. Saraswathy, S. Cha, R. Liu, K. R. L. Scott Vandenberg, M. S. Berger, S. M. Chang and a. S. J. Nelson, Neuro-Oncology, 2008, 11(6), 842–852 CrossRef PubMed
. - D. Fong, A. Yeh, R. Naftalovich, T. H. Choi and M. M. Chan, Cancer Lett., 2010, 293, 65–72 CrossRef CAS PubMed
. - D. P. Leite, F. R. Paolillo, T. N. Parmesano, C. R. Fontana and V. S. Bagnato, Photomed. Laser Surg., 2014, 32, 627–632 CrossRef CAS PubMed
. - E. S. B. Anthony, N. Zorzos and C. G. Fonstad, Opt. Lett., 2010, 35, 4133–4135 CrossRef PubMed
. - F. Kratz, J. Controlled Release, 2008, 132, 171–183 CrossRef CAS PubMed
. - H. C. Cheng AL, J. K. Lin, M. M. Hsu, Y. F. Ho, T. S. Shen, J. Y. Ko, J. T. Lin, B. R. Lin, W. Ming-Shiang, H. S. Yu, S. H. Jee, G. S. Chen, T. M. Chen, C. A. Chen, M. K. Lai, Y. S. Pu, M. H. Pan, Y. J. Wang, C. C. Tsai and C. Y. Hsieh, Anticancer Res., 2001, 21, 2895–2900 Search PubMed
. - J. K. Park, T. Hodges, L. Arko, M. Shen, D. Dello Iacono, A. McNabb, N. Olsen Bailey, T. N. Kreisl, F. M. Iwamoto, J. Sul, S. Auh, G. E. Park, H. A. Fine and P. M. Black, J. Clin. Oncol., 2010, 28, 3838–3843 CrossRef PubMed
. - J. Shaikh, D. D. Ankola, V. Beniwal, D. Singh and M. N. Kumar, Eur. J. Pharm. Sci., 2009, 37, 223–230 CrossRef CAS PubMed
. - K. L. Chaichana, I. Jusue-Torres, R. Navarro-Ramirez, S. M. Raza, M. Pascual-Gallego, A. Ibrahim, M. Hernandez-Hermann, L. Gomez, X. Ye, J. D. Weingart, A. Olivi, J. Blakeley, G. L. Gallia, M. Lim, H. Brem and H. Quinones, Neuro-Oncology, 2013, 16, 113–122 CrossRef PubMed
. - L. N. Dovigo, A. C. Pavarina, A. P. Ribeiro, I. L. Brunetti, C. A. Costa, D. P. Jacomassi, V. S. Bagnato and C. Kurachi, Photochem. Photobiol., 2011, 87, 895–903 CrossRef CAS PubMed
. - L. Li and R. Bhatia, Clin. Cancer Res., 2011, 17, 4936–4941 CrossRef CAS PubMed
. - M. C. Andrade, A. P. Ribeiro, L. N. Dovigo, I. L. Brunetti, E. T. Giampaolo, V. S. Bagnato and A. C. Pavarina, Arch. Oral Biol., 2013, 58, 200–210 CrossRef CAS PubMed
. - M. D. Kent, E. Wallner, J. H. Galicich, M. D. George Krol, M. D. Ehud Arbit, M. D. And Mark, G. Malkin and M. D. Frcpc, Int. J. Radiat. Oncol., Biol., Phys., 1989, 16, 1405–1409 CrossRef
. - M. F. Clarke, J. E. Dick, P. B. Dirks, C. J. Eaves, C. H. Jamieson, D. L. Jones, J. Visvader, I. L. Weissman and G. M. Wahl, Cancer Res., 2006, 66, 9339–9344 CrossRef CAS PubMed
. - M. J. McGirt, D. Mukherjee, K. L. Chaichana, K. D. Than, J. D. Weingart and Q. Hinojosa, Neurosurgery, 2009, 65, 463–470 CrossRef PubMed
. - M. Jackson, F. Hassiotou and A. Nowak, Carcinogenesis, 2015, 36, 177–185 CrossRef CAS PubMed
. - M. M. Yallapu, M. Jaggi and S. C. Chauhan, Colloids Surf., B, 2010, 79, 113–125 CrossRef CAS PubMed
. - N. Moore and S. Lyle, J. Oncol., 2011, 1–11 CrossRef PubMed
. - N. Pawar, K. Rawat and H. B. Bohidar, RSC Adv., 2016, 6, 73677–73682 RSC
. - National Brain Tumor Society, http://braintumor.org/brain-umorinformation/understanding-braintumors/tumor-types/.
- P. A. Hochberg FH, Neurology, 1980, 30, 907–911 CrossRef PubMed
. - P. Agostinis, K. Berg, K. A. Cengel, T. H. Foster, A. W. Girotti, S. O. Gollnick, S. M. Hahn, M. R. Hamblin, A. Juzeniene, D. Kessel, M. Korbelik, J. Moan, P. Mroz, D. Nowis, J. Piette, B. C. Wilson and J. Golab, Ca-Cancer J. Clin., 2011, 61, 250–281 CrossRef PubMed
. - P. Soria-Lozano, Y. Gilaberte, M. P. Paz-Cristobal, L. Perez-Artiaga, V. Lampaya-Perez, J. Aporta, V. Perez-Laguna, I. Garcia-Luque, M. J. Revillo and A. Rezusta, BMC Microbiol., 2015, 15(187), 1–8 Search PubMed
. - P. D. Brown, M. J. Maurer, T. A. Rummans, B. E. Pollock, K. V. Ballman, J. A. Sloan, B. F. Boeve, R. M. Arusell, M. M. Clark and J. C. Buckner, Neurosurgery, 2005, 57, 495–504 CrossRef PubMed
. - S. Bao, Q. Wu, R. E. McLendon, Y. Hao, Q. Shi, A. B. Hjelmeland, M. W. Dewhirst, D. D. Bigner and J. N. Rich, Nature, 2006, 444, 756–760 CrossRef CAS PubMed
. - S. D. Wei Shi, S. Rizk, A. Hussain, H. Tariq, S. Averick, W. L. Amoreaux, A. El Idrissi, P. Banerjee and K. Raja, Org. Lett., 2007, 9, 5461–5464 CrossRef PubMed
. - S. K. Singh, I. D. Clarke, M. Terasaki, V. E. Bonn, C. Hawkins, J. Squire and P. B. Dirks, Cancer Res., 2003, 63, 5821–5828 CAS
. - S. Karmakar, S. Jambhrunkar, A. Popat, M. Yu and C. Yu, RSC Adv., 2014, 4, 709–712 RSC
. - S. L. Hopkins, B. Siewert, S. H. Askes, P. Veldhuizen, R. Zwier, M. Heger and S. Bonnet, Photochem. Photobiol. Sci., 2016, 15, 644–653 CAS
. - S. Mourtas, A. N. Lazar, E. Markoutsa, C. Duyckaerts and S. G. Antimisiaris, Eur. J. Med. Chem., 2014, 80, 175–183 CrossRef CAS PubMed
. - T. Reya, S. J. Morrison, M. F. Clarke and I. L. Weissman, Nature, 2001, 414, 105–111 CrossRef CAS PubMed
. - Z. Song, R. Feng, M. Sun, C. Guo, Y. Gao, L. Li and G. Zhai, J. Colloid Interface Sci., 2011, 354, 116–123 CrossRef CAS PubMed
.
Footnote |
† Electronic supplementary information (ESI) available. See DOI: 10.1039/c6ra20269a |
|
This journal is © The Royal Society of Chemistry 2016 |
Click here to see how this site uses Cookies. View our privacy policy here.