DOI:
10.1039/C6RA21191G
(Review Article)
RSC Adv., 2016,
6, 96154-96175
Current advances of organocatalytic Michael–Michael cascade reaction in the synthesis of highly functionalized cyclic molecules
Received
23rd August 2016
, Accepted 1st October 2016
First published on 3rd October 2016
Abstract
Organocatalytic domino/cascade reactions provide a convenient method for the construction of highly functionalized cyclic molecular structures bearing multiple stereocenters in a highly stereoselective fashion. A wide range of organocatalysts used for the construction of these architecturally complex molecules are reported. A distinct activation mode of monofunctional and bifunctional catalysts with different additive and bases used for synergistic co-operative activation also presented. The strategies and catalyst system described here highlight recent advances in the enantioselective synthesis of poly functionalized cyclic molecules via organocascade (Michael–Michael) reactions. This review focuses on the enantioselective synthesis of cyclic molecules via organocascade reactions and is organized on the basis of four categories of activation such as enamine–iminium catalysis, iminium–enamine catalysis, base–base catalysis and acid–base catalysis (bifunctional catalysis).
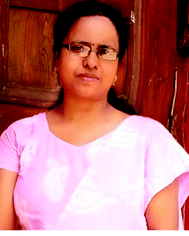 Sabita Nayak | Sabita Nayak was born in Barabati, Odisha, India in 1975. She completed her Ph.D. under the supervision of Dr Mukund K Gurjar from National Chemical Laboratory, Pune, India, in 2008 and after that she worked as a postdoctoral fellow in the research group of Prof. J R Falck at southwestern medical research center in Dallas, Texas, USA till December 2009. She joined as an Asst Professor in Chemistry, Ravenshaw University, Cuttack, Odisha, India in March 2010 and continuing. Her research interests include organocatalyst mediated Michael addition reactions/domino reactions/bioactive hybrid natural product synthesis. |
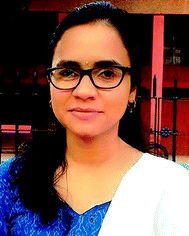 Pravati Panda | Pravati Panda was born in 1991 in Cuttack, Odisha, India. She completed her M.Sc. Chemistry from Ravenshaw University, India in 2013. She joined the Ph.D. programme at Ravenshaw University, Cuttack, Odisha in 2013. Currently she is continuing her Ph.D. work under the supervision of Dr Sabita Nayak at Ravenshaw University, Cuttack, India. She also cleared CSIR-NET exam in Chemical Sciences in 2014. Her research interests include the synthesis of biologically active molecules and organocatalytic Michael addition reactions. |
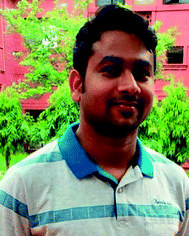 Sujitlal Bhakta | Sujitlal Bhakta was born in Balasore, Odisha, India in 1989. He did his graduation in chemistry honours at Fakir Mohan Autonomous college, Balasore, he received his master degree in chemistry at Ravenshaw University, Cuttack and doing Ph.D. degree under the supervision of Dr Seetaram Mohapatra at Ravenshaw University, Cuttack in 2012. He has cleared GATE-2014. His research interest include organocatalyst mediated chromene synthesis and their application for the synthesis of bioactive hybrid natural product. |
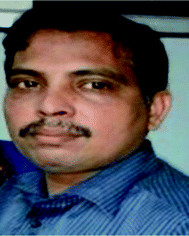 Sambita Kumar Mishra | Sambita Kumar Mishra was born in Baradihi, Sarankul, Nayagarh, Odisha, India in 1975. He completed his M.Sc. Chemistry from Utkal University in 1997. He received his M.Phil Chemistry from Utkal University in 1999. Currently he is continuing his Ph.D. work under the supervision of Dr Sabita Nayak at Ravenshaw University, Cuttack, India. His research interests include the synthesis of biologically active spirocyclic molecules and organocatalytic Michael addition reactions. |
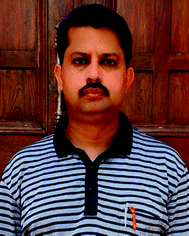 Seetaram Mohapatra | Seetaram Mohapatra was born in Krushnachandrapur, Odisha, India in 1978. He completed his Ph.D. under the supervision of Dr Mukund K. Gurjar from National chemical laboratory, Pune University, India, in 2008 and after that he worked as a postdoctoral research associate in University of Texas in the research group of Prof. J. R. Falck until December 2009. Then he joined as a lecturer in 2010 in Utkal University. After that in 2011, he joined as an Asst Professor in Ravenshaw University, Cuttack, Odisha, India and continuing. His research interest include the synthesis and application of chiral organocatalyst. |
1. Introduction
Organocatalytic reactions in the past few years emerged as a powerful tool for the preparation of optically active compounds with structural diversity from simple and easily available starting materials. Efficient and elegant synthesis of complex organic molecules with multiple stereogenic centres in continue to be important in both academic and industrial laboratories. In particular, catalytic asymmetric multi-component ‘domino’ reactions, used during total syntheses of natural products and synthetic building blocks, are highly desirable. The rapid growth in organocatalysis, over the last decades has dramatically changed the profile of asymmetric catalysis.1 Organocatalytic asymmetric Michael addition reactions can be considered as one of the most powerful and reliable tool for the stereo controlled formation of C–C and C–X (X = O, N, S) bonds.2 Michael–Michael cascade reaction is a powerful tool in forgoing ring systems common to many bioactive natural and synthetic products.3 A number of organocatalytic double Michael addition reactions have been reported in the last few years. This reaction is opened a new range of opportunities for the efficient asymmetric synthesis of complex molecular cyclic structures bearing several stereogenic centers in operationally simple procedure by utilizing readily available precursors. These one-pot transformations also possess high synthetic efficiency by decreasing the number of laboratory operations as well as the quantities of reagents and solvents used, thus saving time, costs and working steps.4
In the beginning, the double Michael addition reactions were governed by the enamine/iminium intermediates using various proline derivatives and cinchona based primary amine catalyst, pioneered by Melchiorre and Wang.5,6 Latter on various group followed this cascade reaction for the synthesis of enantiomerically enriched cyclic compounds.7 As time progressed various basic catalyst such as bisphosphine and amine derivatives were also used for this domino reaction.8 With respect to time, in this field various organocatalyst showing noncovalent catalysis came to the limelight for their higher reactivity and stereoselectivity.9 The significant contributions have been made by bifunctional catalyst, thiourea derivatives (hydrogen bonding catalysis) and squaramide derivatives (hydrogen bonding).10–12
This review addresses the most significant synthetic methods reported on organocatalyst mediated tandem Michael addition reaction of C/N/O/S atom-centered nucleophiles to electron deficient alkenes for the enantioselective and diastereoselective construction of poly functioned carbocyclic, heterocyclic, bicyclic and spirocyclic system in optically enriched forms.
Here, we discuss the enamine–iminium, iminium–enamine, base–base and acid–base catalyzed double Michael addition reactions. We aim to provide information about the current development in this exciting field from 2007–2016.
2. Enamine–iminium activated double Michael addition reactions
2.1. Double Michael addition reactions of enones catalyzed by chiral cinchona
Paolo Melchiorre et al.5 in 2009 developed a method for the construction of a series of diastereomeric mixture of tetra substituted cyclohexanone 5a and 5b through double Michael addition reaction of aliphatic/aromatic enones 1 with nitro olefin 2. The cinchona based catalyst 8 provided 5a and 5b in moderate to good yield, moderate to good enantioselectivities and good diastereomeric ratios. Replacing nitro olefin 2 with α,β-unsaturated nitrile 3 afforded the corresponding cyclohexanone 6 with four stereogenic centres in moderate to good yield, good to very good enantioselectivities and good diastereomeric ratios. The reaction of enone 1 with maleimides 4 resulted in 7 with 34–72% yield, 96 to >99% ee and 7
:
1 to >19
:
1 diastereomeric ratios. The plausible transition state mechanism for the domino reaction is shown in below (Scheme 1).
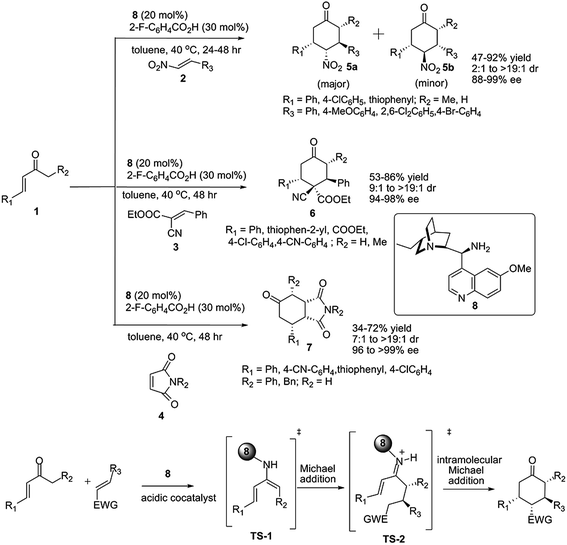 |
| Scheme 1 Organocascade reactions of enones catalyzed by a chiral cinchona. | |
2.2. Double Michael addition reactions of α,β-unsaturated ketones with unsaturated pyrazolones catalyzed by chiral cinchona
Rui Wang group6 in 2013 reported an interesting methodology for the synthesis of spiropyrazolone molecules with an extension of substrate scope. The authors reported a primary cinchona amine 11 catalyzed double Michael cascade reaction between α,β-unsaturated ketones 10 and unsaturated pyrazolones 9 which provides spiropyrazolones core 12 structure containing two intervals or three consecutive stereogenic centers, including a spiro quaternary center with excellent diastereo- (>20
:
1 dr) and enantioselectivities (up to 99% ee) (Scheme 2).
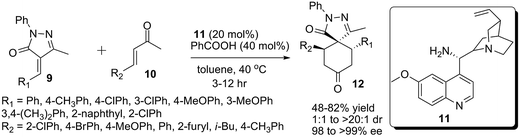 |
| Scheme 2 Double Michael addition reaction between unsaturated ketones and pyrazolones. | |
2.3. Double Michael addition reactions of nitro olefins with aldehyde esters catalyzed by chiral proline like molecule
Taking the advantage of proline like molecules as organocatalyst Delong Liu and Wanbin Zhang group in 2013,7 disclosed a new way for the synthesis of tetra substituted cyclopentane. The biologically important and synthetically challenging cyclopentane 16 with four contiguous stereocenters was constructed via an asymmetric domino double Michael addition reaction of nitro olefins 13 and aldehyde esters 14 with good yield (up to 98%) and excellent diastereo (up to 100% dr) and enantioselectivities (up to 99% ee) by using organocatalyst 15. The influence of R2 group in 14 had a large impact on the progress of the reaction. Presence of aliphatic group increases the yield, whereas aromatic group decreases the yield of the reaction (Scheme 3).
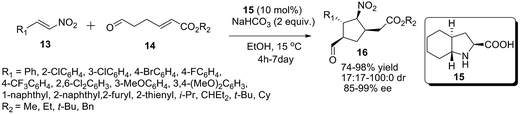 |
| Scheme 3 Asymmetric domino double Michael addition of nitro olefins and aldehyde–esters with trans-perhydroindolic acid as an organocatalyst. | |
2.4. Double Michael addition reactions of 3-nitro-2H-chromenes with α,β-unsaturated ketones catalyzed by chiral cinchona
The last example in this section was reported very recently in 2015 by Da-Ming Du group13 by taking the advantage of quinidine derived primary amine 19 for this cascade reaction. The combination of the primary amine 19 and benzoic acid as additive catalyzed cascade double Michael addition reaction between 3-nitro-2H-chromenes 17 and α,β-unsaturated ketones 18 provided an easy access to highly functionalized tetrahydro-6H-benzo[c]chromenes 20 with three contiguous stereocenters in moderate to good yields (45–90%), with excellent diastereoselectivities (10
:
1 to >25
:
1 dr) and moderate to excellent enantioselectivities (65–95% ee). Here additive plays a major role for the progress of the reaction. When no additive was used the yield as well as the enantioselectivity of the reaction decreases (yield 28%, ee 10%) (Scheme 4).
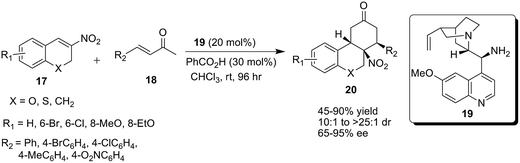 |
| Scheme 4 Double Michael addition reaction of 3-nitro-2H-chromenes with α,β-unsaturated ketones. | |
3. Iminium–enamine activated double Michael addition reactions
3.1. Double Michael addition reactions of α,β-unsaturated aldehyde with α,β-unsaturated ester catalyzed by chiral (S)-diphenylprolinol TMS ether catalyst
Taking the advantage of (S)-diphenylprolinol TMS ether (iminium–enamine based catalysis) 23, Wei Wang group in 2007 designed a Michael–Michael cascade reaction between α,β-unsaturated aldehyde 21 and α,β-unsaturated ester 22 which provided the desired tetra substituted cyclopentane 24 having three stereocenters with high levels of enantio-and diastereo control in a single operation.14 The possible mechanism of the reaction is shown in below (Scheme 5).
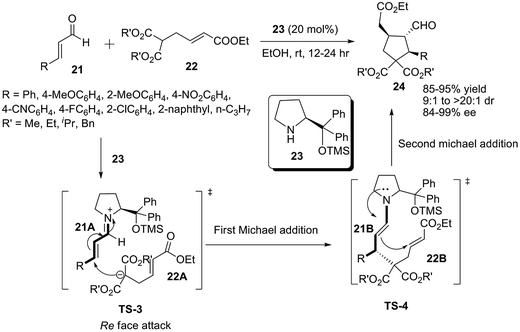 |
| Scheme 5 Double Michael addition reaction of α,β-unsaturated ester with α,β-unsaturated aldehyde. | |
3.2. Double Michael addition reactions of α,β-unsaturated aldehyde with trans-ethyl-4-mercapto-2-butenoate catalyzed by chiral (S)-diphenylprolinol TMS ether catalyst
Soon thereafter successful synthesis of polysubstituted carbocyclic moiety in the 2007,14 Wei Wang group15 in the same year expanded their methodology to heterocyclic system. They utilized the same catalyst, but varied a little in the substrate scope. The (S)-diphenylprolinol TMS ether 23 catalyzed domino thia Michael–Michael addition reaction between a variety of α,β-unsaturated aldehyde 25 and trans-ethyl-4-mercapto-2-butenoate 26 afforded the highly functionalized chiral tetrahydrothiophenes 27 with generation of three new stereogenic centers in excellent enantioselectivities (94–99% ee) and good dr (6
:
1–18
:
1) (Scheme 6).
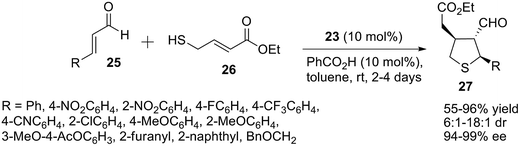 |
| Scheme 6 Enantioselective organocatalytic thia Michael–Michael addition reactions. | |
3.3. Double Michael addition reactions of α,β-unsaturated aldehyde with trans-γ-Ts protected amino α,β-unsaturated ester catalyzed by chiral (S)-diphenylprolinol TMS ether catalyst
After successful synthesis of poly substituted tetrahydrothiophene moiety by the thia-Michael–Michael addition in 2007,15 Wei Wang group16 after one year in 2008 again presented a similar type of synthesis of poly substituted pyrrolidine moiety 30 by taking advantage of same catalyst. The (S)-diphenylprolinol TMS ether 23 catalyzed domino aza-Michael–Michael addition reactions between α,β-unsaturated aldehyde 28 with trans-γ-Ts protected amino α,β-unsaturated ester 29 afforded the corresponding pyrrolidine moiety 30 with three contiguous stereocenters in good yield (80–94%) with excellent enantioselectivity (96 to >99% ee) and high diastereoselectivity (7
:
1 to >30
:
1 dr) (Scheme 7).
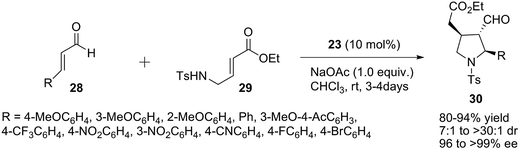 |
| Scheme 7 Highly enantio- and diastereoselective organocatalytic cascade aza-Michael–Michael reactions. | |
3.4. Double Michael addition reactions of 7-oxohept-2-enoate with nitro styrene catalyzed by chiral (S)-diphenylprolinol TMS ether catalyst
Bor-Cherng Hong et al.17 in 2008 reported an organocatalytic conjugate Michael addition of 7-oxohept-2-enoate 31 to nitro styrene 32 provided an intermediate ω-nitro-α,β-unsaturated ester 33, which on subsequent intramolecular cyclization afforded the highly functionalized tetra substituted cyclohexane carbester 34 with four contiguous stereogenic centers with high diastereoselectivity and high enantioselectivity (>99% ee) (Scheme 8).
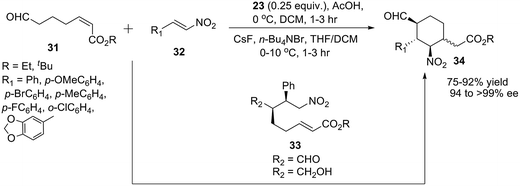 |
| Scheme 8 Prolinol-TMS ether catalyzed double Michael reaction of 7-oxohept-2-enoates and nitro styrene. | |
3.5. Double Michael addition reactions of α,β-unsaturated aldehyde with a β-keto ester catalyzed by chiral (S)-diphenylprolinol TMS ether catalyst
Using same catalyst 23, asymmetric Michael–Michael reaction of an α,β-unsaturated aldehyde 36 with a β-keto ester 35 afforded the poly substituted cyclopentanone 37 having four contiguous chiral center, and among them two are quaternary with excellent enantioselectivity was reported by Dawei Ma et al. in 2010 (Scheme 9).18
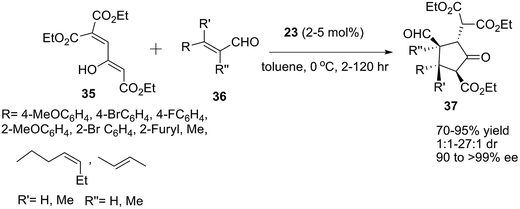 |
| Scheme 9 Double Michael addition reactions of β-keto ester and α,β-unsaturated aldehyde. | |
3.6. Double Michael addition reactions of alkynals with o-hydroxy-β-nitrostyrenes catalyzed by chiral (S)-diphenylprolinol TBDMS ether catalyst
Some years later, Wei Wang group in 2010 disclosed a new atom economic approach for the synthesis of 4H-chromenes 41 moiety by using iminium–allenamine cascade catalysis.19 The diphenylprolinol TBDMS ether 40 catalyzed cascade oxa-Michael–Michael reaction sequence between alkynals 39 and o-hydroxy-β-nitrostyrenes 38 provided the trisubstituted chiral 4H-chromenes 41 moiety in high yield (92–98%) with excellent enantioselectivity (98 to >99% ee) in toluene (Scheme 10).
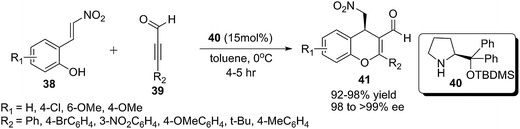 |
| Scheme 10 Enantioselective cascade oxa-Michael–Michael reaction of alkynals with 2-(E)-(2-nitrovinyl)-phenols. | |
3.7. Double Michael addition reactions of dienones with malononitrile catalyzed by chiral cinchona
A primary amine 11 and acid (TFA)-additive mediated domino double Michael addition reaction of dienones 42 with malononitrile 43 afforded the synthesis of polysubstituted cyclohexanones 44 in good yield (7–87%), diastereoselectivities (4
:
1–99
:
1 dr) and excellent enantioselectivities (95–99% ee) was reported by Ming Yan group in 2011.20 Presence of electron donating substituents were favorable both to the yield as well as to the diastereoselectivity of the reaction. Reaction failed when both the R1 and R2 group are isopropyl (Scheme 11).
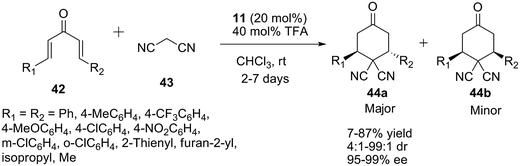 |
| Scheme 11 Cinchona catalysed asymmetric organocatalytic double-conjugate addition of malononitrile to dienones. | |
3.8. Double Michael addition reactions of isatylidene malononitriles with α,β-unsaturated ketones catalyzed by chiral cinchona
The asymmetric synthesis of spirocyclic oxindole derivatives bearing a cyclohexanone ring via double Michael addition reaction of isatylidene malononitriles 45 with α,β-unsaturated ketones 46 was reported by Xing-Wang Wang group21 in 2011. The combination of a cinchona-based chiral primary amine 47 and a BINOL-phosphoric acid in dichloroethane (DCE) allowed the formation of a novel chiral spiro[cyclohexane-1,30-indoline]-20,3-diones 48 bearing two stereogenic centers with one quaternary center in high yields (88–99%) with excellent diastereo- and enantioselectivities (94
:
6–99
:
1 dr, 95–99% ee) (Scheme 12).
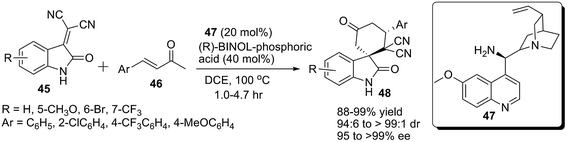 |
| Scheme 12 Double Michael addition of isatylidene malononitriles with α,β-unsaturated ketones. | |
3.9. Double Michael addition reactions of divinyl ketones with N-unprotected oxindoles or N-phenyl-protected pyrazolones catalyzed by chiral cinchona
Very recently after a gap of one year the same group in 2012 reported a nearly similar type of methodology for the synthesis of substituted spiro oxindole taking the advantage of another chiral organocatalyst with a scarce variation in the substrate scope.22 Reaction between divinyl ketones 49 and N-unprotected oxindoles 50 or N-phenyl-protected pyrazolones 51, catalyzed by combinations of quinine based catalysts 11 with additive N-Boc-D-OH provided spiro-[cyclohexanone-oxindoles] 52a in moderate to high yields (up to 98%) and with good to excellent diastereo and enantioselectivities (up to >20
:
1 dr, 99% ee). For N-unprotected oxindoles 50 presence of electron-donating substituents on the dienones resulted in products with higher yields and enantioselectivities, whereas electron-withdrawing substituents resulted in products with lower yields and enantioselectivities. When R1, R2 are phenyl or naphthyl yield of the product was very poor (Scheme 13).
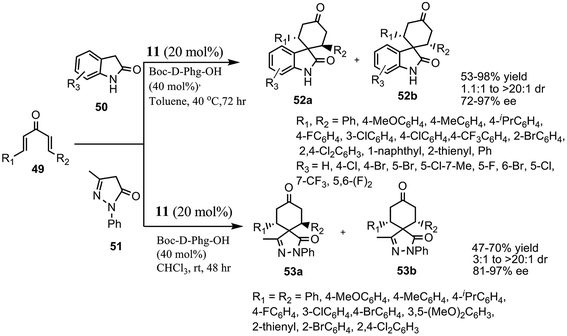 |
| Scheme 13 Enantioselective synthesis of spiro[cyclohexanone-oxindoles] and spiro[cyclohexanone-pyrazolones] by [5 + 1] double Michael reactions. | |
3.10. Double Michael addition reactions of nitroalkenoate with cinnamaldehyde catalyzed by chiral (S)-diphenylprolinol TMS ether catalyst
Filling a small gap in the literature, Bor-Cherng Hong group23 in 2013 again suggested a nearly similar type of methodology as proposed in 2008 for the synthesis of polysubstituted cyclohexane containing an ester group with a little variation in the substrate using the same catalyst 23.17 Double-Michael reaction of nitroalkenoate 54 and cinnamaldehyde 55 in the presence of the organocatalyst 23 afforded the highly functionalized tetra substituted cyclohexane derivative 56. The mild reaction conditions at ambient temperatures as well as the one-pot operation further evident the merit of this protocol (Scheme 14).
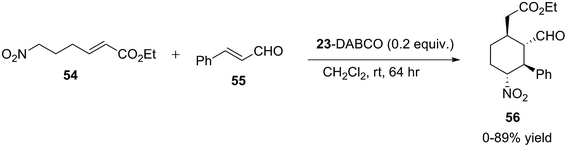 |
| Scheme 14 Double Michael addition reaction of nitroalkenoate and α,β-unsaturated aldehyde. | |
3.11. Double Michael addition reactions of fluorobis(phenylsulfonyl)methane (FBSM) with enals catalyzed by (S)-diphenylprolinol TMS ether catalyst
Taking the advantage of diphenyl prolinol TMS ether 23 as organocatalyst in 2014 Zdeněk Tošner group24 disclosed a new way to synthesize bicyclic compounds containing three contiguous stereocenters with excellent enantioselectivities. The cascade reaction consisted of a double Michael addition reaction via the addition of fluorobis(phenylsulfonyl)methane (FBSM) 58 to enals 57 resulting trans–trans substituted indanes 59 in good yields with excellent stereoselectivities (Scheme 15).
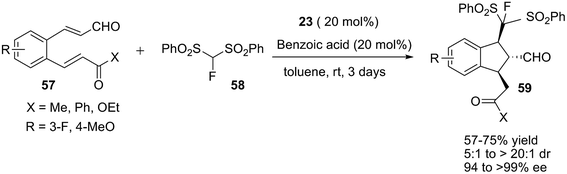 |
| Scheme 15 Double Michael addition of fluorobis(phenylsulfonyl)methane (FBSM) to enals. | |
3.12. Double Michael addition reactions of N-substituted isatylidene malononitriles with (E)-7-alkyl-7-oxohept-5-enalscatalyzed by (S)-diphenylprolinol TMS ether catalyst
Based on the precedent established previously for the synthesis of spiro oxindole by Xing-Wang-Wang group21 in 2011, recently the Zhao group25 in 2016 took the advantage of the reactivity of the same isatylidene malononitriles 61 moiety used by Xing-Wang-Wang group and reported the synthesis of spirooxindole 62 with a minute variation in the substrate scope and catalyst. By employing (S)-α,α-diphenylprolinol trimethyl silyl ether 23 as catalyst, the reaction between N-substituted isatylidene malononitriles 61 and (E)-7-alkyl-7-oxohept-5-enals 60 provided the desired spirooxindole products 62 in good yields (76–95%) and with excellent diastereoselectivities (up to 97
:
3 dr) and enantioselectivities (up to 98% ee) (Scheme 16).
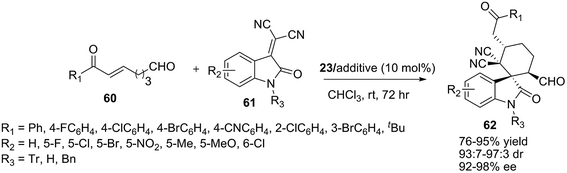 |
| Scheme 16 Stereoselective synthesis of spirooxindole derivatives using an organocatalysed tandem Michael–Michael reaction. | |
4. Base–base catalyzed double Michael addition
4.1. Double Michael addition reactions of β-amino alcohols with methylpropiolate catalyzed by diphenylphosphinopropane (DPPP) catalyst
Inspired by the previous success for the synthesis of heterocyclic compounds by using various organocatalyst through iminium ion activation, Ohyun Kwon et al. in 2007 reported the base mediated synthesis of heterocyclic compound with excellent yield.8a The bisphosphine 65 catalyzed mixed double-Michael addition reactions β-amino alcohols 63 and methylpropiolate 64 afforded the amino carbonyl derivatives of oxazolidines 66 in excellent yields with high diastereoselectivities. The mechanistic pathway for the formation of the product is shown in the scheme below (Scheme 17).
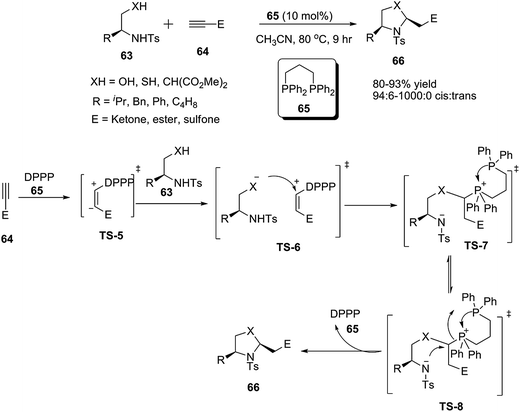 |
| Scheme 17 Bisphosphine-catalyzed mixed double-Michael reactions.![[thin space (1/6-em)]](https://www.rsc.org/images/entities/char_2009.gif) | |
4.2. Double Michael addition reactions of dienone with substituted barbituric acid catalyzed by triethanol amine catalyst
An intriguing methodology for the synthesis of spirocyclic compounds was reported by Jitender M. Khurana group8b in 2007. The authors elegantly disclosed a different approach for the synthesis of substituted spirobarbiturates 70 by the Michael–Michael addition of dienone 67 to substituted barbituric acid 68 by using simple available base triethanol amine 69 and solvent EtOH. Reductive desulfurization of 7,11-diaryl-3-thioxo-2,4-diazaspiro[5.5]undecane-1,5,9-triones 70 by nickel boride in dry methanol at ambient temperature resulted in the formation of a new spiroheterocycle 7,11-diaryl-2,4-diazaspiro[5.5]undecane-1,5,9-triones 71 (Scheme 18).
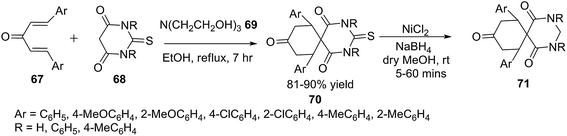 |
| Scheme 18 Double Michel addition reaction of dienone to substituted barbituric acid. | |
4.3. Double Michael addition reactions of N-Boc protected methylene indolinones to N-Ts protected trans-β-nitro styrene catalyzed by quinine derived tertiary amine catalyst
Filling a small gap in literature Chengjian Zhu group26 in 2013 reported a different approach for the enantioselective synthesis of spiro[tetrahydro-quinoline-3,30-oxindole] 75 skeleton from the readily available simple starting materials N-Boc protected methyleneindolinones 72. The aza-Michael–Michael reaction sequence of 72 and 73 catalysed by quinine based bifunctional catalyst 74 provided the desired product 75 in good yield (87–98%) with excellent stereoselectivity (>20
:
1 dr, 98% ee) (Scheme 19).
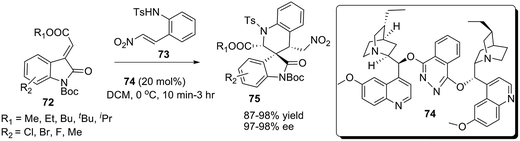 |
| Scheme 19 Asymmetric synthesis of spiro[chroman/tetrahydro-quinoline-3,30-oxindole] following double Michael addition reactions. | |
4.4. Double Michael addition reactions of carbon nucleophiles to 7 and 8 membered ring dienones catalyzed by DBU
Various strategies have also been reported for the synthesis of functionalized bicyclic compounds which have the advantage of containing multiple stereocenters. Jean Alexandre Richard group27 in 2013 reported the synthesis of 8-disubstituted bicyclo[3.2.1]-octane-3-ones 79 and 9-disubstituted bicyclo [3.3.1]octane-3-ones 79a through the double Michael addition (DMA) of carbon nucleophiles 77 to 7 and 8 membered ring dienones 76, catalysed by base DBU 78, with moderate to good yield (42–96%) with an interesting control of stereochemistry of the bridged secondary, tertiary and quaternary center (Scheme 20).
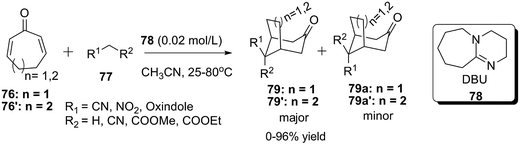 |
| Scheme 20 Expedient synthesis of bicyclo[3.2.1]octanes and bicyclo[3.3.1]nonanes via the double Michael addition to cyclic dienones. | |
4.5. Double Michael addition reactions of 2-cyano-2-cycloalkenones with the Nazarov reagent catalyzed by DBU
Another interesting report on the use of DBU as organocatalyst for the synthesis of substituted bicyclic was reported by Hsing-Jang Liu group28 in 2014. They reported a highly stereoselective annulations reaction of 2-cyano-2-cycloalkenones 80 with the Nazarov reagent 81 catalyzed by 1,8-diazabicyclo[5.4.0]undec-7-ene (DBU) 78, for the synthesis of a highly functionalized bicyclic system 82. This newly developed methodology has a broad synthetic utility in terms of its operational simplicity, good reaction yields and high diastereoselectivity (Scheme 21).
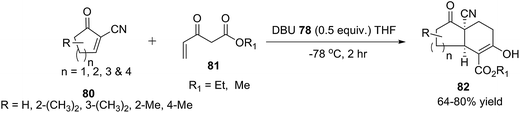 |
| Scheme 21 Double Michael addition reactions of Nazarov reagent with 2-cyano-2-cycloalkenones. | |
5. Acid–base mediated double Michael addition
5.1. Double Michael addition reactions of β-keto ester with β-nitrostyrene catalyzed by bifunctional thiourea catalyst
Taking the advantage of thiourea based bifunctional organocatalyst 85 Yoshiji Takemoto group9a in 2008 designed a Michael–Michael cascade reaction for the synthesis of highly functionalized cyclohexane 86. Asymmetric Michael addition of unsaturated β-keto ester 84 to the β-nitrostyrene 83 by bifunctional thiourea based catalyst 85 generated the intermediate (TS-9), which further undergoes intramolecular Michael addition to furnish the cyclic keto ester 86 in 77% yield and 75% ee. The asymmetric transformation was highly chemo and enantioselective, involving hydrogen bonding interaction by bifunctional thiourea to allow enantioselective Michael addition to form TS-9, followed by fast stereospecific second intramolecular Michael addition to close the ring. The natural product (−)-epibatidine 87 was synthesized from the intermediate (TS-10) in three steps (Scheme 22).
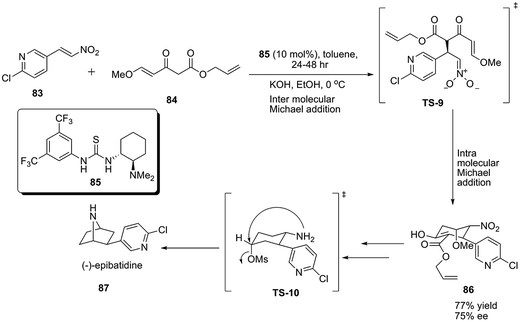 |
| Scheme 22 Bifunctional thiourea catalysed cascade Michael–Michael reaction. | |
5.2. Double Michael addition reactions of diethyl-5-acetylhex-2-enedioate with nitro alkene catalyzed by bifunctional cinchona catalyst
A scenario in which a suitable bifunctional acid–base organocatalyst 90 coordinates through H-bond interactions both electrophiles and nucleophile leading to a highly organized transition state has been designed by Guofu Zhong group in 2008.10 The authors developed a cinchona based bifunctional organocatalyst (9-amino-9-deoxyepiquinin 90) mediated double Michael addition reaction of diethyl-5-acetylhex-2-enedioate 88 with nitro alkene 89 to form highly functionalized cyclopentane 91, in which two C–C bonds and four contiguous stereogenic centers (containing one adjacent quaternary and tertiary stereocenters) were efficiently created in a one-pot operation with an efficient control of stereochemistry. The two substrate involved in the reaction were activated by catalyst 90 as shown in TS-11 (Scheme 23).
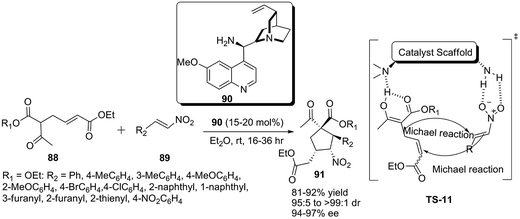 |
| Scheme 23 Double Michael addition reaction of diethyl 5-acetyl-hex-2-enedioate with nitroalkene. | |
5.3. Double Michael addition reactions of 3-(2-mercaptophenyl)-2-propenoic acid ethyl esters with α,β-unsaturated nitro compounds catalyzed by bifunctional cinchona alkaloid amine-thiourea catalyst
Based on their previous success in the field of enantioselective synthesis of polysubstituted carbocycle and thiacycle in 2007, Wei Wang group11 in 2008 elegantly extended the substrate scope to the benzofused heterocyclic system. They synthesized the trisubstituted benzothiochroman moiety 95 using cinchona alkaloid amine-thiourea 94 as catalyst at a low catalytic loading of 2 mol%. Reaction of 3-(2-mercaptophenyl)-2-propenoic acid ethyl esters 92 with α,β-unsaturated nitro compounds 93 in toluene afforded chiral thiochroman 95 bearing three continuous stereogenic centers with high diastereo- and enantioselectivities. The proposed mechanism of the reaction is shown in the TS-12 (Scheme 24).
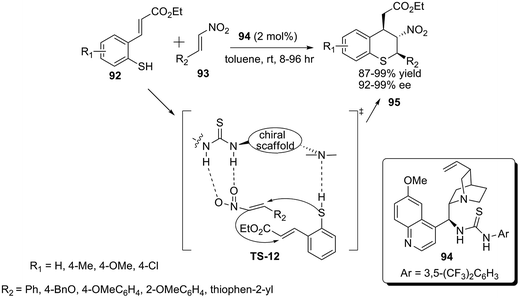 |
| Scheme 24 Cinchona alkaloid thiourea catalyzed Michael–Michael cascade reaction. | |
5.4. Double Michael addition reactions of thiols with nitro olefin enolates catalyzed by bifunctional thiourea catalyst
Inspired by the previous success in the field of thia Michael–Michael addition reaction by Wei Wang group11 in 2008, Jia-Rong Chen and Wen-Jing Xiao group29 in 2010 disclosed a new way for the synthesis of trisubstituted benzopyran moiety 99. They reported a bifunctional thiourea 98 catalysed sulfa-Michael/Michael addition reaction of thiols 96 with nitro olefin enolates 97 to afford polyfunctional chroman derivatives 99 in a highly stereoselective manner. The important features of this reaction is the generation of three consecutive stereogenic carbon centers including one quaternary stereo center with high enantioselectivity (up to 96% ee) and excellent diastereoselectivity (>95
:
5) (Scheme 25).
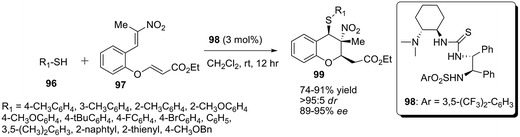 |
| Scheme 25 Organocatalytic asymmetric sulfa-Michael/Michael addition reaction between alkynals and o-hydroxy-β-nitrostyrenes. | |
5.5. Double Michael addition reactions of anilines to nitro olefin enoates catalyzed by bifunctional thiourea catalyst
After successful synthesis of benzofused heterocyclic system by thia-Michael–Michael addition reaction in 2010,29 Wen-Jing Xiao group30 in 2011 proposed a nearly similar type of methodology for the synthesis of poly substituted benzofused moiety through aza-Michael–Michael addition reaction with a little variation in the substrate scope and catalyst. The chiral bifunctional thiourea 102 catalyzed asymmetric aza-Michael–Michael addition reactions of anilines 100 to nitro olefin enoates 101 provided the polysubstituted chiral 4-aminobenzopyrans 103 bearing three consecutive stereocenters in high yields with excellent stereoselectivities. The mechanistic cycle for the formation of the product is shown in TS-13. The Z configuration of nitro olefinates 101 gave better yields of product in comparison to E configuration (Scheme 26).
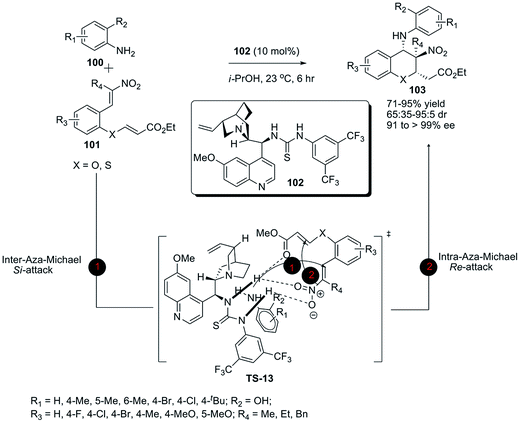 |
| Scheme 26 Catalytic asymmetric aza-Michael–Michael addition reaction of anilines to nitro olefin enoates. | |
5.6. Double Michael addition reactions of N-Boc protected oxindole with various nitro olefins catalyzed by bifunctional thiourea catalyst
An interesting methodology for the synthesis of spirocyclic compounds was reported in 2011 by Zhi-Hui Shao group,31 who used an enantioselective Ru-catalyzed olefin metathesis. As illustrated in the scheme, the reaction consists of a combined cross metathesis and asymmetric double Michael addition reaction to close the ring. The biologically important and synthetically challenging spirocyclopentaneoxindoles 110 with four contiguous stereocenters including one spiroquaternary stereocenter was obtained in good yields (72–87%) with excellent diastereoselectivity (16
:
1–30
:
1 dr) and enantioselectivity (93–99% ee) by the reaction of N-Boc protected oxindole 107 with various nitro olefins 108 using chiral bifunctional catalyst 109 in DCM (Scheme 27).
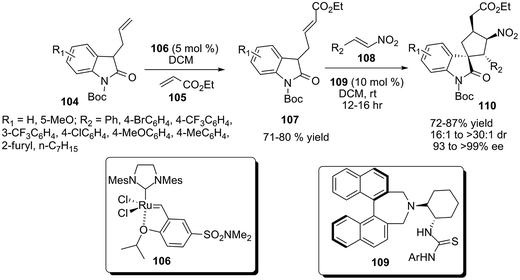 |
| Scheme 27 Synthesis of spirocyclopentaneoxindoles by double Michael addition reaction. | |
5.7. Double Michael addition reactions of malononitrile to 1,5-disubstituted pentadien-3-ones catalyzed by quinine
Using the same concept proposed by Ming Yan group20 in 2011, Alessandra Lattanzi group32 in same year successfully accomplished a similar reaction for the synthesis of poly substituted cyclohexanones from the same available starting material with a little variation in substituents and catalyst. The double Michael addition of malononitrile 43 to 1,5-disubstituted pentadien-3-ones 111 by using quinine catalyst 112 provided trans-4-oxo-2,6-diaryl-cyclohexane-1,1-dicarbonitriles 113a as the major isomer with excellent diastereo and enantioselectivity and cis-4-oxo-2,6-diaryl-cyclohexane-1,1-dicarbonitriles 113b as the minor isomer with very poor diastereoselectivities. The highest ee of trans isomer was observed when the R1, R2 = Ph i.e. symmetrically substituted. Poor yield of the product was obtained when R1 = 2-thienyl, R2 = Ph. No product formation was observed when dimethyl malonate or nitro methane was used instead of malononitrile 43 (Scheme 28).
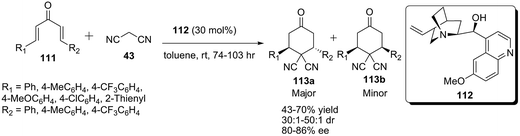 |
| Scheme 28 Quinine catalyzed double Michael addition of malononitrile to 1,5-disubstituted pentadien-3-ones. | |
5.8. Double Michael addition reactions of dienones with nitromethane catalyzed by bifunctional cinchona alkaloid thiourea catalyst
The cinchona alkaloid derived thiourea 94 and base (KOH) mediated enantioselective cascade Michael–Michael addition to generate highly functionalized 4-nitro cyclohexanone with two stereogenic centres was demonstrated by Xing Wang Wang and co-workers in 2011.33 Aromatic dienones and heteroaromatic dienones 114 were tested for the scope of the reaction with nitromethane 115 to afford the corresponding adducts 116 in good yield with high enantio (up to 97
:
3) and diastereoselectivities (>20
:
1). Increasing the amount of nitro methane 115 relative to that of dienone 114 the yield of 117 was considerably improved. The maximum yield, dr and ee of the product was obtained when both the R1 and R2 group were symmetrical (R1, R2 = Ph, 86% yield, >20
:
1 dr and 95
:
5 ee), whereas the unsymmetrical ketones provided the desired product with moderate diastereo and enantioselectivities (R1 ≠ R2, 84% yield, 1.25
:
1 dr and 82
:
18 ee). When the dienones containing alicyclic residues i.e. R1, R2 = cyclohexyl, no product formation was observed. Under solvent-free conditions, the yield was improved to 90%, but the enantioselectivity was reduced drastically (Scheme 29).
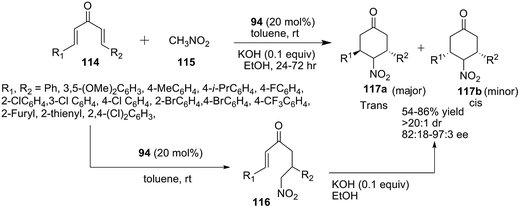 |
| Scheme 29 Cinchona-thiourea catalyzed double Michael addition of nitromethane to dienone. | |
5.9. Double Michael addition reactions of ortho-amino-substituted α,β-unsaturated ketones with nitroalkene catalyzed by bifunctional thiourea catalyst
Taking inspiration from Wen-Jing Xiao group30 in 2011, after one year Peng-Fei Xu group34 in 2012 reported a novel method for the synthesis of substituted tetra hydroquinolines 121 through a cascade aza-Michael–Michael reaction of ortho-amino-substituted α,β-unsaturated ketones 118 with nitroalkene 119 catalyzed by bifunctional thiourea derivative 120 in toluene at room temperature. The cascade process provides the tetrahydroquinoline derivatives with excellent yields (90–97%), good diastereoselectivity (up to 90
:
10 dr), and with high enantioselectivity (up to 99% ee). Electron donating groups in the substrate generally gave a slightly better yield of the product than that of electron withdrawing groups. The mechanistic cycle for the formation of the product is shown in TS-14 (Scheme 30).
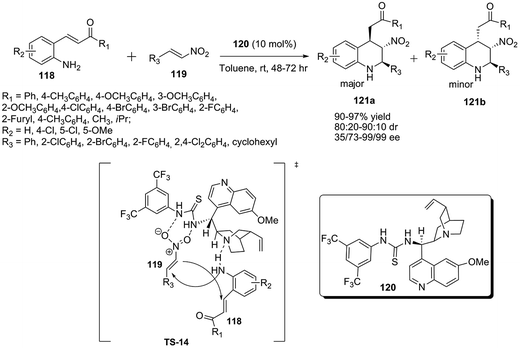 |
| Scheme 30 Organocatalytic aza-Michael–Michael cascade reactions of ortho-amino-substituted α,β-unsaturated ketones with nitroalkene. | |
5.10. Double Michael addition reactions of curcumins to nitro olefin catalyzed by bifunctional thiourea catalyst
With the intention of extending the catalyst and substrate scope, Namboothiri group in 2012 disclosed a new methodology for the synthesis of polysubstituted cyclohexanones 125 by the asymmetric double Michael addition of curcumins 122 to nitro olefin 123 using dihydrocinchonine-thiourea based organocatalyst 124.35 The catalyst 124 have dual mode of action (shown in TS-15), both the tertiary amine group and thiourea group activates the Michael donor and acceptor simultaneously by hydrogen bonding and provided the desired cyclohexanones 125 in good yield with high diastereo and enatioselectivity (Scheme 31).
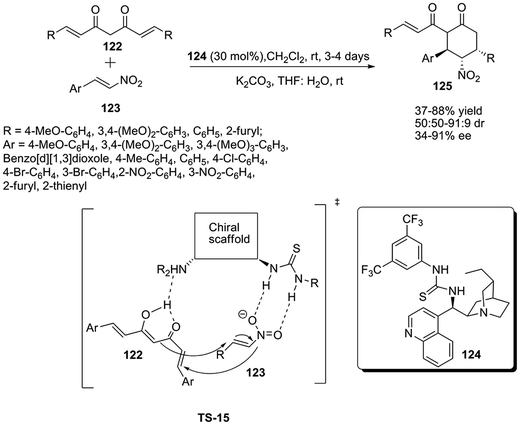 |
| Scheme 31 Double Michael addition of curcumins with nitroalkenes. | |
5.11. Double Michael addition reactions of nitrohex-4-enoates with nitro olefins catalyzed by bifunctional thiourea catalyst
Few year later J. A. Cobb group36 in 2012 presented a nearly similar type of methodology for the synthesis of polysubstituted cyclohexane as proposed by Bor-Cherng Hong group17 in 2008 with a minute discrepancy in the substrate scope and catalyst. In this report, the polysubstituted cyclohexane 127 was formed by an asymmetric domino Michael–Michael reaction between nitrohex-4-enoates 54 and nitro olefins 126, including one with a quaternary center, and three with five contiguous stereocenters catalysed by bifunctional catalyst 94 in DCM. Yield of the product was drastically reduces to 4% with 99% ee when R group was changed from H to methyl (Scheme 32).
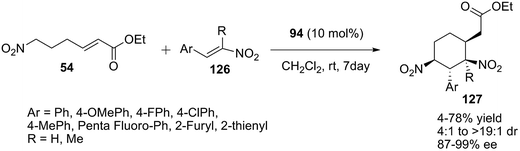 |
| Scheme 32 Double Michael addition of nitrohex-4-enoates and nitro-olefins. | |
5.12. Double Michael addition reactions of benzofuran-2-ones to dienone catalyzed by bifunctional thiourea catalyst
Xin Li group37 in 2013 reported a highly enantioselective synthesis of benzofuran-2-ones 130 by catalytic double-Michael addition reaction of substituted benzofuran-2-ones 128 to dienone 129 by using bifunctional thiourea catalyst 120 in p-xylene. The reaction provided a number of optically enriched spirocyclic benzofuran-2-ones 130 in good yields (up to 99%) with high diastereoselectivities (up to 19
:
1 dr) and very good enantioselectivities (up to 92% ee). The reactivity and selectivity of the reaction were more sensitive towards the substituents of 129. The dienones containing symmetrical m- and p-substituted phenyl groups gave maximum yield of the product where as lower yield and diastereoselectivities were obtained when o-aryl substituted divinyl ketones were selected as Michael acceptors. No product was obtained using aliphatic dienones (Scheme 33).
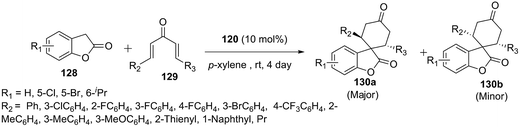 |
| Scheme 33 Bifunctional thiourea- base catalyzed double-Michael addition of benzofuran-2-ones to dienones. | |
5.13. Double Michael addition reactions of ε-nitro-α,β-unsaturated esters and cyanosulfones catalyzed by bifunctional thiourea catalyst
Very recently after a gap of one year the same group in 2013 reported again the synthesis of same polysubstituted cyclohexane 132 by using the same catalyst 94 and Michael acceptor group 54 and vary only in the Michael donor part.38 When ε-nitro-α,β-unsaturated esters 54 are added to conjugated cyanosulfones 131 in the presence of a bifunctional thiourea catalyst 94, a highly stereoselective domino reaction occurs to generate complex cyclohexanes 132 with up to four stereogenic centers, one of which is quaternary in nature. The mechanistic pathway for the formation of the product is shown in TS-16 (Scheme 34).
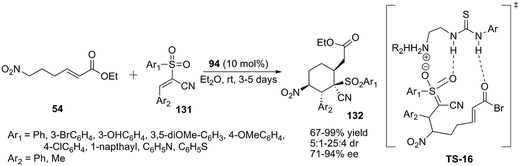 |
| Scheme 34 Double Michael addition of ε-nitro-α,β-unsaturated esters to conjugated cyanosulfones. | |
5.14. Double Michael addition reactions of trans-α-cyano-α,β-unsaturated ketones with trans-tert-butyl-4-mercapto-2-butenoate catalyzed by bifunctional thiourea catalyst
Alessandra Lattanzi et al.39 in 2013 reported the stereoselective synthesis of tetra substituted tetrahydrothiophenes 135 by the double Michael addition reaction of trans-α-cyano-α,β-unsaturated ketones 133 and trans-tert-butyl-4-mercapto-2-butenoate 26 in the presence of a readily available amine thiourea 134 as catalyst. The product 135 consists of three contiguous stereocenters and one of them is a quaternary and obtained in high yield, good diastereoselectivity, and excellent enantioselectivity. The overall stereoselectivity of tetrahydrothiophenes 135 occurs via dynamic kinetic resolution. α-Cyanoenones 133 bearing electron withdrawing substituents gave the maximum yield of the product up to 98% with excellent ee of 99%. Electron donating substituents at the β-position of enones 133 gave the lower yield of 70% but gave good ee (Scheme 35).
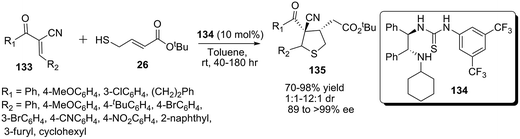 |
| Scheme 35 Double Michael addition reaction of α,β-unsaturated ketones with trans-tert-butyl-4-mercapto-2-butenoate. | |
5.15. Double Michael addition reactions of chalcones enolates with nitro methane catalyzed by bifunctional thiourea catalyst
Bifunctional thiourea 137 catalyzed enantioselective cascade Michael–Michael reaction between chalcones enolates 136 and nitro methane 115 afforded chiral benzopyrans 138 bearing three consecutive stereocenters in high yields (78–88%) with excellent enantioselectivities (97–>99%), was reported by Peng-Fei Xu et al.40 in 2013. The chiral benzopyran 138 then easily converted to corresponding tricyclic product 139 through reductive amination reaction with good yield and excellent enantioselectivity. Substrates with electron deficient substituent at R2 position had high reactivity as compared to electron rich substituent. It took less time for the reaction to complete with the electron-deficient substituent. No product formation was observed when the R2 = Me and R1 = H, which was probably due to its low reactivity (Scheme 36).
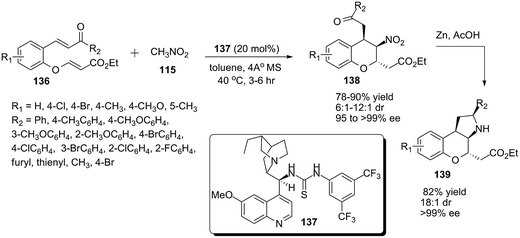 |
| Scheme 36 Organocatalyzed Michael–Michael cascade reaction between chalcones enolates and nitromethane. | |
5.16. Double Michael addition reactions of thiosalicylate to nitroalkene enolates catalyzed by bifunctionalsquaramide-tertiary amine catalyst
Shortly after the publication of Wen-Jing Xiao group30 in 2011, a very similar research work for the synthesis of poly substituted naphtha fused chromans was reported by Da-Ming Du group12 in 2013 with a little variation in the substrate and catalyst. They elegantly showed that they were able to catalyze the same type of reaction with only 1 mol% of a chiral bifunctional squaramide-tertiary amine 142 as catalyst. The asymmetric cascade sulfa-Michael/Michael addition reaction of thiosalicylate 140 to nitroalkene enolates 141 catalyzed by a chiral bifunctional squaramide-tertiary amine catalyst 142 provided an easy access to highly functionalized chromans 143 with three contiguous stereocenters, including one quaternary center with good yield, high diastereoselectivity (up to 95
:
5 dr) and outstanding enantioselectivities (95% ee). It was a novel cascade reaction pathway (sulfa-Michael/retro-sulfa-Michael/sulfa-Michael/Michael process), involving dynamic kinetic resolution (Scheme 37).
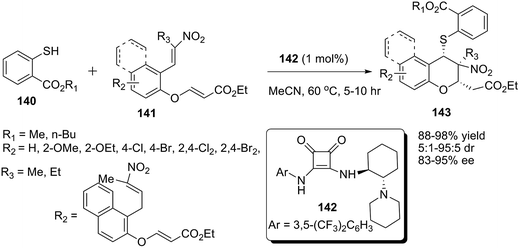 |
| Scheme 37 Double Michael addition reaction of thiosalicylate to nitroalkene enolates. | |
5.17. Double Michael addition reactions of 2-tosylaminochalcone with β-methyl-β-nitrostyrene or 3-nitro-2H-chromenes catalyzed by bifunctional squaramide-tertiary amine catalyst
Another design of cascade aza-Michael–Michael addition reaction procedure for the synthesis of tetrahydroquinolines 149 and tetrahydrochromanoquinolines 148 by the use of a bifunctional squaramide organocatalyst 146 was set up by Da-Ming Du group41 in 2013. At first they reported the synthesis of tetrahydroquinolines 149 using readily accessible 2-tosylaminochalcone 144 and β-methyl-β-nitrostyrene 145 as the model substrates.
After successful synthesis of tetrahydroquinolines 149 they extended the substrate scope to 3-nitro-2H-chromenes 145 for the synthesis of tetrahydrochromanoquinolines 148 by the use of 2-tosyl amino chalcone 144 in presence of the same squaramide catalyst 146. The proposed mechanistic pathway for the formation of the product is shown in TS-17 and TS-18 (Scheme 38).
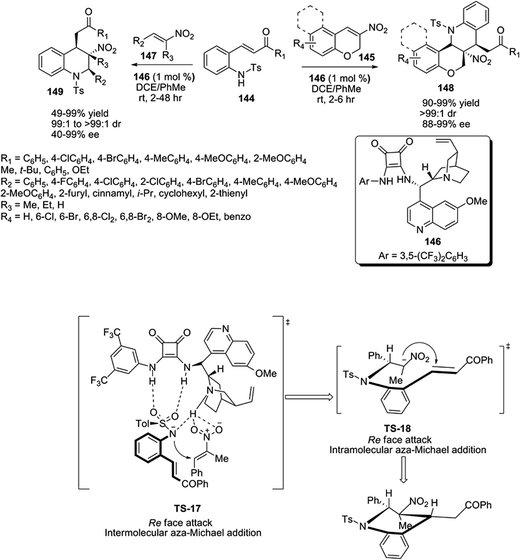 |
| Scheme 38 Organocatalytic enantioselective cascade aza-Michael/Michael addition reaction of 2-tosylaminochalcone with β-methyl-β-nitrostyrene and 3-nitro-2H-chromenes. | |
5.18. Double Michael addition reactions of N-Boc protected methylene indolinones with 2-(E)-(2-nitrovinyl) phenol catalyzed by bifunctional squaramide-tertiary amine catalyst
The group of Chengjian Zhu26 in 2013 developed a range of chiral cinchona catalyzed Michael–Michael conjugate addition reactions of N-Boc protected methyleneindolinones 72. The double Michael addition reaction of N-Boc protected methyleneindolinones 72 with N-Ts-2-(E)-(2-nitrovinyl) aniline 73 was successfully catalyzed with cinchona catalyst 74 providing spiro [tetrahydro-quinoline-3,30-oxindole] 75 with good yield and excellent enantioselectivities (Scheme 19). A related process utilizing the same N-Boc protected methyleneindolinones 72 as Michael acceptor with a little variation in the Michael donor i.e. 2-(E)-(2-nitrovinyl) phenol 150 was reported by the same author. Here a squarimide catalyst 151 with a different chiral backbone was employed to deliver the products with good stereoselectivity. The oxa-Michael–Michael reaction of 72 and 2-(E)-(2-nitrovinyl)phenol 150 derivatives catalyzed by a squaramide-tertiary amine 151 proceeded well under mild conditions to furnish a series of spiro[chroman-3,30-oxindole] 152 derivatives containing three contiguous stereocenters, including one spiro quaternary chiral center with maximum up to 71% yield, 85% ee and 10
:
1 dr (Scheme 39).
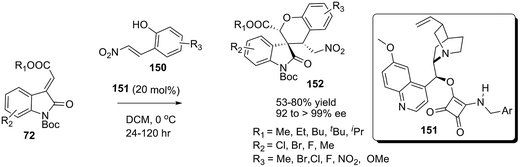 |
| Scheme 39 Asymmetric synthesis of spiro [chroman/tetrahydro-quinoline-3, 30-oxindole] following double Michael addition reactions. | |
5.19. Double Michael addition reactions of indolin-3-one derivatives with nitro alkene catalyzed by bifunctional thiourea catalyst
Thereafter Da-Ming Du's work in 2013,41 another paper on the synthesis of bicyclic compound appeared in the literature by Peng-Fei Xu et al.42 in 2014. Here the authors reported the construction of highly functionalized N-fused piperidinoindoline derivatives 156 by employing much less explored indolin-3-one derivatives 153 via a bifunctional thiourea-catalyzed asymmetric Michael–Michael cascade reaction. The authors reported the synthesis of structurally interesting polysubstituted piperidino[1,2-a]-indoline compounds 156 containing four contiguous stereocenters including one tetra substituted carbon center with good yields (up to 94% yield) and excellent enantioselectivities (up to >99% ee) by employing indolin-3-one derivatives 153 and nitro alkene 154 as substrates via a bifunctional catalysis 155 in DCM. The temperature had a marked effect on the diastereoselectivities of the reaction, by lowering the temperature the yield and diastereoselectivities of the reaction increase significantly. Reaction failed when nitro alkene was containing 2-furyl group. The tentative mechanism for the formation of the product is shown in TS-19 (Scheme 40).
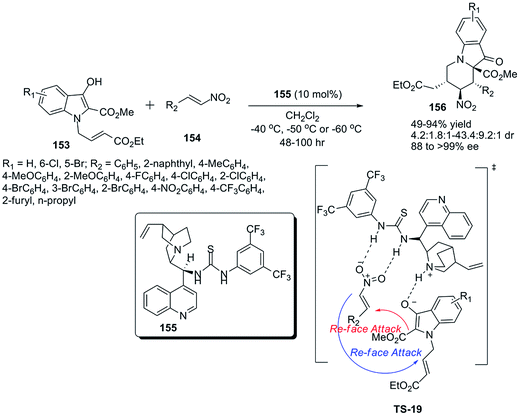 |
| Scheme 40 Organocatalytic asymmetric Michael–Michael cascade for the construction of highly functionalized N-fused piperidinoindoline derivatives. | |
5.20. Double Michael addition reactions of nitromethane with enones catalyzed by bifunctional thiourea catalyst
Inspired by their previous success in the synthesis of poly substituted carbocycles and spirocycles, Xing-Wang Wang group43 in 2014 developed an interesting methodology for the synthesis of bicyclic compound containing multiple stereocenters. The authors reported a cascade regio- and enantioselective double Michael addition to construct challenging multifunctionalized chiral indane 160 derivatives in the presence of a bifunctional acid–base organocatalyst 158 and 159. They envisaged that simultaneous double Michael addition of nitromethane 115 to both the enones of 157 in one pot through activation of an appropriate enantiopure bifunctional acid–base organocatalyst 158 and 159 to generate multifunctionlised indane derivative 160. The resulting optically active indane derivatives 160 with three alternating trans-stereocenters were produced in moderate to good yields with excellent diastereoselectivities and excellent enantioselectivities. No product formation was observed when R1, R2 = Me (Scheme 41).
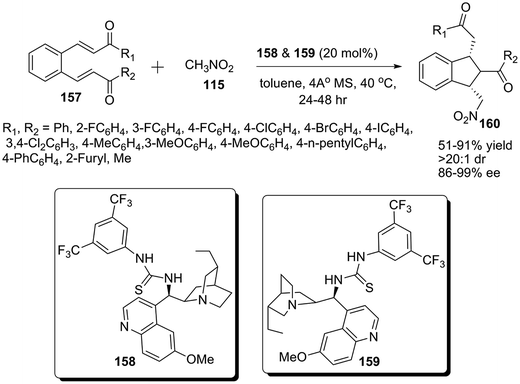 |
| Scheme 41 Double Michael addition reaction of nitromethane to enones. | |
5.21. Double Michael addition reactions of 2-tosylaminoenones with unsaturated pyrazolones catalyzed by bifunctional squaramide-tertiary amine catalyst
Da-Ming Du group44 in 2014 reported the synthesis of novel chiral spiropyrazolone tetrahydroquinolines 164 containing three continuous stereogenic centers by an organocatalyzed diastereo- and enantioselective cascade aza-Michael/Michael addition of 2-tosylaminoenones 162 to unsaturated pyrazolones 161. This cascade reaction proceeded well with 2 mol% chiral bifunctional tertiary amine squaramide catalyst 163 to give the desired products in excellent yields (up to 99%) with excellent diastereoselectivity (up to >25
:
1 dr) and high enantioselectivity (up to 91% ee). The proposed mechanism for the formation of the product is shown in TS-20 (Scheme 42).
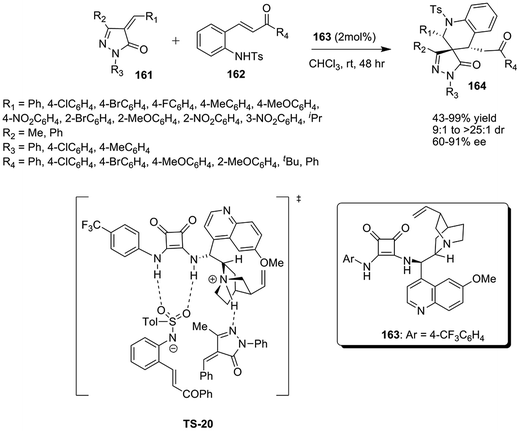 |
| Scheme 42 Organo catalysed cascade aza-Michael/Michael addition reaction of 2-tosylaminoenones to unsaturated pyrazolones. | |
5.22. Double Michael addition reactions of methylene indolinone with 3-substituted oxindole catalyzed by bifunctional squaramide-tertiary amine catalyst
One of the most elegant enantioselective methodologies for the synthesis of spiro compounds was proposed by Rui Wang group45 in 2014 after getting success in the synthesis of spiropyrazolones in 2013.6 The authors reported an organocatalytic Michael–Michael cascade for the enantioselective construction of spirocyclopentane bioxindoles 168 by the reaction of methyleneindolinone 165 and 3-substituted oxindole 166 in moderate to good yield with good diastereoselectivities and excellent enantioselectivities catalysed by 167 (Scheme 43).
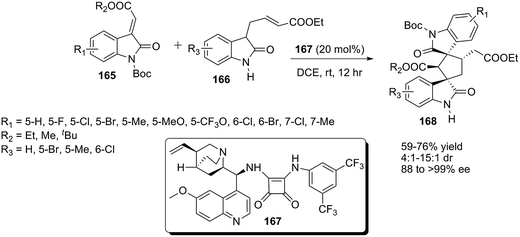 |
| Scheme 43 Double Michael addition reaction between the methyleneindolinone and 3-substituted oxindole. | |
5.23. Double Michael addition reactions of aculeatin A
A new organocatalyst free synthetic strategy for the total synthesis of aculeatin A 171 was reported by Rongbiao Tong group46 in 2014. The authors reported the concise total synthesis of aculeatin A 171 as a single spiro isomer in both racemic and enantioselective fashions in 8–10 steps with ∼10% overall yield from the known alkyne 169, featuring phenol oxidative dearomatization, double intramolecular oxa-Michael addition of secondary/tertiary alcohols, and chemo- and stereoselective reduction of ketone (Scheme 44).
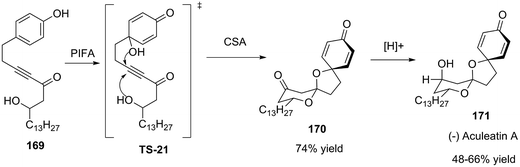 |
| Scheme 44 Total synthesis of aculeatin A via double intramolecular oxa-Michael–Michael addition of secondary/tertiary alcohols. | |
5.24. Double Michael addition reactions of 1,5-diaryl-1,4-pentadien-3-one with substituted barbituric acid catalyzed by ethylene glycol
Filling a remarkable gap in the literature after seven years in 2014, Jitender M. Khurana group again reported another closely related example for the synthesis of spirobarbiturates without the use of any organocatalyst.47 In this report, the authors presented the synthesis of 2,4-diazaspiro[5.5]undecane-1,3,5,9-tetraones and 3-thioxo-2,4-diazaspiro[5.5]undecane-1,5,9-triones 174 via double Michael addition of 1,5-diaryl-1,4-pentadien-3-one 172 with active methylene compounds such as N,N-dimethyl barbituric acid, barbituric acid, thio-barbituric acid and N,N-diphenyl thio-barbituric acid 173 in ethylene glycol at 100 °C in the absence of any catalyst to give high yields within a short reaction time. The reaction proceeds faster in thio-barbituric acid as compared to N,N-diphenyl thio-barbituric acid (Scheme 45).
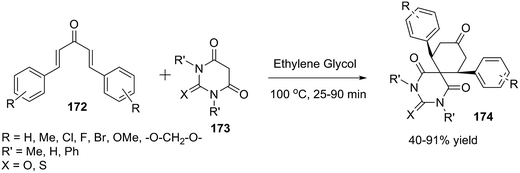 |
| Scheme 45 Double Michael addition reaction between 1,5-diaryl-1,4-pentadien-3-one with active methylene compounds. | |
6. Conclusions
In summary, this review summarises the recent developments in the field of organocatalysed Michael–Michael cascade reaction for the formation of highly functionalised cyclic molecules bearing multiple stereocenters in a stereoselective manner. A great variety of organic substrates, and diverse range of organocatalysts have found application in constructing five and six membered highly functionalised ring via organocatalytic double Michael addition reaction. Current limitations concern the relatively high catalyst loading, and the long reaction time of the organocatalyst, which will require the design and employment of more active organocatalysts to promote the process. It is expected that using suitable substrate, higher membered cyclic ring with multiple stereocenters can be synthesized following these processes to increase the molecular diversity in organic transformations.
References
-
(a) J. Franzén, M. Marigo, D. Fielenbach, T. C. Wabnitz, A. Kjærsgaard and K. A. Jørgensen, J. Am. Chem. Soc., 2005, 127, 18296–18304 CrossRef PubMed;
(b) D. Enders, M. R. M. Huttl, C. Grondal and G. Raabe, Nature, 2006, 441, 861–863 CrossRef CAS PubMed;
(c) Y. Deng, S. Kumar and H. Wang, Chem. Commun., 2014, 50, 4272–4284 RSC.
-
(a) S. Nayak, S. Chakroborty, S. Bhakta, P. Panda and S. Mohapatra, Res. Chem. Intermed., 2015, 41, 4545–4553 CrossRef;
(b) Y. Zhang and W. Wang, Catal. Sci. Technol., 2012, 2, 42–53 RSC;
(c) C. Grondal, M. Jeanty and D. Enders, Nat. Chem., 2010, 2, 167–178 CrossRef CAS PubMed;
(d) D. W. C. MacMillan, Nature, 2008, 455, 304–308 CrossRef CAS PubMed.
-
(a) C. Bhanja, S. Jena, S. Nayak and S. Mohapatra, Beilstein J. Org. Chem., 2012, 8, 1668–1694 CrossRef CAS PubMed;
(b) S. Nayak, S. Chakroborty, S. Bhakta, P. Panda, S. Mohapatra, S. Kumar, P. K. Jena and C. Purohit, Lett. Org. Chem., 2015, 12, 352–358 CrossRef CAS;
(c) M. Ihara and K. Fukumoto, Angew. Chem., Int. Ed. Engl., 1993, 32, 1010–1022 CrossRef.
- P. Chauhan, S. Mahajan, U. Kaya, D. Hack and D. Enders, Adv. Synth. Catal., 2015, 357, 253–281 CrossRef CAS.
- L. Y. Wu, G. Bencivenni, M. Mancinelli, A. Mazzanti, G. Bartoli and P. Melchiorre, Angew. Chem., Int. Ed., 2009, 48, 7196–7199 CrossRef CAS PubMed.
- J. Liang, Q. Chen, L. Liu, X. Jiang and R. Wang, Org. Biomol. Chem., 2013, 11, 1441–1445 CAS.
- Q. An, J. Shen, N. Butt, D. Liu, Y. Liu and W. Zhang, Synthesis, 2013, 45, 1612–1623 CrossRef CAS.
-
(a) V. Sriramurthy, G. A. Barcan and O. Kwon, J. Am. Chem. Soc., 2007, 129, 12928–12929 CrossRef CAS PubMed;
(b) J. M. Khurana, R. Arora and S. Satija, Heterocycles, 2007, 71, 2709–2716 CrossRef CAS.
-
(a) H. Miyabe and Y. Takemoto, Bull. Chem. Soc. Jpn., 2008, 81, 785–795 CrossRef CAS;
(b) T. Akiyama, J. Itoh and K. Fuchibea, Adv. Synth. Catal., 2006, 348, 999–1010 CrossRef CAS.
- B. Tan, Z. Shi, P. J. Chua and G. Zhong, Org. Lett., 2008, 10, 3425–3428 CrossRef CAS PubMed.
- J. Wang, H. Xie, H. Li, L. Zu and W. Wang, Angew. Chem., Int. Ed., 2008, 47, 4177–4179 CrossRef CAS PubMed.
- W. Yang, Y. Yang and D. M. Du, Org. Lett., 2013, 15, 1190–1193 CrossRef CAS PubMed.
- J. H. Li and D. M. Du, Org. Biomol. Chem., 2015, 13, 9600–9609 CAS.
- L. Zu, H. Li, H. Xie, J. Wang, W. Jiang, Y. Tang and W. Wang, Angew. Chem., 2007, 119, 3806–3808 CrossRef.
- H. Li, L. Zu, H. Xie, J. Wang, W. Jiang and W. Wang, Org. Lett., 2007, 9, 1833–1835 CrossRef CAS PubMed.
- H. Li, L. Zu, H. Xie, J. Wang and W. Wang, Chem. Commun., 2008, 5636–5638 RSC.
- B. C. Hong, R. Y. Nimje, M. F. Wu and A. A. Sadani, Eur. J. Org. Chem., 2008, 1449–1457 CrossRef.
- A. Ma and D. Ma, Org. Lett., 2010, 12, 3634–3637 CrossRef CAS PubMed.
- X. Zhang, S. Zhang and W. Wang, Angew. Chem., Int. Ed., 2010, 49, 1481–1484 CrossRef CAS PubMed.
- X. Li, B. Wang, J. Zhang and M. Yan, Org. Lett., 2011, 13, 374–377 CrossRef CAS PubMed.
- Y. B. Lan, H. Zhao, Z. M. Liu, G. G. Liu, J. C. Tao and X. W. Wang, Org. Lett., 2011, 13, 4866–4869 CrossRef CAS PubMed.
- B. Wu, J. Chen, M. Q. Li, J. X. Zhang, X. P. Xu, S. J. Ji and X. W. Wang, Eur. J. Org. Chem., 2012, 1318–1327 CrossRef CAS.
- B. C. Hong, W. K. Liao, N. S. Dange and J. H. Liao, Org. Lett., 2013, 15, 468–471 CrossRef CAS PubMed.
- Y. S. Kim, S. M. Kim, B. Wang, X. Company, J. Li, A. Moyano, S. Im, Z. Tošner, J. W. Yang and R. Riosa, Adv. Synth. Catal., 2014, 356, 437–446 CrossRef CAS.
- H. Huang, M. Bihani and J. C. Zhao, Org. Biomol. Chem., 2016, 14, 1755–1763 CAS.
- H. Mao, A. Lin, Y. Tang, Y. Shi, H. Hu, Y. Cheng and C. Zhu, Org. Lett., 2013, 15, 4062–4065 CrossRef CAS PubMed.
- A. Moulia, J. Teo, C. W. Johannes and J. A. Richard, RSC Adv., 2013, 3, 22882–22886 RSC.
- C. H. Tu, Y. K. Wu, K. S. Shia, S. C. Kuo, H. J. Liu and M. T. Hsieh, Synlett, 2014, 25, 543–546 CAS.
- X. F. Wang, Q. L. Hua, Y. Cheng, X. L. An, Q. Q. Yang, J. R. Chen and W. J. Xiao, Angew. Chem., Int. Ed., 2010, 49, 8379–8383 CrossRef CAS PubMed.
- X. F. Wang, J. An, X. X. Zhang, F. Tan, J. R. Chen and W. J. Xiao, Org. Lett., 2011, 13, 808–811 CrossRef CAS PubMed.
- Y.-M. Li, X. Li, F.-Z. Peng, Z.-Q. Li, S.-T. Wu, Z.-W. Sun, H.-B. Zhang and Z.-H. Shao, Org. Lett., 2011, 13, 6200–6203 CrossRef CAS PubMed.
- C. D. Fuscoand and A. Lattanzi, Eur. J. Org. Chem., 2011, 3728–3731 Search PubMed.
- B. Wu, G. G. Liu, M. Q. Li, Y. Zhang, S. Y. Zhang, J. R. Qiu, X. P. Xu, S. J. Ji and X. W. Wang, Chem. Commun., 2011, 47, 3992–3994 RSC.
- Z. X. Jia, Y. C. Luo, Y. Wang, L. Chen, P. F. Xu and B. Wang, Chem.–Eur. J., 2012, 18, 12958–12961 CrossRef CAS PubMed.
- N. Ayyagari and I. N. N. Namboothiri, Tetrahedron, 2012, 23, 605–610 CrossRef CAS.
- S. Rajkumar, K. Shankland, G. D. Brownb and A. J. A. Cobb, Chem. Sci., 2012, 3, 584–588 RSC.
- X. Li, C. Yang, J. L. Jin, X. S. Xue and J. P. Cheng, Chem.–Asian J., 2013, 8, 997–1003 CrossRef CAS PubMed.
- S. Rajkumar, K. Shankland, J. M. Goodman and A. J. A. Cobb, Org. Lett., 2013, 15, 1386–1389 CrossRef CAS PubMed.
- S. Meninno, G. Croce and A. Lattanzi, Org. Lett., 2013, 15, 3436–3439 CrossRef CAS PubMed.
- Z.-X. Jia, Y.-C. Luo, X.-N. Cheng, P.-F. Xu and Y.-C. Gu, J. Org. Chem., 2013, 78, 6488–6494 CrossRef CAS PubMed.
- W. Yang, H. X. He, Y. Gao and D. M. Dua, Adv. Synth. Catal., 2013, 355, 3670–3678 CrossRef CAS.
- Y.-L. Zhao, Y. Wang, J. Cao, Y.-M. Liang and P.-F. Xu, Org. Lett., 2014, 16, 2438–2441 CrossRef CAS PubMed.
- N. Li, G. G. Liu, J. Chen, F. F. Pan, B. Wu and X. W. Wang, Eur. J. Org. Chem., 2014, 2677–2681 CrossRef CAS.
- J. H. Li and D. M. Du, Chem.–Asian J., 2014, 9, 3278–3286 CrossRef CAS PubMed.
-
(a) W. Sun, L. Hong, G. Zhu, Z. Wang, X. Wei, J. Ni and R. Wang, Org. Lett., 2014, 16, 544–547 CrossRef CAS PubMed;
(b) D. Cheng, Y. Ishihara, B. Tan and C. F. Barbas, ACS Catal., 2014, 4, 743–762 CrossRef CAS;
(c) P. Chauhan, S. Mahajan and D. Enders, Chem. Commun., 2015, 51, 12890–12907 RSC.
- H. Yao, L. Song and R. Tong, J. Org. Chem., 2014, 79, 1498–1504 CrossRef CAS PubMed.
- K. Aggarwal, K. Vij and J. M. Khurana, RSC Adv., 2014, 4, 13313–13321 RSC.
|
This journal is © The Royal Society of Chemistry 2016 |
Click here to see how this site uses Cookies. View our privacy policy here.