DOI:
10.1039/C5SC04403K
(Edge Article)
Chem. Sci., 2016,
7, 3689-3693
Supramolecular reactions of metallo-architectures: Ag2-double-helicate/Zn4-grid, Pb4-grid/Zn4-grid interconversions, and Ag2-double-helicate fusion†
Received
17th November 2015
, Accepted 3rd February 2016
First published on 29th February 2016
Abstract
Supramolecular reactions are of importance in many fields. We report herein three examples where complexes of hydrazone-based ligands are involved. A Ag2-double-helicate was converted, by treatment with Zn(OTf)2, into a Zn4-grid (exchange of metal ions and change of the nature of the initial complex). A Pb4-grid was converted, upon reaction with ZnCl2 or ZnBr2, into a Zn4-grid (exchange of metal ions, but conservation of the nature of the initial complex). The reverse conversions were also achieved. The fusion of a Ag2-double-helicate with another Ag2-double-helicate was performed (exchange of ligands, but conservation of the nature of the complexes) and resulted in a mixture of three helicates (two homostranded ones and one heterostranded one).
Introduction
Like covalent molecules, supramolecular1 assemblies may participate in various reactions. The understanding of supramolecular reactions is of much interest because they are involved in many areas such as complex chemical systems and networks,2 adaptive3 and stimuli-responsive4 chemical systems, fabrication of nanodevices and materials,4 biomolecular processes. Thus, in the complexity and diversity of supramolecular chemistry, the reactivity of supramolecules plays a crucial role. It includes the processes:
(a) of (self)assembly (i.e. formation of supramolecular architectures through assembly, but also their participation, as subunits, in more complex assemblies), and correlatively, partial or total disassembly;
(b) of partial or total reorganization or exchange (at the supramolecular and, additionally and possibly, at the covalent level), that involves the breaking of several or all of the initial supramolecular connections and formation of new ones;
(c) without breaking or formation of new supramolecular connections (e.g. covalent modifications after self-assembly5).
Amongst supramolecular architectures, double helices and helicates,6 as well as grids7 arouse much interest and work. For example, DNA8 and the ion channel generated by gramicidine9 have a double helical structure, and there are double helical complexes that act as molecular machines10 or catalysts.11 Grid-like complexes have been studied for their electrochemical and magnetic properties,7 for their capacity to encapsulate ions12 or as starting materials for building more complex architectures (e.g. a Solomon link13), amongst other things. However, supramolecular interconversions of grids and helicates have not, except several examples,14,15 been much explored.
With these ideas in mind – and using principles such as the displacement of an equilibrium through precipitation, and the preference of Ag+ for tetrahedral and of Zn2+ for octahedral coordination – we designed, as reported herein, three supramolecular reactions16 of reorganization and exchange (Fig. 1) involving grids and double helicates. They are related through the ligands17 (which are pyrimidine-bis-hydrazones;18Fig. 2) that produce the supramolecular complexes, as well as through the nature of complexes, and occur due to the dynamic character of the present metal–ligand connections. These reactions (Fig. 1) can be seen as:
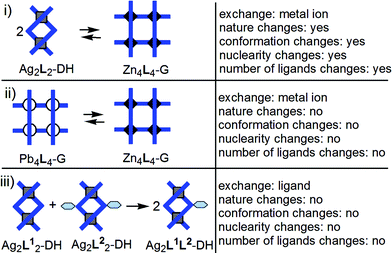 |
| Fig. 1 Stylized representation of the three types of supramolecular reactions reported herein. | |
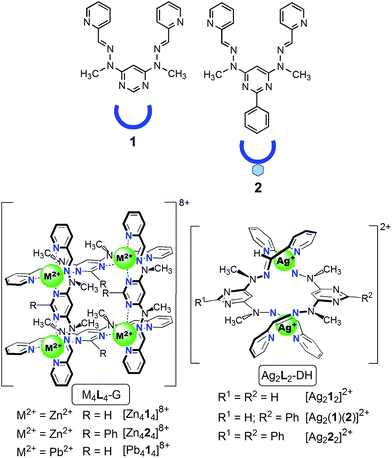 |
| Fig. 2 Structural formulae and stylized representations of ligands 1 and 2, and of grids and double helicates. | |
(i) a change of the nature of the supramolecular architecture, from a Ag+ dinuclear double helicate (DH) into a Zn2+ tetranuclear grid (G), induced by replacement of Ag+ by Zn2+ (Fig. 3). In this reaction, not only the nature of the complex and that of the metal ion change, but also the conformation of the ligand (helical → unfolded), the charge (2+ → 8+) and the nuclearity of the complex (2 → 4) and the number of ligands per complex (2 → 4). In regard to this last change, this process can be compared with the conversion or the equilibrium between supramolecular dimer and tetramer of bioactive proteins,19 or between other homo-oligomers20 with influence on the protein functions.
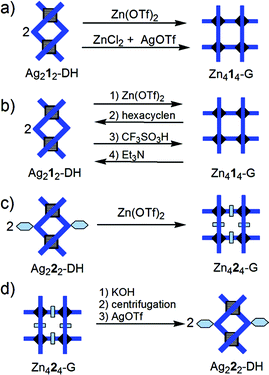 |
| Fig. 3 Stylized representation of: (a) the conversion of Ag212-DH double helicate into the Zn414-G grid; (b) the interconversion Ag212-DH/Zn414-G; (c) the conversion of Ag222-DH double helicate into the Zn424-G grid; (d) the conversion of Zn424-G grid into Ag222-DH double helicate. Charges and stoichiometric coefficients are omitted for simplicity. | |
(ii) a substitution21 (metal ion exchange or transmetallation), in a sole operation, of the four Pb2+ ions of a grid-like22 complex by Zn2+ ions (Fig. 4);
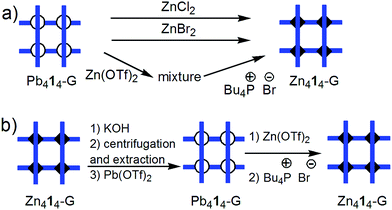 |
| Fig. 4 Stylized representation of: (a) reaction of Pb414-G grid with ZnCl2, ZnBr2, and Zn(OTf)2 and Bu4P+Br− (solvent CD3CN); (b) Zn414-G/Pb414-G and Pb414-G/Zn414-G conversions. Charges and stoichiometric coefficients are omitted for simplicity. | |
(iii) a fusion (conproportionation)23 between two Ag+ double helicates24 (Fig. 5).
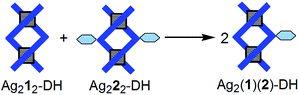 |
| Fig. 5 Stylized representation of the fusion (conproportionation) reaction of double helicates Ag212-DH and Ag222-DH with formation of heterostranded species Ag2(1)(2)-DH. Charges are omitted for simplicity. | |
While in case (ii) the equilibrium is shifted towards the Zn2+ grid through the precipitation of Pb2+ as its halides (chloride and bromide), in cases (i) and (iii), the conversions can be done without precipitation.
Results and discussion
(i) The conversion Ag2L2-DH → Zn4L4-G (L = 1, 2) through transmetallation is a dramatic reorganization of the nature of the metallo-supramolecular architecture induced by the replacement of Ag+ by Zn2+ (Fig. 3a and c): 2 Ag2L2-DH + 4 Zn2+ → Zn4L4-G + 4 Ag+. Ag+ prefers a tetrahedral coordination geometry which is, in the case of ligands 1 and 2, achieved from 2 two-Nsp2-atom bidentate pyridine-hydrazone sites. In this way, Ag+ induces the formation of double helicates with ligands 1 and 2. Zn2+ prefers an octahedral coordination environment that results from 2 three-Nsp2-atom tridentate sites of type pyridine-hydrazone-pyrimidine, thus generating a grid.
Reaction of 1 equiv. of Ag2L2-DH15c with 2 equiv. of Zn(OTf)2 (OTf− = CF3SO3−) produces – without the need to precipitate Ag+ as a halide – the corresponding grid Zn4L4-G15b,22a (solvent: CD3NO2 with 6–14% CD3CN; ESI, pp. S9–S11†). Where ZnCl2 is used in the reaction with Ag212-DH, two equivalents of AgOTf per equiv. of DH are required according to the equation (ESI p. S8†):
2Ag212-DH + 4ZnCl2 + 4Ag+ → Zn414-G + 8AgCl |
On treatment of the double helicate Ag222-DH in CD3NO2 with 2 equiv. of Zn(OTf)2 – added as a solution in a small volume of CD3CN, or as a solid – the grid Zn424-G was obtained. When the double helicate Ag212-DH in CD3NO2 was treated with 2 equiv. of Zn(OTf)2, added as a solution in a small volume of CD3CN (about 6–14% of the CD3NO2 volume), the grid Zn414-G was obtained. When Zn(OTf)2 was added as a solid, without CD3CN, was obtained a mixture without the Zn414-G grid; addition of a small volume of CH3CN (about 6–14% of the CD3NO2 volume) to this mixture produced the expected grid Zn414-G. A possible explanation could be that, in the case of the reaction Ag212-DH → Zn414-G, the CH3CN acts as a coordinating species for the Ag+ ions and so contributes to the displacement of the equilibrium from the double helix towards the grid. The grid Zn424-G should be – due to the π-stacking aromatic interaction between a phenyl ring and the two ligands between which that phenyl is located within the grid – more stable than the grid Zn414-G. This stability may be sufficient to make possible the formation of the grid Zn424-G from the corresponding double helicate without, unlike in the case of the grid Zn414-G, the assistance of CH3CN.
DOSY NMR was also used to study the conversion Ag2L2-DH → Zn4L4-G (L = 1, 2). As expected, the volume of the grid species obtained from double helicates on treatment with Zn(OTf)2 was found in agreement with that of the grid prepared from the free ligands L and Zn(OTf)2.
The reverse conversion Zn4L4-G→ Ag2L2-DH can be done as follows: after treatment of the grid with KOH, the solvent (CD3CN or CD3NO2) is removed, and the ligand is extracted with CDCl3 and separated from the solid residue (by centrifugation or filtration); after removal of CDCl3, CD3NO2 is added, then AgOTf is added to form the helicate. In order to simplify the procedure, we used ligand 2 and a mixture of CDCl3 and CD3NO2 where ligand 2, as well as the corresponding grid and double helicate were soluble. After precipitation of Zn2+ with KOH, the mixture was centrifuged (the ligand 2 being soluble in the mixture of solvents), and to the recovered liquid phase AgOTf was added to produce the Ag222-DH (ESI, p. S13†).
In a pH-dependent system (Fig. 3b), the interconversion between Ag212-DH and Zn414-G was achieved as follows (ESI, p. S10†): the grid was generated from the double helicate by reaction with Zn2+; then, Zn2+ was complexed with hexacyclen, and the double helicate was regenerated; partial protonation of hexacyclen with TfOH caused release of Zn2+ and formation of the grid (incomplete yield); finally, addition of triethylamine reactivated the hexacyclen that again encapsulated Zn2+ and resulted in the reformation of the double helicate.
(ii) The Pb414-G → Zn414-G conversion (Fig. 4a) can formally be seen as a substitution of Pb2+ by Zn2+ ions, although the real mechanism, involving breaking and formation of supramolecular bonds, must be more complex. Reaction of Pb414-G15b with 4 equiv. of Zn(OTf)2 produces a mixture which no longer contains the grid-like species Pb414-G or Zn414-G (ESI p. S2†). This suggests that the affinity of Zn2+ for the ligand, as well as its preference for octahedral coordination are not sufficient to displace the equilibrium towards Zn414-G. We considered that the involvement of Pb2+ ions in a weakly dissociating or sparingly soluble compound should displace the equilibrium. Indeed, addition of Br− (as Bu4P+Br−) to the above mixture, or treatment of Pb414-G with four equivalents of ZnBr2 or ZnCl2 produced – along with the formation of PbX2 (X = Br, Cl) which precipitates and, doing so, shifts the equilibrium – the expected Zn414-G grid (solvent: CD3CN; ESI pp. S3–S4†): Pb414-G + 4 ZnX2 → Zn414-G + 4 PbX2.
The reverse conversion Zn414-G → Pb414-G grid was achieved in several steps (Fig. 4b). Treatment of Zn414-G (in CD3CN) with KOH led to the precipitation of Zn2+ (as Zn(OH)2 or K2[Zn(OH)4]), as well as of the free ligand 1. After removal of CD3CN, the free ligand 1 was extracted with CDCl3 and used further for the preparation of Pb414-G (see ESI, p. S5†).
Thus, in addition to its self-assembly from Zn2+ and a ligand, the same Zn2+ grid, Zn414-G, can be obtained, in reactions (i) and (ii), from a Ag+ dinuclear double helicate or from a Pb2+ tetranuclear grid (exchange of metal ions and reorganization of the architectures).
(iii) The fusion (conproportionation) reaction of double helicate Ag212-DH15c with 1 equiv. of Ag222-DH (Fig. 5) according to the equation
Ag212-DH + Ag222-DH → 2Ag2(1)(2)-DH |
produces a mixture that contains each of the three helicates, namely two homoleptic (homostranded) ones and one heteroleptic (heterostranded) one. Ligands
1 and
2 equally participate to homo- and heteroleptic helicates, and so the observed molar percentages are of approximately 25% for Ag
212-DH, 25% for Ag
222-DH, and 50% for Ag
2(
1)(
2)-DH. For characterization of the new compound Ag
222-DH, see ESI pp. S14–S20;
† for
1H,
13C and DOSY of the mixture of three helicates, see ESI pp. S25–S31.
†
For the reactions described above it might appear necessary, in practice, to slightly (2–10%) increase the amounts of reagents with respect to those theoretically calculated.
Experimental
For experimental details, see the ESI.†
Conclusions
To summarize, three supramolecular reactions were investigated: (i) a Ag2L2-DH double-helicate into Zn4L4-G grid conversion, where the exchange of metal ions changes the nature of the metallo-supramolecular architecture, (ii) a Zn414-G grid into Pb414-G grid conversion driven by a halide-induced precipitation and where the nature of the metallo-supramolecular architecture is conserved, and (iii) a double exchange of ligands during the fusion of two double helicates.
The grid/grid and double-helicate/grid conversions were made reversible by precipitation of Zn2+ with KOH and subsequent reaction of the free ligand with Ag+ or Pb2+, or, for one DH/G interconversion, in a pH-dependent way.
In perspective, such ligands could be introduced in larger and more complex, suitably decorated, architectures where such supramolecular reactions can act as actuators of various properties (charge, volume, multivalency).
Acknowledgements
We thank Prof. Jack Harrowfield for helpful discussions, and Stéphanie Kouaho for mass spectrometry analyses.
Notes and references
- J.-M. Lehn, Angew. Chem., Int. Ed. Engl., 1988, 27, 89–121 CrossRef; J.-M. Lehn, Angew. Chem., Int. Ed. Engl., 1990, 29, 1304–1319 CrossRef.
- R. F. Ludlow and S. Otto, Chem. Soc. Rev., 2008, 37, 101–108 RSC.
- D. G. Kurth, Sci. Technol. Adv. Mater., 2008, 9, 014103 CrossRef PubMed.
- J.-M. Lehn, Angew. Chem., Int. Ed., 2013, 52, 2836–2850 CrossRef CAS PubMed.
- K. C.-F. Leung, C.-P. Chak, C.-M. Lo, W.-Y. Wong, S. Xuan and C. H. K. Cheng, Chem.–Asian J., 2009, 4, 364–381 CrossRef CAS PubMed; X. Yan, F. Wang, B. Zheng and F. Huang, Chem. Soc. Rev., 2012, 41, 6042–6065 RSC; A. J. McConnell, C. S. Wood, P. P. Neelakandan and J. R. Nitschke, Chem. Rev., 2015, 115, 7729–7793 CrossRef PubMed.
- See, for example: M. Wang, W.-J. Lan, Y.-R. Zheng, T. R. Cook, H. S. White and P. J. Stang, J. Am. Chem. Soc., 2011, 133, 10752–10755 CrossRef CAS PubMed.
- For a selection of reviews, see: C. Piguet, G. Bernardinelli and G. Hopfgartner, Chem. Rev., 1997, 97, 2005–2062 CrossRef CAS PubMed; C. Piguet, J. Inclusion Phenom. Macrocyclic Chem., 1999, 34, 361–391 CrossRef; M. Albrecht, Chem. Rev., 2001, 101, 3457–3497 CrossRef PubMed; M. J. Hannon and L. J. Childs, Supramol. Chem., 2004, 16, 7–22 CrossRef For reviews, see: M. Ruben, J. Rojo, F. J. Romero-Salguero, L. H. Uppadine and J.-M. Lehn, Angew. Chem., Int. Ed., 2004, 43, 3644–3662 CrossRef PubMed; L. N. Dawe, T. S. M. Abedin and L. K. Thompson, Dalton Trans., 2008, 1661–1675 RSC; L. N. Dawe, K. V. Shuvaev and L. K. Thompson, Chem. Soc. Rev., 2009, 38, 2334–2359 RSC; A.-M. Stadler, Eur. J. Inorg. Chem., 2009, 4751–4770 CrossRef; J. Hardy, Chem. Soc. Rev., 2013, 42, 7881–7899 RSC.
- J. D. Watson and F. H. Crick, Nature, 1953, 171, 737–738 CrossRef CAS PubMed.
- B. M. Burkhart, N. Li, D. A. Langs, W. A. Pangborn and W. L. Duax, Proc. Natl. Acad. Sci. U. S. A., 1998, 95, 12950–12955 CrossRef CAS.
- K. Miwa, Y. Furusho and E. Yashima, Nat. Chem., 2010, 2, 444–449 CrossRef CAS PubMed.
- C.-T. Yeung, L.-H. Yeung, C.-S. Tsang, W.-Y. Wong and H.-L. Kwong, Chem. Commun., 2007, 5203–5205 RSC.
- B. R. Manzano, F. A. Jalón, I. M. Ortiz, M. L. Soriano, F. Gómez de la Torre, J. Elguero, M. A. Maestro, K. Mereiter and T. D. W. Claridge, Inorg. Chem., 2008, 47, 413–428 CrossRef CAS PubMed.
- J. E. Beves, J. J. Danon, D. A. Leigh, J.-F. Lemonnier and I. J. Vitorica-Yrezabal, Angew. Chem., Int. Ed., 2015, 54, 7555–7559 CrossRef CAS PubMed.
- For assembly/disassembly of double helicates, see: J.-P. Gisselbrecht, M. Gross, J.-M. Lehn, J.-P. Sauvage, R. Ziessel, C. Piccinni-Leopardi, J. M. Arrieta, G. Germain and M. V. Meersche, Nouv. J. Chim., 1984, 8, 661–667 Search PubMed; Y. Yao, M. W. Perkovic, D. P. Rillema and C. Woods, Inorg. Chem., 1992, 31, 3956–3962 CrossRef CAS; K. T. Potts, M. Keshavarz-K, F. S. Tham, H. D. Abruña and C. R. Arana, Inorg. Chem., 1993, 32, 4422–4435 CrossRef; V. Amendola, L. Fabbrizzi, L. Linati, C. Mangano, P. Pallavicini, V. Pedrazzini and M. Zema, Chem.–Eur. J., 1999, 5, 3679–3688 CrossRef; V. Amendola, L. Fabbrizzi, L. Gianelli, C. Maggi, C. Mangano, P. Pallavicini and M. Zema, Inorg. Chem., 2001, 40, 3579–3587 CrossRef PubMed; V. Amendola, L. Fabbrizzi, P. Pallavicini, E. Sartirana and A. Taglietti, Inorg. Chem., 2003, 42, 1632–1636 CrossRef PubMed; M. Boiocchi and L. Fabbrizzi, Chem. Soc. Rev., 2014, 43, 1835–1847 RSC . See also: M. Barboiu, G. Vaughan, N. Kyritsakas and J.-M. Lehn, Chem.–Eur. J., 2003, 9, 763–769 CrossRef PubMed.
-
(a) Grid/double-cross/stick: M. Barboiu, G. Vaughan, R. Graff and J.-M. Lehn, J. Am. Chem. Soc., 2003, 34, 10257–10265 CrossRef PubMed;
(b) Grid/stick: A.-M. Stadler, N. Kyritsakas, R. Graff and J.-M. Lehn, Chem.–Eur. J, 2006, 12, 4503–4522 CrossRef CAS PubMed;
(c) Double helicate/stick: A.-M. Stadler, N. Kyritsakas, G. Vaughan and J.-M. Lehn, Chem.–Eur. J., 2007, 13, 59–68 CrossRef CAS PubMed;
(d) Grid/pincer: J. Ramirez, A. M. Stadler, N. Kyritsakas and J.-M. Lehn, Chem. Commun., 2007, 237–239 RSC;
(e) Pincer/stick: J. Ramirez, A.-M. Stadler, L. Brelot and J.-M. Lehn, Tetrahedron, 2008, 64, 8402–8410 CrossRef CAS;
(f) Double helicate/stick: D. J. Hutchinson, S. A. Cameron, L. R. Hanton and S. C. Moratti, Inorg. Chem., 2012, 51, 5070–5081 CrossRef CAS PubMed;
(g) Double-helicate/grid: A.-M. Stadler, C. Burg, J. Ramírez and J.-M. Lehn, Chem. Commun., 2013, 49, 5733–5735 RSC;
(h) see also: P. N. W. Baxter, R. G. Khoury, J.-M. Lehn, G. Baum and D. Fenske, Chem.–Eur. J., 2000, 6, 4140–4148 CrossRef CAS PubMed;
(i) C. S. Campos-Fernandez, B. L. Schottel, H. T. Chifotides, J. K. Bera, J. Bacsa, J. M. Koomen, D. H. Russell and K. R. Dunbar, J. Am. Chem. Soc., 2005, 127, 12909–12923 CrossRef CAS PubMed.
- For examples of supramolecular interconversions, see: D. P. Funeriu, J.-M. Lehn, K. M. Fromm and D. Fenske, Chem.–Eur. J., 2000, 6, 2103–2111 CrossRef CAS; K. Kumazawa, Y. Yamanoi, M. Yoshizawa, T. Kusukawa and M. Fujita, Angew. Chem., Int. Ed., 2004, 43, 5936–5940 CrossRef PubMed; A. M. Brown, M. V. Ovchinnikov, C. L. Stern and C. A. Mirkin, J. Am. Chem. Soc., 2004, 126, 14316–14317 CrossRef PubMed; O. Mamula, M. Lama, H. Stoeckli-Evans and S. Shova, Angew. Chem., Int. Ed., 2006, 45, 4940–4944 CrossRef PubMed; K. Harano, S. Hiraoka and M. Shionoya, J. Am. Chem. Soc., 2007, 129, 5300–5301 CrossRef PubMed; J. Heo, Y.-M. Jeon and C. A. Mirkin, J. Am. Chem. Soc., 2007, 129, 7712–7713 CrossRef PubMed; L. Zhao, B. H. Northrop and P. J. Stang, J. Am. Chem. Soc., 2008, 130, 11886–11888 CrossRef PubMed; S. Hiraoka, Y. Sakata and M. Shionoya, J. Am. Chem. Soc., 2008, 130, 10058–10059 CrossRef PubMed; H. Dong, J. Yang, X. Liu and S. Gou, Inorg. Chem., 2008, 47, 2913–2915 CrossRef PubMed; P. J. Lusby, P. Müller, S. J. Pike and A. M. Z. Slawin, J. Am. Chem. Soc., 2009, 131, 16398–16400 CrossRef PubMed; K. Parimal, E. H. Witlicki and A. H. Flood, Angew. Chem., Int. Ed., 2010, 49, 4628–4632 CrossRef PubMed; S. Chen, L.-J. Chen, H.-B. Yang, H. Tian and W. Zhu, J. Am. Chem. Soc., 2012, 134, 13596–13599 CrossRef PubMed; X. Yan, J.-F. Xu, T. R. Cook, F. Huang, Q.-Z. Yang, C.-H. Tung and P. J. Stang, Proc. Natl. Acad. Sci. U. S. A., 2014, 111, 8717–8722 CrossRef PubMed; X. Lu, X. Li, K. Guo, T.-Z. Xie, C. N. Moorefield, C. Wesdemiotis and G. R. Newkome, J. Am. Chem. Soc., 2014, 136, 18149–18155 CrossRef PubMed; Y.-F. Han, L. Zhang, L.-H. Weng and G.-X. Jin, J. Am. Chem. Soc., 2014, 136, 14608–14615 CrossRef PubMed; Y. Li, Z. Jiang, J. Yuan, D. Liu, T. Wu, C. N. Moorefield, G. R. Newkome and P. Wang, Chem. Commun., 2015, 51, 5766–5769 RSC; D. Preston, A. Fox-Charles, W. K. C. Lo and J. D. Crowley, Chem. Commun., 2015, 51, 9042–9045 RSC; M. Han, Y. Luo, B. Damaschke, L. Gýmez, X. Ribas, A. Jose, P. Peretzki, M. Seibt and G. H. Clever, Angew. Chem., Int. Ed., 2016, 55, 445–449 CrossRef PubMed.
- J.-L. Schmitt, A.-M. Stadler, N. Kyritsakas and J.-M. Lehn, Helv. Chim. Acta, 2003, 86, 1598–1624 CrossRef CAS.
- X. Su and I. Aprahamian, Chem. Soc. Rev., 2014, 43, 1963–1981 RSC.
- See for example the cases of
(a) spectrin: S.-C. Liu and J. Palek, Nature, 1980, 285, 586–588 CrossRef CAS PubMedE. Ungewickell and W. Gratzer, Eur. J. Biochem., 1978, 88, 379–385 CrossRef CAS PubMed;
(b) the clock protein KaiB: T. Iida, R. Mutoh, K. Onai, M. Morishita, Y. Furukawa, K. Namba and M. Ishiura, Genes Cells, 2015, 20, 173–190 CrossRef CAS PubMed;
(c) 6-phosphogluconate dehydrogenase, see: S. Hanau, L. Proietti d'Empaire, I. Capone, S. Alberighi, R. Montioli and F. Dallocchio, Biochim. Biophys. Acta, 2013, 1834, 2647–2652 CrossRef CAS PubMed;
(d) Bovine IF1, see: E. Cabezon, P. J. G. Butler, M. J. Runswick and J. E. Walker, J. Biol. Chem., 2000, 275, 25460–25464 CrossRef CAS PubMed;
(e) phosphofructokinase, see: J. Xu, T. Oshima and M. Yoshida, J. Mol. Biol., 1990, 215, 597–606 CrossRef CAS PubMed.
- For the case of
(a) uracil phosphoribosyltransferase from Escherichia coli, see: K. F. Jensen and B. Mygind, Eur. J. Biochem., 1996, 240, 637–645 CrossRef CAS PubMed;
(b) mushroom tyrosinase, see: R. L. Jolley Jr, D. A. Robb and H. S. Mason, J. Biol. Chem., 1969, 244, 1593–1599 CAS;
(c)
D-amino acid oxidase, see: E. Antonini, M. Brunori, R. Bruzzesi, E. Chiancone and V. Massey, J. Biol. Chem., 1966, 241, 2358–2366 CAS See also:
(d) E. K. Jaffe, Open Conf. Proc. J, 2010, 1, 1–6 CAST. Selwood and E. K. Jaffe, Arch. Biochem. Biophys., 2012, 519, 131–143 CrossRef CAS PubMed.
- For an example of supramolecular substitution reaction, see: J. Santamaría, T. Martín, G. Hilmersson, S. L. Craig and J. Rebek Jr, Proc. Natl. Acad. Sci. U. S. A., 1999, 96, 8344–8347 CrossRef.
- Pb2+ or Zn2+ hydrazone-based grids:
(a) M. Barboiu, M. Ruben, G. Blasen, N. Kyritsakas, E. Chacko, M. Dutta, O. Radekovich, K. Lenton, D. J. R. Brook and J.-M. Lehn, Eur. J. Inorg. Chem., 2006, 784–789 CrossRef CAS;
(b) A. R. Stefankiewicz, G. Rogez, J. Harrowfield, M. Drillon and J.-M. Lehn, Dalton Trans., 2009, 5787–5802 RSC;
(c) M. Dutta, M. Movassat, D. J. R. Brook, A. Oliver and D. Ward, Supramol. Chem., 2011, 23, 630–640 CrossRef;
(d) D. J. Hutchinson, L. R. Hanton and S. C. Moratti, Inorg. Chem., 2011, 50, 7637–7649 CrossRef CAS PubMed.
- M. L. Saha, S. Pramanik and M. Schmittel, Chem. Commun., 2012, 48, 9459–9461 RSC.
- For other examples of Ag+ hydrazone-based double helicates, see: D. J. Hutchinson, P. M. James, L. R. Hanton and S. C. Moratti, Inorg. Chem., 2014, 53, 2122–2132 CrossRef CAS PubMed; D. J. Hutchinson, L. R. Hanton and S. C. Moratti, Dalton Trans., 2014, 43, 8205–8218 RSC.
Footnote |
† Electronic supplementary information (ESI) available. See DOI: 10.1039/c5sc04403k |
|
This journal is © The Royal Society of Chemistry 2016 |
Click here to see how this site uses Cookies. View our privacy policy here.