DOI:
10.1039/C5SC04091D
(Edge Article)
Chem. Sci., 2016,
7, 2151-2156
A smart “off–on” gate for the in situ detection of hydrogen sulphide with Cu(II)-assisted europium emission†
Received
28th October 2015
, Accepted 7th December 2015
First published on 7th December 2015
Introduction
Hydrogen sulphide (H2S) is the smallest bioactive thiol that may act as a gaseous signalling agent,1 and its production in different tissue types is associated with a wide range of physiological responses such as vascular smooth muscle relaxation,2 mitochondrial ATP production,3 insulin-signalling inhibition,4 regulation of inflammation response5 and mediation of neurotransmission.6 Moreover, recent investigations show that abnormal levels of H2S are associated with a variety of diseases, such as neurodegenerative diseases,7 diabetes8 and cancer.9 However, the biological targets of H2S and the mechanisms of these H2S-related physiological phenomena remain unclear. Therefore the development of responsive and reversible luminescence probes for non-invasive real time monitoring of H2S may be useful for understanding its biological modes of action.
One of the major approaches for developing luminescence H2S detection10 is based on sulphide-specific chemical reactions, such as reduction of an azide11 and nucleophilic addition of a sulphide ion.12 This type of luminescence probe is generally irreversible and usually requires a considerably long incubation time. An alternative approach is based on CuS precipitation13 due to the low-solubility of CuS (Ksp = 6.3 × 10−36). These luminescence probes are generally reversible with low detection limits. We are particularly interested in developing H2S luminescence sensors based on organo-lanthanide complexes due to their water-solubility and unique photophysical properties, including line-like emission spectra and long luminescence lifetimes (micro to milli second scale) that can effectively separate the observing signal from biological autofluorescence noise and are suitable for time-gated detection. Recently, a few studies have been found in the literature with irreversible H2S lanthanide probes.12a Herein, we report the development of a novel responsive europium-based luminescence “off–on” gate for the in situ detection of H2S in water.
As illustrated in Fig. 1, EuL1 contains a DO3A–Eu3+ complex and an aza-18-crown-6 moiety, which are linked to the 2- and 6-positions of a pyridine-containing chromophore constituting a switch-like structure. In the ground state, EuL1 should be emissive due to the coordination of the pyridine chromophore to a Eu3+ ion, which favours energy transfer from the organic chromophore to the Eu3+ ion. Upon binding of the aza-18-crown-6 moiety with a Cu2+ ion, pyridine is expected to coordinate with the Cu2+ ion, resulting in luminescence quenching. The europium emission should be recovered after the displacement of the Cu2+ ion upon copper sulphide precipitation.
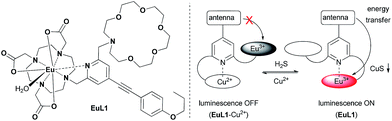 |
| Fig. 1 The structure of EuL1 and the illustration of the design of a reversible Eu-based luminescence probe (EuL1–Cu2+) for H2S detection. | |
Results and discussion
Synthesis and photophysical properties of L1 and EuL1
Ligand L1 was readily prepared from (4-iodopyridine-2,6-diyl)dimethanol (1)14via a desymmetrization synthetic strategy. As shown in Scheme 1, a pyridine-containing chromophore (based on a D–π–A motif) was established via a Sonogashira cross-coupling reaction between 1 and 1-ethynyl-4-propoxybenzene (2).15 After converting both hydroxyl groups of 3 into the corresponding bromide, the aza-18-crown-6 and DO3A moieties were incorporated into 4 sequentially under basic conditions and afforded L1 in good yields. L1 was fully characterized using 1H and 13C NMR spectroscopy and HRMS. Finally, acid hydrolysis of the t-butyl esters followed by Eu complex formation provided EuL1, which was characterized unambiguously using HRMS and HPLC (Table S1 and Fig. S1†).
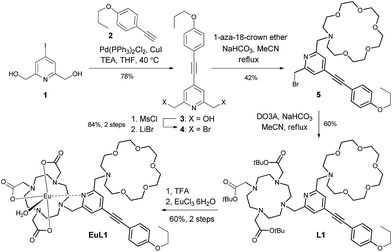 |
| Scheme 1 Synthesis of L1 and EuL1. | |
In the UV-vis absorption spectrum, L1 showed strong absorption bands at 235 and 310 nm in methanol which are attributed to the π to π* transitions. The absorption bands were broadened and red-shifted in EuL1 (245 and 333 nm, ε333 nm = 7560 M−1 cm−1) in water (Fig. S2†). The excitation spectrum of EuL1 at 615 nm showed maxima at 240 and 340 nm (Fig. S2†), evidencing an antenna effect due to energy transfer from the ligand to the Eu3+ ion. The 5D0 → 7FJ transitions of EuL1 (λex = 325 nm) were found at 578 (J = 0), 585–603 (J = 1), 604–637 (J = 2), 646–658 (J = 3), and 673–712 nm (J = 4) in the emission spectrum (Fig. 2). The quantum yield of EuL1 corresponding to the 5D0 → 7F2 transitions of Eu3+ ions in water is 0.5% (Table S2†).
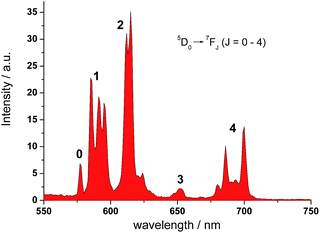 |
| Fig. 2 Emission spectrum of EuL1 (H2O, λex = 325 nm, 10 μM). | |
Fluorimetric titration studies of EuL1
With EuL1 in hand, its binding properties towards Cu2+ ions were investigated. Upon the addition of 1 equiv. of Cu2+ ions (CuCl2 as the source of Cu2+ ions), the absorption maximum of EuL1 showed a slight red shift and the absorption ability slightly decreased due to the effect of the copper metal. In a titration study, EuL1 exhibited a 17-fold quenching of the europium emission with an excess of Cu2+ ions and the Benesi–Hildebrand plot showed a 1
:
1 binding stoichiometry with KB = 1.2 × 105 M−1 (inset of Fig. 3a).16 The Job's plot also supported the formation of a EuL1–Cu2+ complex in a 1
:
1 ratio (Fig. S3†). In a competitive study, the addition of a large excess of various metal ions, such as Na+, K+, Ca2+, Mg2+, Ba2+, Co2+, Zn2+, Ni2+, Fe2+, Mn2+, Cu+ and Li+ ions, to EuL1 resulted in only slight luminescence changes (red columns in Fig. 3b). The subsequent addition of excess Cu2+ ions caused significant luminescence quenching (blue columns in Fig. 3b). These results indicate the high selectivity of EuL1 towards Cu2+ ions and that the binding between EuL1 and Cu2+ ions is not interfered by other metal ions. In a pH study, EuL1 remains highly emissive and was quenched by Cu2+ ions in the pH range 6 to 8 (Fig. S4†), indicating that EuL1 is stable and can bind to Cu2+ ions under physiological conditions.
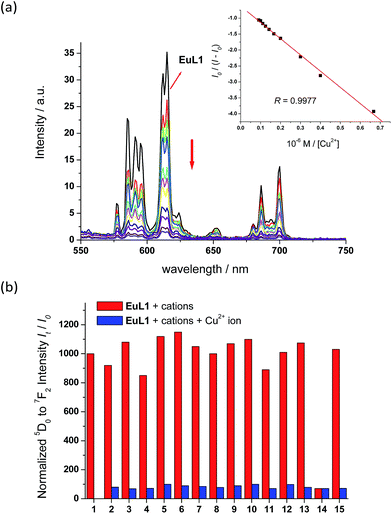 |
| Fig. 3 (a) Fluorimetric titration of EuL1 (10 μM) towards Cu2+. The inset shows the plot of I0/(I − I0) vs. [Cu2+] (0–20 μM). I and I0 stand for intensity of europium emission 5D0 → 7F2. (b) Effects of various metal ions on the luminescence intensity of EuL1 (10 μM). 1: EuL1 only; 2: Na+; 3: K+; 4: Ca2+; 5: Mg2+; 6: Ba2+; 7: Co2+; 8: Zn2+; 9: Ni2+; 10: Fe2+; 11: Mn2+; 12: Cu+; 13: Li+; 14: Cu2+; 15: all of the above metal ions except Cu2+. All spectra were acquired in water with excitation at 325 nm. | |
To study the reversibility of the binding between EuL1 and Cu2+ ions, a small amount of H2S (Na2S as the source of H2S) was added. The EuL1–Cu2+ complex responded instantaneously (requiring only 40 s to reach saturation without stirring or shaking) (Fig. S5†), and Eu emission resumed with a similar profile for the emission spectrum to that of EuL1 (Fig. 4). This result indicated that the DO3A–Eu3+ complex was not displaced by a Cu2+ ion, forming the EuL1–Cu2+ complex in the previous step. More interestingly, Eu emission was further enhanced (40-fold) with an excess of H2S and the Eu3+ emission profile showed significant changes, suggesting binding between EuL1 and H2S (Fig. 5a). The Benesi–Hildebrand plot showed a 1
:
1 binding stoichiometry with KB = 1.5 × 104 M−1 (inset of Fig. 5a).16 The detection limit of EuL1 towards H2S was calculated according to the 3SD/slope as low as 60 nM. Surprisingly, direct titration of EuL1 against H2S resulted in only about a 5-fold luminescence enhancement with a non-linear relationship in the 1
:
1 Benesi–Hildebrand plot (Fig. 6). These results indicated that the Cu2+ ion facilitates the specific 1
:
1 binding of EuL1 and H2S, presumably via pre-organizing the conformation of EuL1. On the other hand, non-specific binding (possibly a mixture of 1
:
1 and 2
:
1 binding) between EuL1 and H2S resulted without the favourable conformation that is induced by the pre-complexation of a Cu2+ ion. This proposal was further supported by the dramatic luminescence drop of the EuL1–Na2S complex upon heating (>70 °C) (Fig. S6†). This type of Cu2+-assisted luminescence enhancement of Eu emission is unprecedented. In a competitive study, EuL1–Cu2+ showed insignificant changes in luminescence with a large excess of anions, including Cl−, SO42−, HSO4−, I−, CO32−, HPO42−, Br− and HCO3−, and only small changes for GSH and cysteine (red columns in Fig. 5b). Upon the addition of H2S, the Eu emissions were recovered in all the above cases, indicating a high selectivity of EuL1–Cu2+ towards H2S.
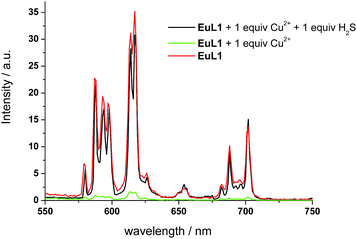 |
| Fig. 4 The emission spectra of EuL1 (10 μM) (red), with 1 equiv. of Cu2+ ions (green), and with 1 equiv. of Cu2+ ions and 1 equiv. of H2S (black). All spectra were acquired in water with λex at 325 nm. | |
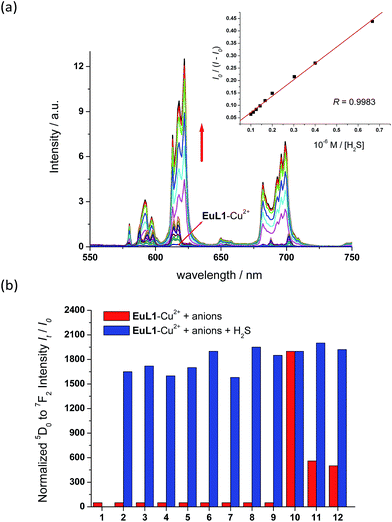 |
| Fig. 5 (a) Fluorimetric titration of EuL1–Cu2+ (10 μM, generated in situ with 2 equiv. of Cu2+) towards H2S (0–100 μM). The inset shows the plot of I0/(I − I0) vs. [Na2S] (0–100 μM). I and I0 stand for intensity of europium emission 5D0 → 7F2. (b) Effects of various anions on the luminescence intensity of EuL1 (10 μM). 1: EuL1 only; 2: Cl−; 3: SO42−; 4: HSO4−; 5: I−; 6: CO32−; 7: HPO42−; 8: Br−; 9: HCO3−; 10: S2−; 11: GSH; 12: cysteine. All spectra were acquired in water with excitation at 325 nm. | |
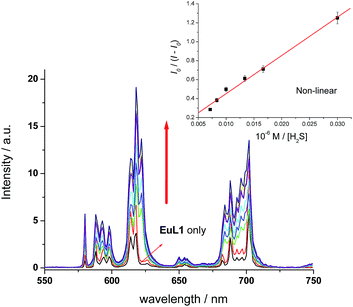 |
| Fig. 6 Fluorimetric titration of EuL1 (10 μM) towards H2S (0–300 μM). The inset shows the plot of I0/(I − I0) vs. [H2S] (0–300 μM). I and I0 stand for intensity of europium emission 5D0 → 7F2. All spectra were acquired in water with λex at 325 nm. | |
Mechanistic studies
The binding mechanisms of EuL1 towards Cu2+ ions and the EuL1–Cu2+ complex towards H2S were studied using a comparative analysis of the emission spectra of the Eu complexes and the 1H NMR spectra of La complexes.17 As shown in Fig. 7, the profile of the emission spectrum of EuL1 did not change significantly upon the addition of Cu2+ ions. Comparing [EuL1], [EuL1 + Cu2+] and [EuL1 + Cu2+ + H2S], measured under the same solution conditions, similar spectra were observed for [EuL1] and [EuL1 + Cu2+] (5D0 → 7F1
:
7F2
:
7F4 of [EuL1] = 1
:
1.122
:
0.55 and 5D0 → 7F1
:
7F2
:
7F4 [EuL1 + Cu2+] = 1
:
1.186
:
0.91, Table 1). This is correlated with the NMR data and shows that the Cu2+ ion is coordinated in the aza-crown. However, signal broadening was observed in the 1H NMR spectrum of LaL1, indicating rapid metal–ligand exchange. These results suggested that the pyridine moiety of the organic chromophore is rapidly switching between the DO3A–Eu3+ and aza-18-crown-6–Cu2+ complexes, causing significant luminescence quenching. Moreover, the binding of Cu2+ would also provide a favourable conformation for forming a new 1
:
1 complex with H2S. Upon the addition of H2S, the emission profile of EuL1 changed significantly, ΔJ = 2/ΔJ = 1 for [EuL1 + Cu2+ + H2S],18 and the intensity ratio was about >200% higher for [EuL1] and [EuL1 + Cu2+]. This increase can be attributed to the lower symmetry of the complexes with the addition of sulphide ions (Fig. 7) and the 1H NMR signals of LaL1 were sharpened. These results suggested new complex formation after the displacement of the Cu2+ ion via CuS precipitation. This proposal is further supported by the HRMS spectrum of the EuL1–Na2S complex (Fig. S7†) and the change in the quantum yields (Table S2†). The EuL1–Na2S complex is highly emissive probably due to its rigid structure.
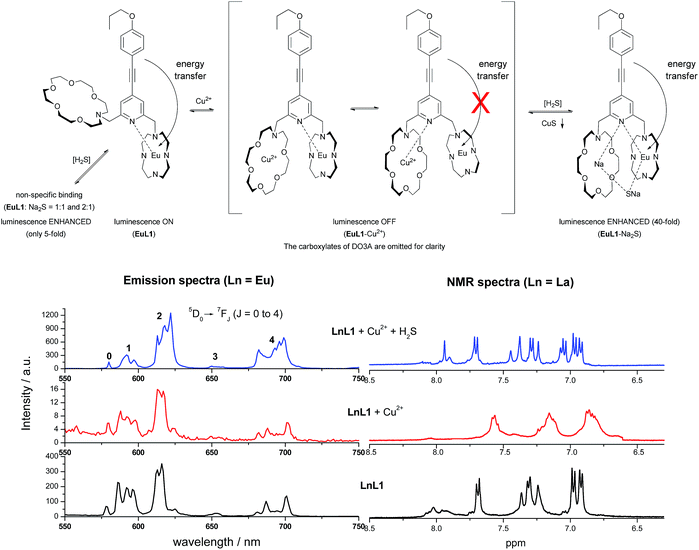 |
| Fig. 7 Top: proposed binding mechanism of EuL1 towards Cu2+ and H2S (Na2S as the source of H2S). Bottom left: emission spectra of the Eu complexes (λex = 325 nm). Bottom right: 1H NMR spectra of the La complexes (6.5–8.5 ppm). | |
Table 1 The ratio of 5D0 → 7FJ (J = 0 to 4) emission bands of EuL1, EuL1 + Cu2+ and EuL1 + Cu2+ + H2Sa
5D0→ |
7F0 |
7F1 |
7F2 |
7F3 |
7F4 |
All spectra were acquired in water with excitation at 325 nm.
|
EuL1
|
0.01 |
1 |
1.22 |
0.08 |
0.55 |
EuL1 + Cu2+ |
0.08 |
1 |
1.86 |
0.15 |
0.91 |
EuL1 + Cu2+ + H2S |
0.48 |
1 |
3.98 |
0.15 |
1.95 |
The proposed binding mechanism was also examined using a series of negative control compounds (Fig. 8).19EuL2 showed no luminescence quenching upon the addition of Cu2+ ions (Fig. 9a). This result indicated that the carbonyl linker of aza-18-crown-6 may be too rigid for coordination between Cu2+ and pyridine, which could be essential for Eu emission quenching. Without the aza-crown moiety, EuL3 also showed no luminescence quenching towards Cu2+ (Fig. 9b), suggesting DO3A–Eu3+ is stable with Cu2+ and the aza-crown motif is important for the Cu2+ binding. L4 bearing the pyridine-chromophore showed profound luminescence quenching, but its phenyl analogue (L5) showed no significant change in luminescence upon the addition of Cu2+ ions (Fig. 9c and d). These results indicated that the pyridine moiety of the chromophore is essential for the binding of Cu2+ to the aza-crown moiety. The results of this series of negative control compounds are in full agreement with the proposed mechanism in Fig. 7.
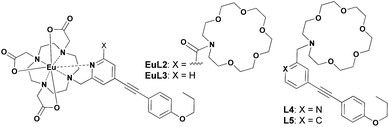 |
| Fig. 8 The structures of the negative control compounds EuL2, EuL3, L4 and L5. | |
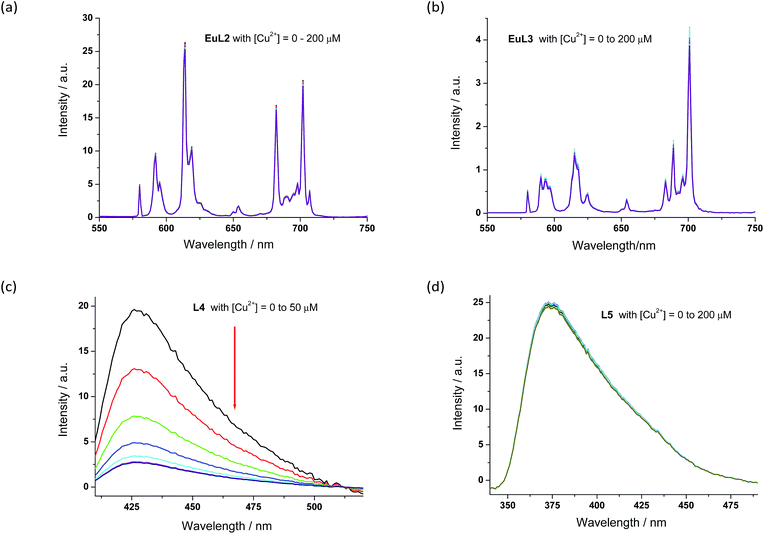 |
| Fig. 9 The emission spectra of negative control compounds (10 μM) with various concentration of Cu2+ ions. (a): EuL2; (b): EuL3; (c): L4; (d): L5. All spectra were acquired in water with λex at 325 nm. | |
Conclusions
In summary, we have prepared a water-soluble and emissive Eu-complex (EuL1) based on a DO3A(Eu3+)–pyridine–aza-crown motif, and studied its consecutive binding properties towards Cu2+ and H2S extensively. EuL1 binds to Cu2+ ions selectively (KB = 1.2 × 105 M−1) inducing 17-fold luminescence quenching and forming a 1
:
1 stoichiometric complex (EuL1–Cu2+), which responds to H2S selectively with restoration of the original EuL1 emission followed by a further 40-fold luminescence enhancement and a nano-molar detection limit (60 nM). Mass spectroscopic analysis showed the formation of a 1
:
1 stoichiometric complex (EuL1–Na2S) with KB = 1.5 × 104 M−1. Without Cu2+ ions, EuL1 shows non-specific binding towards H2S with only a 5-fold luminescence enhancement. These results indicate that the Cu2+ ion may pre-organize the conformation of EuL1 and facilitate the formation of the EuL1–Na2S complex. The studies on this unprecedented Cu2+-assisted luminescence enhancement of Eu emission are still ongoing. With long-lived Eu emission, reversible binding properties, an instantaneous response and high selectivity towards H2S, this Eu-based luminescence “off–on” gate could find suitable applications for H2S imaging in biological systems.
Acknowledgements
This work is funded by the Peking University Shenzhen Graduate School (Key State Laboratory of Chemical Genomics open-project fellowship program), grants from Shenzhen Science, Technology Innovation Committee (KQTD201103), Nanshan (KC2014ZDZJ0026A), Hong Kong Baptist University (HKBU) (FRG2/14-15/013013), Hong Kong Polytechnic University (HKPolyU), Hong Kong Research Grants Council (HKBU 203012), Hong Kong Polytechnic University central Research Grant (G-UC08), Natural Science Foundation of China (21401158) and HKBU and HKPolyU Joint Research Programme (RC-ICRS/15-16/02F-WKL02F-WKL).
Notes and references
-
(a) B. Olas, Clin. Chim. Acta, 2015, 439, 212 CrossRef CAS PubMed;
(b) H. Kimura, Antioxid. Redox Signaling, 2014, 20, 783 CrossRef CAS PubMed;
(c) H. Kimura, N. Shibuya and Y. Kimura, Antioxid. Redox Signaling, 2012, 17, 45 CrossRef CAS PubMed;
(d) C. Szabó, Nat. Rev. Drug Discovery, 2007, 6, 917 CrossRef PubMed.
- G. D. Yang, L. Y. Wu, B. Jiang, W. Yang, J. S. Qi, K. Cao, Q. H. Meng, A. K. Mustafa, W. T. Mu, S. M. Zhang, S. H. Snyder and R. Wang, Science, 2008, 322, 587 CrossRef CAS PubMed.
-
(a) M. Fu, W. Zhang, L. Wu, G. Yang, H. Li and R. Wang, Proc. Natl. Acad. Sci. U. S. A., 2012, 109, 2943 CrossRef CAS PubMed;
(b) G. A. Benavides, G. L. Squadrito, R. W. Mills, H. D. Patel, T. S. Isbell, R. P. Patel, V. M. Darley-Usmar, J. E. Doeller and D. W. Kraus, Proc. Natl. Acad. Sci. U. S. A., 2007, 104, 17977 CrossRef CAS PubMed.
-
(a) Y. Kaneko, Y. Kimura, H. Kimura and I. Niki, Diabetes, 2006, 55, 1391 CrossRef CAS PubMed;
(b) W. Yang, G. D. Yang, X. M. Jia, L. Y. Wu and R. Wang, J. Physiol., 2005, 569, 519 CrossRef CAS PubMed.
-
(a) Y. J. Peng, J. Nanduri, G. Raghuraman, D. Souvannakitti, M. M. Gadalla, G. K. Kumar, S. H. Snyder and N. R. Prabhakar, Proc. Natl. Acad. Sci. U. S. A., 2010, 107, 10719 CrossRef CAS PubMed;
(b) L. Li, M. Bhatia, Y. Z. Zhu, Y. C. Zhu, R. D. Ramnath, Z. J. Wang, F. B. M. Anuar, M. Whiteman, M. Salto-Tellez and P. K. Moore, FASEB J., 2005, 19, 1196 CAS.
- K. Abe and H. J. Kimura, J. Neurosci., 1996, 16, 1066 CAS.
-
(a) B. D. Paul, J. I. Sbodio, R. Xu, M. S. Vandiver, J. Y. Cha, A. M. Snowman and S. H. Snyder, Nature, 2014, 509, 96 CrossRef CAS PubMed;
(b) L. F. Hu, M. Lu, C. X. Tiong, G. S. Dawe, G. Hu and J. S. Bian, Aging Cell, 2010, 9, 135 CrossRef CAS PubMed;
(c) D. Giuliani, A. Ottani, D. Zaffe, M. Galantucci, F. Strinati, R. Lodi and S. Guarini, Neurobiol. Learn. Mem., 2013, 104, 82 CrossRef CAS PubMed.
-
(a) L. Wu, W. Yang, X. Jia, G. Yang, D. Duridanova, K. Cao and R. Wang, Lab. Invest., 2009, 89, 59 CrossRef CAS PubMed;
(b) W. Yang, G. Yang, X. Jia, L. Wu and R. Wang, J. Physiol., 2005, 569, 519 CrossRef CAS PubMed.
-
(a) J. Huang, S. Kumar, N. Abbassi-Ghadi, P. Španěl, D. Smith and G. B. Hanna, Anal. Chem., 2013, 85, 3409 CrossRef CAS PubMed;
(b) C. Szabó, C. Coletta, C. Chao, K. Módis, B. Szczesny, A. Papapetropoulos and M. R. Hellmich, Proc. Natl. Acad. Sci. U. S. A., 2013, 110, 12474 CrossRef PubMed.
- For reviews, see:
(a) V. S. Lin, W. Chen, M. Xian and C. J. Chang, Chem. Soc. Rev., 2015, 44, 4596 RSC;
(b) E. L. Que, D. W. Domaille and C. J. Chang, Chem. Rev., 2008, 108, 1517 CrossRef CAS PubMed.
- For selected examples, see:
(a) M. Tropiano and S. Faulkner, Chem. Commun., 2014, 50, 4696 RSC;
(b) V. S. Lin, A. R. Lippert and C. J. Chang, Proc. Natl. Acad. Sci. U. S. A., 2013, 110, 7131 CrossRef CAS PubMed;
(c) S. Chen, Z.-J. Chen, W. Ren and H.-W. Ai, J. Am. Chem. Soc., 2012, 134, 9589 CrossRef CAS PubMed;
(d) A. R. Lippert, E. J. New and C. J. Chang, J. Am. Chem. Soc., 2011, 133, 10078 CrossRef CAS PubMed;
(e) H. Peng, Y. Cheng, C. Dai, A. L. King, B. L. Predmore, D. J. Lefer and B. A. Wang, Angew. Chem., Int. Ed., 2011, 50, 9672 CrossRef CAS PubMed.
- For selected examples, see:
(a) J. Cao, R. Lopez, J. M. Thacker, J. Y. Moon, C. Jiang, S. N. S. Morris, J. H. Bauer, P. Tao, R. P. Masonc and A. R. Lippert, Chem. Sci., 2015, 6, 1979 RSC;
(b) Z. Huang, S. Ding, D. Yu, F. Huang and G. Feng, Chem. Commun., 2014, 50, 9185 RSC;
(c) X. Li, S. Zhang, J. Cao, N. Xie, T. Liu, B. Yang, Q. He and Y. Hu, Chem. Commun., 2013, 49, 8656 RSC;
(d) Y. Qian, L. Zhang, S. Ding, X. Deng, C. He, X. E. Zheng, H.-L. Zhu and J. Zhao, Chem. Sci., 2012, 3, 2920 RSC;
(e) Y. Qian, J. Karpus, O. Kabil, S.-Y. Zhang, H.-L. Zhu, R. Banerjee, J. Zhao and C. He, Nat. Commun., 2011, 2, 495 CrossRef PubMed.
- For selected examples, see:
(a) L. E. Santos-Figueroa, C. de la Torre, S. El Sayed, F. Sancenón, R. Martínez-Máñez, A. M. Costero, S. Gil and M. Parra, Eur. J. Inorg. Chem., 2014, 41 CrossRef CAS;
(b) X. Qu, C. Li, H. Chen, J. Mack, Z. Guo and Z. Shen, Chem. Commun., 2013, 49, 7510 RSC;
(c) M.-Q. Wang, K. Li, J.-T. Hou, M.-Y. Wu, Z. Huang and X.-Q. Yu, J. Org. Chem., 2012, 77, 8350 CrossRef CAS PubMed;
(d) F. Hou, J. Cheng, P. Xi, F. Chen, L. Huang, G. Xie, Y. Shi, H. Liu, D. Bai and Z. Zeng, Dalton Trans., 2012, 41, 5799 RSC;
(e) F. Hou, L. Huang, P. Xi, J. Cheng, X. Zhao, G. Xie, Y. Shi, F. Cheng, X. Yao, D. Bai and Z. Zeng, Inorg. Chem., 2012, 51, 2454 CrossRef CAS PubMed;
(f) K. Sasakura, K. Hanaoka, N. Shibuya, Y. Mikami, Y. Kimura, T. Komatsu, T. Ueno, T. Terai, H. Kimura and T. Nagano, J. Am. Chem. Soc., 2011, 133, 18003 CrossRef CAS PubMed.
- L. C. Gilday, T. Lang, A. Caballero, P. J. Costa, V. Flix and P. D. Beer, Angew. Chem., Int. Ed., 2013, 52, 4356 CrossRef CAS PubMed.
- K. Sonogashira, Y. Tohda and N. Hagihara, Tetrahedron Lett., 1975, 16, 4467 CrossRef.
-
(a) H. Benesi and J. H. Hildebrand, J. Am. Chem. Soc., 1949, 71, 2703–2707 CrossRef CAS;
(b)
K. A. Connors, Binding constants: the measurement of molecular complex stability, Wiley, New York, 1987 Search PubMed.
- The preparation and characterization of LaL1 are available in the ESI.†.
- J.-C. G. Bünzli and G.-O. Pradervand, J. Chem. Phys., 1986, 85, 2489 CrossRef.
- The synthesis and characterization of the negative control compounds (EuL2, EuL3, L4 and L5) are available in the ESI.†.
Footnote |
† Electronic supplementary information (ESI) available: Detailed experimental procedures, characterization of compounds, NMR spectra and supplementary fluorometric titration studies. See DOI: 10.1039/c5sc04091d |
|
This journal is © The Royal Society of Chemistry 2016 |
Click here to see how this site uses Cookies. View our privacy policy here.