DOI:
10.1039/C5SC04133C
(Edge Article)
Chem. Sci., 2016,
7, 3737-3741
Redirecting immunity via covalently incorporated immunogenic sialic acid on the tumor cell surface†
Received
31st October 2015
, Accepted 23rd February 2016
First published on 24th February 2016
Abstract
Techniques eliciting anti-tumor immunity are of interest for immunotherapy. We herein report the covalent incorporation of a non-self immunogen into the tumor glycocalyx by metabolic oligosaccharide engineering with 2,4-dinitrophenylated sialic acid (DNPSia). This enables marked suppression of pulmonary metastasis and subcutaneous tumor growth of B16F10 melanoma cells in mice preimmunized to produce anti-DNP antibodies. Located on the exterior glycocalyx, DNPSia is well-positioned to recruit antibodies. Given the high levels of natural anti-DNP antibodies in humans and ubiquitous sialylation across many cancers, DNPSia offers a simplified route to redirect immunity against diverse tumors without recourse to preimmunization.
Introduction
Immune systems eradicate deleterious “non-self” cells while saving “self” cells by sensing cell surface biomarkers. As cancers evade the immune system surveillance, extensive effort has been devoted to redirect immunity against tumors by targeting biomarkers that are often nonimmunogenic. For instance, cytotoxic T cells genetically engineered with chimeric antigen receptors have been actively explored for targeting tumor surface antigens.1 However, challenges remain with these self-antigen targeted therapies owing to the presence of self-antigens on normal tissues and the inability to turn off persistent T cell activity.2 Alternatively, ligand–antigen adaptor molecules that bind avidly to tumor surface receptors (e.g. the folate receptor) enable exogenous antigens displayed on tumors to trigger immune responses.3 Albeit powerful, these approaches rely on tumor-specific high affinity receptors that are often not defined in many cancers.
Mammalian cells are covered with a dense layer of glycans, known as glycocalyx, which mediates diverse cellular events, such as immunological recognition and cancer metastasis.4 Sialic acids (Sia) are a family of 9-carbon monosaccharides commonly located at the cell surface glycan termini.5 Hypersialylation contributes to the metastatic potentials of many cancers,6 and facilitates tumor evasion of the immune system surveillance.7 Cell surface sialosides have been engineered with exogenous N-acyl mannosamines, metabolic precursors of Sia.8 However, the oligosaccharide engineering approach using peracetylated sugars is of low cell type- or tissue-specificity, leading to expression of N-acyl Sia in diverse tissues in animals.9 We recently observed a marked propensity of tumors to take up Sia derivatives with selected substitutions at C-9 in mice.10 We herein report covalent incorporation of a non-self immunogen into tumor glycocalyx with DNP-conjugated Sia (DNPSia) to redirect immunity against tumors. DNPSia effectively accumulates in tumors and is covalently installed into the cell surface glycocalyx by an endogenous sialylation pathway. Mice preimmunized with DNP-labelled keyhole limpet hemocyanin (DNPKLH) display marked antitumor effects against DNPSia-displaying B16F10 murine melanoma cells. Complementing receptor–ligand affinity based tumor coatings,3 this work suggests an alternative approach for tumor therapy via a metabolically incorporated non-self antigen (Scheme 1).
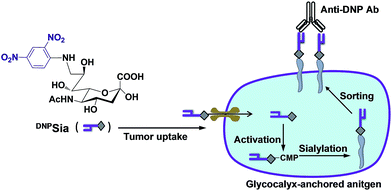 |
| Scheme 1 Schematic for the incorporation of the non-self antigen into glycocalyx. DNPSia taken up by the tumors is metabolically transferred to glycoconjugates. The neosialoconjugates sorted to the cell surface enable DNPSia to be well-positioned to trigger immunity. | |
Results and discussion
Metabolic incorporation of DNPSia on the cell surface glycocalyx
Sia chemically modified with C-9 substitutions are often compatible with cellular sialylation, leading to the incorporation of abiotic Sia into glycoconjugates. For instance, FITC-labelled Sia sialylates proteins in permeabilized CHO cells.11 Sia bearing an aromatic azide can be effectively incorporated into CD22 on the B cell surface.12 We recently observed that Sia with hydrophobic groups at C-9 preferentially accumulates in tumors in mice.10 Encouraged by these observations, we explored the efficacy of DNPSia incorporated on glycocalyx for tumor suppression.
We first probed the impact of the spacers of DNP-Sia diads on the cell surface sialoside expression. DNPSia featuring an amino spacer was synthesized from the nucleophilic aromatic substitution of 2,4-dinitrofluorobenzene with 9-amino-Sia whereas DNP–TzSia bearing a 1,2,3-triazole (Tz) linker was synthesized by the copper(I)-catalyzed azide–alkyne cycloaddition of 9-azido-Sia with 2,4-dinitro-1-propargylamino-benzene (Fig. 1A, Scheme S1 and S2, ESI†). B16F10 cells, poised to hypersialylation,13 were cultivated in Dulbecco's modified Eagle medium (DMEM) spiked with the methyl esters of DNP–TzSia or DNPSia (Scheme S1 and S2, ESI†), and then stained with biotin-labelled anti-DNP antibodies (Ab) and phycoerythrin (PE)-labelled streptavidin to probe the degrees of cell surface DNP. Confocal microscopic images reveal bright PE fluorescence confined to the plasma membranes of the cells treated with the methyl esters of DNPSia or DNP–TzSia, whereas no signal is observed in the control cells (Fig. 1B). Flow cytometry analysis revealed a 4-fold enhancement of cell surface DNPSia (MF = 7221) compared to DNP–TzSia (MF = 1686) (Fig. 1C). Western blotting confirms the high abundance of DNPSia-bearing proteins over DNP–TzSia-bearing proteins (Fig. 1D). These results validate the covalent incorporation of DNPSia into the glycocalyx with superior efficacy relative to DNP–TzSia. DNPSia was also effectively installed on the cell surface of Raw 264.7 macrophages, HeLa cells, L929, SMMC-7721 and U87-MG cells (Fig. S1, ESI†), demonstrating the compatibility of DNPSia with sialylation pathways of diverse cancer cell lines. The immunostaining of cell surface DNP shows that glycocalyx-anchored DNPSia is well-positioned to recruit anti-DNP Ab. We then monitored the temporal changes of cell surface DNPSia on B16F10 cells cultivated in fresh DMEM. Albeit decaying over time, the levels of glycocalyx-anchored DNPSia remained high after 24 h incubation (Fig. S2, ESI†). In addition, cells surface DNPSia was shown to be more resistant to sialidase-mediated hydrolysis relative to Sia (Fig. S3, ESI†), which is beneficial for in vivo immunotherapy.
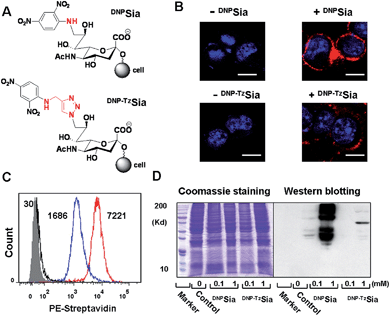 |
| Fig. 1 Incorporation of DNP-Sia diads into cell glycocalyx. Chemical structures of sialosides of DNPSia and DNP–TzSia displayed on a cell surface (A). B16F10 cells treated with the methyl esters of DNP–TzSia or DNPSia (0, 1 mM) were stained with biotin-labelled anti-DNP Ab, PE-labelled streptavidin, and DAPI specific for the nucleus, and then analysed by confocal fluorescence microscopy (B) or flow cytometry (C) with the mean channel fluorescence (MF) indicated. Bars: 10 μm. (D) Western blot of the lysate of cells treated with the DNP-Sia diads. Protein loading was verified by Coomassie Blue staining. | |
Anti-tumor effects of glycocalyx-anchored DNPSia in mice
Next, DNPSia was assessed for its influence on tumor cell proliferation in vitro. B16F10 cells pretreated without or with DNPSia were maintained in fresh DMEM for 24–72 h. No detrimental effects of DNPSia were observed on cell growth (Fig. 2A and S4, ESI†). With the negligible effects on cell growth in vitro, DNPSia was then explored for its capability to redirect immunity in vivo. C57BL/6 mice were immunized with DNPKLH and subsequently boosted with another injection. An enzyme-linked immunosorbent assay (ELISA) shows that the level of anti-DNP Ab in the serum from treated mice is 5-fold higher than in the untreated mice (Fig. 2B), proving induction of DNP-specific Ab by injected DNPKLH. DNPSia-displaying B16F10 cells (DNPSia+) and cells devoid of DNPSia (DNPSia−) were subcutaneously inoculated into the left or right flank of DNPKLH-treated mice, respectively. The tumor from the DNPSia+ cells exhibits about 75% volume reduction compared to that from the DNPSia− tumor 7 days after inoculation, whereas the tumor formation of the DNPSia− cells with natural Sia was largely unaffected in DNP-immunized mice relative to unimmunized mice (Fig. 2C). In unimmunized mice, the tumor from DNPSia+ cells was about 50% smaller relative to that from the DNPSia− cells, whereby retarded growth of the DNPSia+ tumor is likely due to endogenous low levels of anti-DNP Ab (Fig. 2B). In addition, time course monitoring reveals consistent and obvious suppression of tumor formation from implanted DNPSia+ cells over DNPSia− cells in DNPKLH-immunized mice (Fig. 2D) in the early stage, demonstrating synergistic effects of anti-DNP Ab and DNPSia on the anti-tumor response.
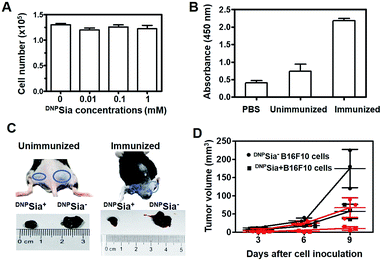 |
| Fig. 2
DNPSia mediated anti-tumor responses in mice. (A) Effects of DNPSia on cell proliferation. B16F10 cells pretreated with DNPSia (0–1 mM) were cultured in fresh DMEM for 24 h prior to cell number determination. (B) ELISA of anti-DNP Ab in the serum from C57BL/6 mice untreated or treated with an injection of DNPKLH. (C) Suppressed growth of inoculated DNPSia+ B16F10 cells over DNPSia-free cells that are injected in opposite flanks of mice. Tumors were excised 7 days post inoculation. (D) Anti-DNP Ab mediated inhibition of DNPSia+ B16F10 cells and DNPSia− cells subcutaneously inoculated in mice unimmunized (in dark) or immunized with DNPKLH (in red). Assays were performed in triplicate each using 3 mice. Error bars represent ±SD of experimental data on a representative assay. | |
To further evaluate the therapeutic scope of this approach, DNPKLH-immunized mice subcutaneously inoculated with B16F10 cells were treated with a tail-vein injection of PBS or DNPSia after tumor transplantation. DNPSia treatment resulted in a 50–90% reduction in the tumor volume in the early stage (6–9 days after cell inoculation) compared to the control mice treated with PBS (Fig. 3), proving the effectiveness of DNPSia for systemic tumor suppression.
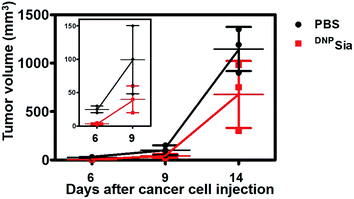 |
| Fig. 3 Tumor suppression with intravenously injected DNPSia in mice. DNPKLH-immunized C57BL/6 mice were subcutaneously inoculated with B16F10 cells, and then treated with a tail-vein injection of PBS (100 μL) or DNPSia (30 mg kg−1) on the 3rd, 6th, and 9th day post cell inoculation. The tumor volumes were monitored over time. The inset shows the correlation of early stage tumor volume vs. time. The assays were performed in triplicate, using 3 mice each time. The error bars represent ±SD of the experimental data on a representative assay. | |
Metastasis is a major cause of cancer-associated mortality. Cell surface sialosides are critical for cancer metastasis, promoting the use of sialyltransferase inhibitors to decrease cancer sialylation.14 We therefore evaluated the effects of DNPSia on B16F10 metastasis with an experimental pulmonary metastasis model. DNPSia+ B16F10 cells were injected into DNPKLH-immunized mice via the tail-vein. Lungs and representative organs were isolated 7 days after cell administration. The metastasis in the lung from mice treated with DNPSia+ cells is significantly smaller than that from mice treated with DNPSia− cells (Fig. 4 and S5, ESI†), revealing the capability of DNPSia to inhibit metastasis. Our results suggest an alternative approach against metastasis with chemically modified Sia on the cell surface.
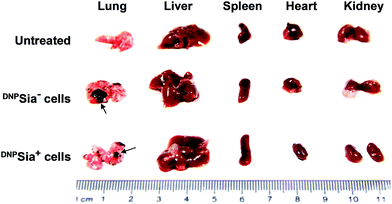 |
| Fig. 4 Glycocalyx-anchored DNPSia decreases pulmonary metastasis of B16F10 cells in mice. DNPKLH-immunized C57BL/6 mice were untreated or treated with DNPSia−, or DNPSia+ B16F10 cells by tail-vein injection. The organs were excised 7 days after injection. The arrows denote metastases. | |
Compared with affinity based tumor decoration,3b,d,15 we employ antigens covalently installed on glycocalyx via a metabolic sialylation pathway to elicit antitumor responses. Historically, sialosides with selected N-acyl groups at C-5 have been reported by Guo's group and Bertozzi's group to be more immunogenic than natural sialoside.16 The feasibility of this approach has been examined in both cell and mice models with exogenous N-acyl mannosamines, metabolic precursors of C-5-N-acyl Sia.16e,17 This abiotic Sia-mediated cancer therapy entails tandem preimmunizations to elicit Ab specific to the abiotic sialosides, and subsequent tumor expression of the abiotic Sia. These studies lay the foundation for unnatural Sia mediated immunotherapy. One percent of circulating antibodies in the human body binds DNP.18 Of note, our approach directly uses DNP-bearing Sia for metabolic tumor engineering, and offers simplified immunotherapy by recruiting high levels of natural anti-DNP Ab in humans without recourse to preimmunization.
Biodistribution of DNPSia in tumor-bearing mice
To probe the DNPSia biodistribution, C57BL/6 mice bearing subcutaneous B16F10 tumors were treated with DNPSia by tail-vein injection. The tumors and selected organs were excised 1 h post-injection and examined for DNPSia expression. Immunostaining reveals intense fluorescence in the tumors whereas negligible to moderate fluorescence is present in the kidney, heart, spleen, lung and liver (Fig. 5), showing that DNPSia is preferentially taken up and incorporated into glycoconjugates by tumors in mice. DNPSia displayed compromised tumor accumulation as the tumor volume increases (data not shown). A time course study shows that the tumor-associated DNPSia decreased over time and yet remained substantial 24 h post-injection (Fig. 6). By contrast, DNPSia incorporated in the heart and liver decreased to baseline levels 4 h after sugar administration (Fig. S6, ESI†), suggesting in vivo clearance of non-self antigens, which offers a means to temporarily turn off immunity and thus is beneficial for decreased systemic toxicity after treatment. Although B16F10 is a cell line featuring oversialylation,13 the elevated expression of the tumor surface DNPSia sialoside might also benefit from rapid cell division and glycoprotein biosynthesis in tumor cells relative to normal tissue and organs. The lower levels of DNPSia in heart tissue could trigger off-target immune responses. In the future, the selective expression of DNPSia on tumors could be potentiated with the aid of emerging vectors for the tumor-specific delivery of Sia.14b,19
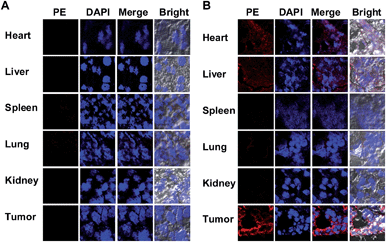 |
| Fig. 5
In vivo distribution of DNPSia. C57BL/6 mice bearing subcutaneous B16F10 tumors were injected via the tail-vein with PBS (A), or DNPSia (60 mg kg−1) (B). The tumors (10 mm3) and organs were excised 1 h post-injection, sectioned, and stained with biotin-labelled anti-DNP Ab, PE-labelled streptavidin and DAPI prior to fluorescence analysis. | |
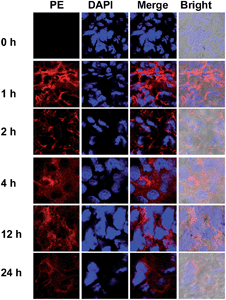 |
| Fig. 6 Temporal retention of DNPSia in tumors. C57BL/6 mice with B16F10 tumors (10 mm3) were injected with DNPSia (60 mg kg−1) in the tail-vein. At 0–24 h after the injection, the tumors were excised, sectioned, and stained with biotin-labelled anti-DNP Ab, PE-labelled streptavidin, and DAPI prior to fluorescence detection. | |
Cytotoxicity of DNPSia
Low toxicity is critical for agents to be administered in vivo. To probe the systemic toxicity, DNPSia was injected into healthy mice by the tail-vein at a dose of 300 mg kg−1, which is 10-fold higher than the dose used for the systemic tumor suppression mentioned in Fig. 3. No signs of abnormal behavior or death were observed in the mice up to 14 days after injection. Histological analysis reveals that the morphologies of the organs from mice untreated or treated with DNPSia were virtually identical (Fig. S7, ESI†), indicating that DNPSia is of low systemic toxicity.
Conclusions
We have demonstrated the use of DNPSia, a non-self immunogen tagged monosaccharide, to trigger immunity against tumors in mice. DNPSia is preferentially taken up by tumors and then metabolically incorporated into the cell surface, enabling marked suppression of pulmonary metastasis of DNPSia-bearing B16F10 melanoma cells and suppression of subcutaneous tumor formation by intravenously injected DNPSia in DNPKLH-immunized mice. Given the high levels of natural anti-DNP antibodies in humans,18aDNPSia on the outmost glycocalyx is well-positioned to recruit pre-existing antibodies and might offer a simplified immunotherapy in humans without recourse to preimmunization. Compared to ligand–receptor affinity mediated tumor targeting,3 our approach takes advantage of the widespread cellular sialylation pathway to covalently install non-self antigen conjugated Sia on the tumor glycocalyx, which, in principle, could increase the immunogenicity of diverse tumors with a broad range of immunogens.
Acknowledgements
This work was supported by grants from NSF China (21572189, 21272196), the Fundamental Research Funds for the Central Universities (20720160052, 20720150047), the 973 program 2013CB933901, PCSIRT, and open project grants from the State Key Laboratory of Cellular Stress Biology, Xiamen University. Dr J. Han was supported by grants from NSF China (91429301, 31420103910, 31330047, 31221065), the National Scientific and Technological Major Project (2013ZX10002-002), the Hi-Tech Research and Development Program of China (863 program; 2012AA02A201), the 111 Project (B12001), the Science and Technology Foundation of Xiamen (3502Z20130027), the National Science Foundation of China for Fostering Talents in Basic Research (J1310027) and the Open Research Fund of State Key Laboratory of Cellular Stress Biology, Xiamen University.
Notes and references
-
(a) G. Lipowska-Bhalla, D. E. Gilham, R. E. Hawkins and D. G. Rothwell, Cancer Immunol. Immunother., 2012, 61, 953 CrossRef CAS PubMed;
(b) E. J. Cheadle, H. Gornall, V. Baldan, V. Hanson, R. E. Hawkins and D. E. Gilham, Immunol. Rev., 2014, 257, 91 CrossRef PubMed;
(c) C. A. Klebanoff, T. N. Yamamoto and N. P. Restifo, Nat. Rev. Clin. Oncol., 2014, 11, 685 CrossRef CAS PubMed.
-
(a) D. M. Barrett, N. Singh, D. L. Porter, S. A. Grupp and C. H. June, Annu. Rev. Med., 2014, 65, 333 CrossRef CAS PubMed;
(b) G. P. Linette, E. A. Stadtmauer, M. V. Maus, A. P. Rapoport, B. L. Levine, L. Emery, L. Litzky, A. Bagg, B. M. Carreno, P. J. Cimino, G. K. Binder-Scholl, D. P. Smethurst, A. B. Gerry, N. J. Pumphrey, A. D. Bennett, J. E. Brewer, J. Dukes, J. Harper, H. K. Tayton-Martin, B. K. Jakobsen, N. J. Hassan, M. Kalos and C. H. June, Blood, 2013, 122, 863 CrossRef CAS PubMed;
(c) G. L. Beatty, A. R. Haas, M. V. Maus, D. A. Torigian, M. C. Soulen, G. Plesa, A. Chew, Y. Zhao, B. L. Levine, S. M. Albelda, M. Kalos and C. H. June, Cancer Immunol. Res., 2014, 2, 112 CrossRef CAS PubMed;
(d) C. H. Lamers, S. Sleijfer, S. van Steenbergen, P. van Elzakker, B. van Krimpen, C. Groot, A. Vulto, M. den Bakker, E. Oosterwijk, R. Debets and J. W. Gratama, Mol. Ther., 2013, 21, 904 CrossRef CAS PubMed;
(e) R. A. Morgan, J. C. Yang, M. Kitano, M. E. Dudley, C. M. Laurencot and S. A. Rosenberg, Mol. Ther., 2010, 18, 843 CrossRef CAS PubMed.
-
(a) C. B. Carlson, P. Mowery, R. M. Owen, E. C. Dykhuizen and L. L. Kiessling, ACS Chem. Biol., 2007, 2, 119 CrossRef CAS PubMed;
(b) C. E. Jakobsche, C. G. Parker, R. N. Tao, M. D. Kolesnikova, E. F. Douglass Jr. and D. A. Spiegel, ACS Chem. Biol., 2013, 8, 2404 CrossRef CAS PubMed;
(c) C. Bertozzi and M. Bednarski, Carbohydr. Res., 1992, 223, 243 CrossRef CAS PubMed;
(d) R. P. Murelli, A. X. Zhang, J. Michel, W. L. Jorgensen and D. A. Spiegel, J. Am. Chem. Soc., 2009, 131, 17090 CrossRef CAS PubMed;
(e) K. M. Shokat and P. G. Schultz, J. Am. Chem. Soc., 1991, 113, 1861 CrossRef CAS;
(f) M. K. O'Reilly, B. E. Collins, S. Han, L. Liao, C. Rillahan, P. I. Kitov, D. R. Bundle and J. C. Paulson, J. Am. Chem. Soc., 2008, 130, 7736 CrossRef PubMed;
(g) L. Cui, P. I. Kitov, G. C. Completo, J. C. Paulson and D. R. Bundle, Bioconjugate Chem., 2011, 22, 546 CrossRef CAS PubMed.
-
(a) M. Fukuda, Cancer Res., 1996, 56, 2237 CAS;
(b) S. Hakomori, Curr. Opin. Immunol., 1991, 3, 646 CrossRef CAS PubMed;
(c) P. R. Crocker, Curr. Opin. Struct. Biol., 2002, 12, 609 CrossRef CAS PubMed.
- T. Angata and A. Varki, Chem. Rev., 2002, 102, 439 CrossRef CAS PubMed.
-
(a) R. S. Bresalier, S. B. Ho, H. L. Schoeppner, Y. S. Kim, M. H. Sleisenger, P. Brodt and J. C. Byrd, Gastroenterology, 1996, 110, 1354 CrossRef CAS PubMed;
(b) G. Yogeeswaran and P. L. Salk, Science, 1981, 212, 1514 CrossRef CAS PubMed;
(c) R. Kannagi, M. Izawa, T. Koike, K. Miyazaki and N. Kimura, Cancer Sci., 2004, 95, 377 CrossRef CAS PubMed.
-
(a) J. E. Hudak, S. M. Canham and C. R. Bertozzi, Nat. Chem. Biol., 2014, 10, 69 CrossRef CAS PubMed;
(b) M. S. Macauley and J. C. Paulson, Nat. Chem. Biol., 2014, 10, 7 CrossRef CAS PubMed.
-
(a) S. J. Luchansky, S. Goon and C. R. Bertozzi, ChemBioChem, 2004, 5, 371 CrossRef CAS PubMed;
(b) C. Oetke, R. Brossmer, L. R. Mantey, S. Hinderlich, R. Isecke, W. Reutter, O. T. Keppler and M. Pawlita, J. Biol. Chem., 2002, 277, 6688 CrossRef CAS PubMed;
(c) C. Oetke, S. Hinderlich, R. Brossmer, W. Reutter, M. Pawlita and O. T. Keppler, Eur. J. Biochem., 2001, 268, 4553 CrossRef CAS PubMed;
(d) L. K. Mahal, K. J. Yarema and C. R. Bertozzi, Science, 1997, 276, 1125 CrossRef CAS PubMed;
(e) E. Saxon and C. R. Bertozzi, Science, 2000, 287, 2007 CrossRef CAS PubMed.
-
(a) H. Kayser, R. Zeitler, C. Kannicht, D. Grunow, R. Nuck and W. Reutter, J. Biol. Chem., 1992, 267, 16934 CAS;
(b) J. A. Prescher, D. H. Dube and C. R. Bertozzi, Nature, 2004, 430, 873 CrossRef CAS PubMed;
(c) A. A. Neves, H. Stockmann, R. R. Harmston, H. J. Pryor, I. S. Alam, H. Ireland-Zecchini, D. Y. Lewis, S. K. Lyons, F. J. Leeper and K. M. Brindle, FASEB J., 2011, 25, 2528 CrossRef CAS PubMed.
-
(a) X. Wu, Y. Tian, B. Lin, J. Han and S. Han, Biomater. Sci., 2014, 2, 1120 RSC;
(b) X. Wu, B. Lin, M. Yu, H. Xing, J. Han and S. Han, Chem. Sci., 2015, 6, 798 RSC.
-
(a) H. J. Gross and R. Brossmer, Eur. J. Biochem., 1988, 177, 583 CrossRef CAS PubMed;
(b) H. J. Gross, Eur. J. Biochem., 1992, 203, 269 CrossRef CAS PubMed.
- S. Han, B. E. Collins, P. Bengtson and J. C. Paulson, Nat. Chem. Biol., 2005, 1, 93 CrossRef CAS PubMed.
- Y. Kinoshita, S. Sato and T. Takeuchi, Cell Struct. Funct., 1989, 14, 35 CrossRef CAS PubMed.
-
(a) C. D. Rillahan, A. Antonopoulos, C. T. Lefort, R. Sonon, P. Azadi, K. Ley, A. Dell, S. M. Haslam and J. C. Paulson, Nat. Chem. Biol., 2012, 8, 661 CrossRef CAS PubMed;
(b) C. Bull, T. J. Boltje, E. A. van Dinther, T. Peters, A. M. de Graaf, J. H. Leusen, M. Kreutz, C. G. Figdor, M. H. den Brok and G. J. Adema, ACS Nano, 2015, 9, 733 CrossRef PubMed;
(c) C. Bull, T. J. Boltje, M. Wassink, A. M. de Graaf, F. L. van Delft, M. H. den Brok and G. J. Adema, Mol. Cancer Ther., 2013, 12, 1935 CrossRef PubMed.
-
(a) C. G. Parker, R. A. Domaoal, K. S. Anderson and D. A. Spiegel, J. Am. Chem. Soc., 2009, 131, 16392 CrossRef CAS PubMed;
(b) J. M. Fura, M. J. Sabulski and M. M. Pires, ACS Chem. Biol., 2014, 9, 1480 CrossRef CAS PubMed.
-
(a) Q. Wang, J. Zhang and Z. Guo, Bioorg. Med. Chem., 2007, 15, 7561 CrossRef CAS PubMed;
(b) P. Chefalo, Y. Pan, N. Nagy, Z. Guo and C. V. Harding, Biochemistry, 2006, 45, 3733 CrossRef CAS PubMed;
(c) G. A. Lemieux and C. R. Bertozzi, Chem. Biol., 2001, 8, 265 CrossRef CAS PubMed;
(d) L. M. Krug, G. Ragupathi, K. K. Ng, C. Hood, H. J. Jennings, Z. Guo, M. G. Kris, V. Miller, B. Pizzo, L. Tyson, V. Baez and P. O. Livingston, Clin. Cancer Res., 2004, 10, 916 CrossRef CAS PubMed;
(e) T. Liu, Z. Guo, Q. Yang, S. Sad and H. J. Jennings, J. Biol. Chem., 2000, 275, 32832 CrossRef CAS PubMed.
- W. Zou, S. Borrelli, M. Gilbert, T. Liu, R. A. Pon and H. J. Jennings, J. Biol. Chem., 2004, 279, 25390 CrossRef CAS PubMed.
-
(a) F. S. Farah, Immunology, 1973, 25, 217 CAS;
(b) K. Karjalainen and O. Makela, Eur. J. Immunol., 1976, 6, 88 CrossRef CAS PubMed.
- R. Xie, S. Hong, L. Feng, J. Rong and X. Chen, J. Am. Chem. Soc., 2012, 134, 9914 CrossRef CAS PubMed.
Footnotes |
† Electronic supplementary information (ESI) available: The synthesis of DNP conjugated sialic acids, western blot analysis, treatment and analysis of sugar-treated cells and mice. See DOI: 10.1039/c5sc04133c |
‡ Both authors contributed equally to this work. |
|
This journal is © The Royal Society of Chemistry 2016 |
Click here to see how this site uses Cookies. View our privacy policy here.