DOI:
10.1039/C5SC03084F
(Edge Article)
Chem. Sci., 2016,
7, 1033-1037
Carborane-based design of a potent vitamin D receptor agonist†‡
Received
19th August 2015
, Accepted 26th October 2015
First published on 27th October 2015
Abstract
The vitamin D nuclear receptor (VDR) is a potential target for cancer therapy. It is expressed in many tumors and its ligand shows anticancer actions. To combine these properties with the application of boron neutron capture therapy (BNCT), we design and synthesize a potent VDR agonist based on the skeleton of the hormone 1α,25-dihydroxyvitamin D3 (1,25D) and an o-carborane (dicarba-o-closo-1,2-dodecaborane) at the end of its side chain. The present ligand is the first secosteroidal analog with the carborane unit that efficiently binds to VDR and functions as an agonist with 1,25D-like potency in transcriptional assay on vitamin D target genes. Moreover it exhibits similar antiproliferative and pro-differentiating activities but is significantly less hypercalcemic than 1,25D. The crystal structure of its complex with VDR ligand binding domain reveals its binding mechanism involving boron-mediated dihydrogen bonds that mimic vitamin D hydroxyl interactions. In addition to the therapeutic interest, this study establishes the basis for the design of new unconventional vitamin D analogs containing carborane moieties for specific molecular recognition, and drug research and development.
Introduction
Icosahedral carboranes (dicarba-closo-dodecaboranes) are carbon–boron clusters with spherical geometry and a hydrophobic surface. They are thermally and metabolically stable, with high lipophilicity and ability to form an unusual proton–proton (dihydrogen) bond B–H⋯H–X (X = N, O, C).1,2 Carboranes have been used as unique pharmacophores in biologically active compounds.3,4 Initial development of the medicinal chemistry application of boron was applied to boron neutron capture therapy (BNCT) of cancer with the objective to selectively destroy cancer cells as an alternative to conventional radiation therapy. BNCT is based on the nuclear capture of neutrons by 10B to produce high energy particles that selectively destroy tumors without affecting healthy tissue.5–8 The development of this technique depends on the availability of boron compounds which bind receptors in the tumors. To target tumors more specifically, derivatives of natural ligands that target receptors but also bear boron atoms have been synthesized.4,9,10 More recently, boron compounds have been shown to have broader applications in medicinal chemistry as active biomolecules such as carborane compounds that have anticancer activity or act as HIV replication inhibitors.3,4
Among the receptors expressed in tumors that can be targeted by carborane ligands for cancer therapy, the vitamin D receptor (VDR), a member of the nuclear receptor superfamily, is a potential candidate.11 Vitamin D3, before eliciting its physiological functions, undergoes two hydroxylations, first in the liver and then in the kidney, to produce its active form, 1α,25-dihydroxyvitamin D3 (1,25D, Fig. 1). The hormone 1,25D through binding to VDR regulates a number of physiological and pathological processes, including cell proliferation and differentiation, which has led to the study of its effects in cancer. Significant antitumor activity (i.e. anti-proliferative effects, induction of apoptosis, stimulation of differentiation, and inhibition of invasion and metastasis) has been demonstrated for 1,25D in different types of cancers including breast, prostate, colon, and acute myeloid leukemia.12,13 In fact, recent studies have established that VDR expression should be taken into account for breast cancer treatment.14
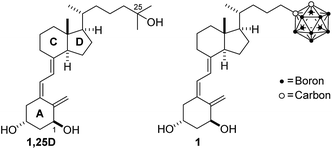 |
| Fig. 1 Vitamin D hormone (1,25D) and the target carborane 1. | |
Results and discussion
In this study, we describe the design, the synthesis and the functional and structural characterization of a novel vitamin D analog bearing an o-carborane group at the side chain (1, Scheme 1). To date, only a few simple carborane mimics of vitamin D have been developed. These compounds, which show reduced activity, lack the 1,25D A-ring moiety and possess a p-carborane unit that replaces the natural CD-rings.15–18 The p-carborane unit of theses vitamin D mimics interacts with the VDR only through van der Waals interactions.15 More recently19 the same authors synthesized structure-related compounds which exhibit similar pro-differentiation activities in HL-60 cells as the natural 1,25D hormone.
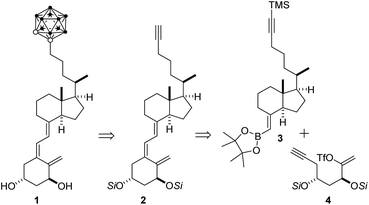 |
| Scheme 1 Retrosynthesis of target carborane 1. Si = protecting group, TMS = SiMe3. | |
Carborane 1 is the first secosteroidal vitamin D analog with the carborane unit that efficiently binds to the VDR ligand binding domain (LBD) and is as transcriptionally active as 1,25D on vitamin D target genes, but is significantly less hypercalcemic than 1,25D. In addition to the therapeutic interest, our study helps clarify the functional behavior of this molecule.
Design
On the basis of the crystal structure of an engineered active ligand-binding domain of the human vitamin D receptor (hVDR LBD) bound to 1,25D,20 which reveals an additional hydrophobic space around the side chain, and inspired by the biological profile of carboranes,3,4 we designed a new analog bearing an o-carborane moiety at the end of the side chain (1, Fig. 1). Docking results show that the novel vitamin D analog fits reasonably well in the ligand binding pocket. The ligand adopts the canonical active conformation in which the rings and the triene system of the new ligand have similar positions as the natural hormone 1,25D. The A-ring hydroxyl groups form hydrogen bonds with the same amino acid residues as 1,25D. Remarkably, the side-chain carborane moiety binds by unusual dihydrogen bonding with the His305 and His397 residues (Fig. 2).
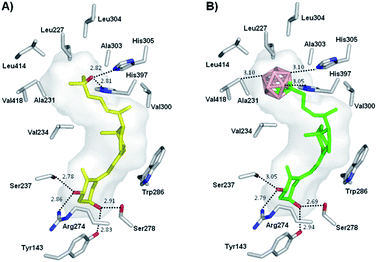 |
| Fig. 2 (A) Structure of 1,25D (yellow) in complex with hVDR LBD (PDB ID: 1DB1). (B) Docked structure of analog 1 (green) into hVDR LBD. Distances are shown in Å. | |
Synthesis
Our plan for the synthesis of the target analog 1 entailed formation of the o-carborane unit late in the synthesis to circumvent anticipated problems related to carborane chemistry and characterization. Construction of the carborane-containing side was envisaged to arise by reaction between alkyne 2 and decaborane (B10H14).4 It was not clear at this point if the labile vitamin D triene system would withstand the reaction conditions. The vitamin D triene system would arise by a Pd-catalyzed cascade process21 involving ring-closure of enol-triflate 4 and coupling with boronate 3 (Scheme 1).
The synthesis of carborane 1 is illustrated in Scheme 2 and started with known Inhoffen-Lythgoe diol (5a),22 which was converted to known tosylate 5b. Exposure of tosylate 5b to magnesium reagent 6
23 in the presence of catalytic Li2CuCl4 provided alcohol 7a (96%), which was oxidized with pyridinium dichromate to the corresponding ketone 7b (98%). Wittig chemistry on 7b using ylide Ph3P
CHBr (prepared by treatment of Ph3PCH2Br2 with KOtBu)21,24 provided vinyl bromide 8 (77%), which was converted to boronate 3 (88%) by metalation with tBuLi, reaction of the organolithium with triisopropyl boronate, and transesterification with pinacol. The triene system of 2 was constructed in 90% yield by Pd-catalyzed assembling21 of boronated 3 with the Pd-intermediate resulting from ring-closure of enol-triflate 4
25 in the presence of PdCl2(PPh3)2 and K3PO4, followed by desilylation of the side chain (HF). In the crucial step, the carborane unit of 1 was introduced in the side chain in 43% yield by reaction of alkyne 2 with decaborane in the presence of N,N-dimethylaniline. Finally, deprotection of 10 furnished the desired carborane analog 1 in 93% yield (19% overall yield, 9 steps).
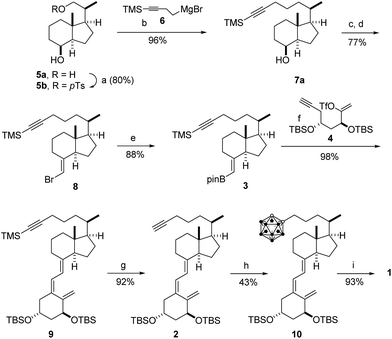 |
| Scheme 2 Synthesis of target analog 1. Reagents and conditions: (a) pTsCl (1.5 equiv.), py, 0 °C, 12 h; (b) 6 (4 equiv.), Et2O/THF (1 : 1); Li2CuCl4 (0.01 equiv.), THF, −78 °C → 23 °C, 12 h; (c) oxidation of 7a to the corresponding ketone 7b: PDC (3 equiv.), CH2Cl2, 23 °C, 5 h; (d) (Ph3PCH2Br)Br (8 equiv.), tol, ultrasound, 15 min; KOtBu in THF (7.9 equiv., 1 M), −15 °C → 0 °C, 2.5 h, then 7b in tol, −15 °C → 23 °C, 3 h; (e) tol/THF (3 : 1), −78 °C, tBuLi (2 equiv.), 1 h; B(OiPr)3 (1.5 equiv.), 1.5 h; pinacol (1.3 equiv.), −78 °C → 23 °C, 4 h; (f) 4 (1 equiv.), K3PO4 (27 equiv., aqueous sol, 2 M), THF, PdCl2(PPh3)2 (0.05 equiv.), 2 h; (g) K2CO3, MeOH, 23 °C, 14 h; (h) PhNMe2 (6.5 equiv.), tol, decaborane (B10H14, 3 equiv.), 110 °C, 1 h; (i) HF (48%, 2.5 equiv.), CH2Cl2/CH3CN (2 : 1), 23 °C, 12 h. pTsCl = p-toluenesulfonyl chloride, PDC = pyridinium dichromate, py = pyridine, tol = toluene, TBS = SitBuMe2. | |
Functional activity
We next analyzed the biological properties of carborane 1 in various cell lines. The biological transcriptional activity of 1, as compared to 1,25D, was evaluated in human MCF-7 breast cancer cells. Both compounds at 10−8 and 10−7 M concentrations significantly decreased cell proliferation with respect to control cells (Fig. 3a) although they are 3-fold less effective than calcipotriol.26 Carborane 1 also showed similar differentiation-inducing activity compared to 1,25D in HaCaT keratinocytes (Fig. 3b). Competitive binding assay demonstrated that VDR binding affinity of 1 was two times higher than that of 1,25D (IC50: 2.9 × 10−9 M vs. 6.8 × 10−9 M, respectively) (Fig. 3c). Ligand-induced transcriptional activities were determined in HEK 293 cells transiently transfected with expression vectors encoding the full-length hVDR and a luciferase reporter plasmid encompassing the promoter region of hCYP24A1. We found that VDR transcriptional activity is induced by lower doses of ligand 1 as compared with 1,25D, with EC50 of 1.4 × 10−10 M (Fig. 3d). In addition, using MCF-7 cells, we evaluated the effects of carborane 1 administration on a vitamin D target gene by real-time PCR. 1,25D and carborane 1 showed similar biological potency to induce CYP24A1 mRNA expression (Fig. 3e). Finally, serum calcium levels were evaluated in mice 21 days after intraperitoneal administration of 1,25D or the carborane 1. Mice treated with carborane 1 did not develop hypercalcemia and they had significantly lower calcium levels than 1,25D-injected mice (P = 0.03, Fig. 3f). Thus, carborane 1 strongly binds to VDR, induces similar biological activities and displays reduced calcemic effects.
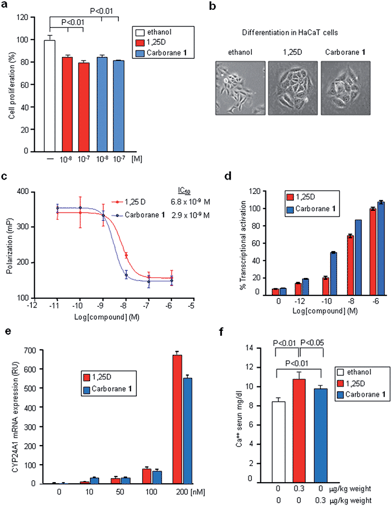 |
| Fig. 3 Biological characterization of the carborane 1. (a) Cell proliferation in human MCF-7 breast adenocarcinoma cells. P < 0.01 vs. control cells. (b) Differentiation activity in human HaCaT keratinocytes cells. (c) Vitamin D receptor binding. Competitive binding of 1,25D and carborane 1 to the full-length human VDR. IC50 values are derived from dose–response curves. (d) Transcriptional responses of hVDR in HEK293 EBNA cells. (e) Real-time PCR of CYP24A1 mRNA levels in human MCF-7 breast cancer cells. (f) Calcium levels in sera of mice treated with 1,25D and carborane 1. Error bars represent standard deviation (SD). | |
Crystal structure
To decipher the mode of recognition of carborane 1 by the VDR, we co-crystallized the zebrafish wild-type VDR LBD in complex with the ligand and the coactivator peptide corresponding to the second LXXLL motif of SRC-2 and solved the structure with a resolution up to 2.4 Å. Although numerous carborane ligands for nuclear receptors have been synthesized27–29 very few of them have been crystallized as protein-ligand complexes.16 The data collection and refinement statistics of the structure are summarized in ESI Table 1.‡ The overall structure (Fig. 4a) is highly homologous to the VDR-1,25D structure with the insertion region between helices H2 and H3 not visible in the electron density map indicating local disorder of this region.30 Despite the bulky carborane moiety at the end of the side chain, the ligand-VDR complex adopts the canonical active conformation of the 1,25-VDR complex. The position and conformation of the activated helix H12 in the zVDR complex is strictly maintained in its agonistic position (Fig. 4a).30 When compared to the zVDR LBD-1,25D structure, the Cα atoms of the zVDR LBD–carborane complex have a root mean square deviation of 0.3 Å over 238 residues. However, small shifts in secondary structural elements are observed for the loop H6–H7 and the last turn of helix H11, shifted by 0.6 Å. The ligand is buried in the predominantly hydrophobic pocket and adopts the same orientation as 1,25D (Fig. 4b and c). Regarding the interface VDR/coactivator peptide, we observed that contacts between protein residues and the coactivator peptide are conserved. The C1–OH and C3–OH groups of the ligand form similar H-bonds as 1,25D.
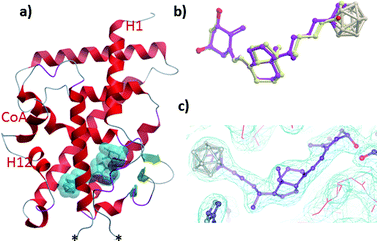 |
| Fig. 4 (a) Overall structure of the zVDR LBD bound to the carborane 1 and the coactivator peptide. The stars indicate the insertion region not visible in the electron density map. (b) Overlay of 1,25D (carbon atoms in yellow and oxygen atoms in red) with 1 (violet) within zVDR LBD complexes. (c) The carborane 1 shown in the 2Fo–Fc electron density map contoured at 1σ. | |
The secosteroidal part of the ligand forms similar interactions with the zVDR ligand binding pocket compared to 1,25D. The side-chain adopts a conformation slightly different than that observed for 1,25D as a consequence of the carborane moiety and the longer length of the side chain (8.9 Å instead of 6.5 Å). The carborane group forms extensive interactions with Leu255, Leu258, Ala259, Val262, Ala331, His333, His423, Tyr427, Leu430, and C-terminal residues: Leu440, Val444 and Phe448. Stronger contacts are observed with Ala259, Ala331 and Leu440 compared to 1,25D. The two histidines His333 and His423 interacts with boron atoms (Fig. 5). Although the resolution of the crystal structure (2.4 Å) is not sufficient to resolve the position of the H atoms, on the basis of the hydride-like hydrogens at the boron atoms, we considered that in the VDR–carborane complex the two histidines interact with boron atoms through unconventional (BH⋯HN) dihydrogen bonds (Fig. 5), replacing the H-bonds formed between the C25–OH group of the natural ligand and both histidines.
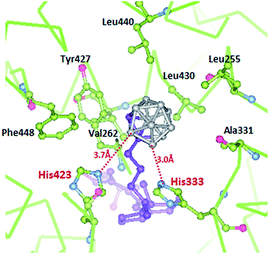 |
| Fig. 5 Details of the interactions mediated by the carborane moiety of ligand 1 with residues of the zVDR LBD at a 3.8 Å distance cutoff. Dihydrogen bonds between boron atoms and His333 and His423 are shown by red dashed lines. Distances are shown in Å. | |
As hydrogen atoms at CH of carborane are far more acidic than those at BH, interaction between carborane CH and histidine would also be possible.31 Unconventional dihydrogen bonds with amino acids residues have been already reported.32 Overall, the numerous contacts among the atoms of the carborane and hydrophobic residues of H3, H11 and H12 significantly stabilize the agonist conformation of VDR in agreement with the agonist potency of this novel compound. The crystal structures of some of these p-carborane VD mimics revealed weaker H-bonds compared to 1,25D and lack the crucial CH–π interaction with Trp.18 Remarkably, our carborane 1 that occupies the crucial anchoring points of the natural ligand to VDR, binds tighter (2.4×) to VDR and is slightly more potent in transcriptional activation than 1,25D.
Conclusions
An unique secosteroidal vitamin D analog bearing an o-carborane unit at the side chain has been designed, synthesized and biologically tested. In comparison with the natural hormone 1,25D, the novel analog, which lacks the 25-hydroxy group, strongly binds to VDR, induces similar biological activities and displays reduced calcemic effects. The carborane unit mimics the interaction of the 25-hydroxyl group of the natural hormone with the VDR and shows additional hydrophobic contacts between the boron atoms and important residues of helix H12. The crystal structure of the analog in complex with the zebrafish wild-type VDR-LBD, reveals that the carborane cage interacts efficiently through extensive van der Waals interactions and dihydrogen bonds with the two histidines His333 and His423. These results establish the basis for the rational design of new unconventional vitamin D analogs containing carborane moieties for specific molecular recognition, and drug research and development.
Acknowledgements
We thank the Spanish Ministry of Economy and Innovation (MEI, SAF2010-15291 and SAF2012-38240), Xunta de Galicia (project GPC2014/001), Agence Nationale de la Recherche (ANR-13-BSV8-0024-01), French Infrastructure for Integrated Structural Biology (FRISBI) (ANR-10-INSB-05-01), and INSTRUCT as part of the European Strategy Forum on Research Infrastructures (ESFRI), for financial support and CESGA for computing time. R. O. thanks the Spanish MEI for an FPI fellowship (BES-2011-0419192). S. S. thanks the Asociación Española Contra el Cáncer for a fellowship (AIOA1101SEOA). R. S. thanks the Xunta de Galicia for a postdoctoral fellowship (POS-A/2012/112). We thank Oliver Smart (Global Phasing Ltd), Alastair McEwen (IGBMC) and the staff of PX1 (SOLEIL synchrotron) for their collaborations.
Notes and references
- V. I. Bregadze, Chem. Rev., 1992, 92, 209 CrossRef CAS.
- E. Meggers, Angew. Chem., Int. Ed., 2011, 50, 2442 CrossRef CAS PubMed.
- M. Scholz and E. Hey-Hawkins, Chem. Rev., 2011, 111, 7035 CrossRef CAS PubMed.
- F. Issa, M. Kassiou and L. M. Rendina, Chem. Rev., 2011, 111, 5701 CrossRef CAS PubMed.
- M. F. Hawthorne, Angew. Chem., Int. Ed., 1993, 32, 950 CrossRef.
- A. H. Soloway, W. Tjarks, B. A. Barnum, F.-G. Rong, R. F. Barth, I. M. Codogni and J. G. Wilson, Chem. Rev., 1998, 98, 1515 CrossRef CAS PubMed.
- J. F. Valliant, K. J. Guenther, A. S. King, P. Morel, P. Schaffer, O. O. Sogbein and K. A. Stephenson, Coord. Chem. Rev., 2002, 232, 173 CrossRef CAS.
- A. F. Armstrong and J. F. Valliant, Dalton Trans., 2007, 4240 RSC.
- D. A. Feakes, R. C. Waller, D. K. Hathaway and V. S. Morton, Proc. Natl. Acad. Sci. U. S. A., 1999, 96, 6406 CrossRef CAS.
- D. Alberti, A. Toppino, S. G. Crich, C. Meraldi, C. Prandi, N. Protti, S. Bortolussi, S. Altieri, S. Aime and A. Deagostino, Org. Biomol. Chem., 2014, 12, 2457 CAS.
- D. D. Bikle, Chem. Biol., 2014, 21, 319 CrossRef CAS PubMed.
- K. K. Deeb, D. L. Trump and C. S. Johnson, Nat. Rev. Cancer, 2007, 7, 684 CrossRef CAS PubMed.
- D. Feldman, A. V. Krishnan, S. Swami, E. Giovannucci and B. J. Feldman, Nat. Rev. Cancer, 2014, 14, 342 CrossRef CAS PubMed.
- S. Santagata, A. Thakkar, A. Ergonul, B. Wang, T. Woo, R. Hu, J. C. Harrell, G. McNamara, M. Schwede, A. C. Culhane, D. Kindelberger, S. Rodig, A. Richardson, S. J. Schnitt, R. M. Tamimi and T. A. Ince, J. Clin. Invest., 2014, 124, 859 CAS.
- S. Fujii, H. Masuno, Y. Taoda, A. Kano, A. Wongmayura, M. Nakabayashi, N. Ito, M. Shimizu, E. Kawachi, T. Hirano, Y. Endo, A. Tanatani and H. Kagechika, J. Am. Chem. Soc., 2011, 133, 20933 CrossRef CAS PubMed.
- A. Wongmayura, S. Fujii, S. Ito, A. Kano, Y. Taoda, E. Kawachi, H. Kagechika and A. Tanatani, Bioorg. Med. Chem. Lett., 2012, 22, 1756 CrossRef CAS PubMed.
- S. Fujii, A. Kano, C. Songkram, H. Masuno, Y. Taoda, E. Kawachi, T. Hirano, A. Tanatani and H. Kagechika, Bioorg. Med. Chem., 2014, 22, 1227 CrossRef CAS PubMed.
- S. Yamada and M. Makishima, Trends Pharmacol. Sci., 2014, 35, 324 CrossRef CAS PubMed.
- S. Fujii, R. Sekine, A. Kano, H. Masuno, C. Songkram, E. Kawachi, T. Hirano, A. Tanatani and H. Kagechika, Bioorg. Med. Chem., 2014, 22, 5891 CrossRef CAS PubMed.
- N. Rochel, J. M. Wurtz, A. Mitschler, B. Klaholz and D. Moras, Mol. Cell, 2000, 5, 173 CrossRef CAS PubMed.
- P. Gogoi, R. Sigüeiro, S. Eduardo and A. Mouriño, Chem.–Eur. J., 2000, 16, 1432 CrossRef PubMed.
- H. H. Inhoffen, G. Quinckert, S. Schuzt, G. Friedrich and E. Tober, Chem. Ber., 1958, 91, 781 CrossRef CAS.
- J. Bian, M. Van Wingerden and J. M. Ready, J. Am. Chem. Soc., 2006, 128, 7428 CrossRef CAS PubMed.
- B. M. Trost, J. Dumas and M. Villa, J. Am. Chem. Soc., 1992, 114, 9836 CrossRef CAS.
- A. Mouriño, M. Torneiro, C. Vitale, S. Fernández, J. Pérez-Sestelo, S. Anné and C. Gregorio, Tetrahedron Lett., 1997, 38, 4713 CrossRef.
- J. Wietrzyk, M. Chodynski, H. Fitak, E. Wojdat, A. Kutner and A. Opolski, Anti-Cancer Drugs, 2007, 18, 447 CrossRef CAS PubMed.
- Y. Endo, T. Yoshimi, K. Ohta, T. Suzuki and S. Ohta, J. Med. Chem., 2005, 48, 3941 CrossRef CAS PubMed.
- T. Goto, K. Ohta, S. Fuji, S. Ohta and Y. Endo, J. Med. Chem., 2010, 53, 4917 CrossRef CAS PubMed.
- T. Iijima, Y. Endo, M. Tsuji, E. Kawachi, H. Kagechika and K. Shudo, Chem. Pharm. Bull., 1999, 47, 398 CrossRef CAS PubMed.
- F. Ciesielski, N. Rochel and D. Moras, J. Steroid Biochem. Mol. Biol., 2007, 103, 235 CrossRef CAS PubMed.
- J. M. Oliva, P. von Ragué Schleyer, G. Aullón, J. I. Burgos, A. Fernández-Barbero and I. Alkorta, Phys. Chem. Chem. Phys., 2010, 12, 5101 RSC.
- J. Fanfrlík, M. Lepsík, D. Horinek, Z. Havlas and P. Hobza, ChemPhysChem, 2006, 7, 1100 CrossRef PubMed.
Footnotes |
† Accession number. The coordinates and structure factors are deposited in the Protein Data Bank under the accession code 5E7V. |
‡ Electronic supplementary information (ESI) available: Experimental procedures, computational details, spectroscopic data and copies of 1H and 13C NMR spectra and characterization data for all new compounds. See DOI: 10.1039/c5sc03084f |
§ R. Otero and S. Seoane contributed equally. |
|
This journal is © The Royal Society of Chemistry 2016 |
Click here to see how this site uses Cookies. View our privacy policy here.