DOI:
10.1039/C6RA26126D
(Paper)
RSC Adv., 2017,
7, 1229-1232
Direct vinylogous oxidative cross-dehydrogenative coupling of 4-nitroisoxazoles with N-aryl tetrahydroisoquinolines in water under air conditions†
Received
1st November 2016
, Accepted 19th December 2016
First published on 4th January 2017
Abstract
A direct vinylogous cross-dehydrogenative coupling (CDC) reaction of N-aryl tetrahydroisoquinolines with 3,5-dialkyl-4-nitroisoxazoles catalysed by CuBr using air as the oxidant in water has been developed. This new strategy provides an efficient and environmentally benign way to access medicinally important isoxazole substituted tetrahydroisoquinoline derivatives under mild conditions.
The development of novel and environmentally benign strategies for constructing C–C bonds is of great significance to organic chemists. In this respect, the direct catalytic oxidative cross-dehydrogenative coupling (CDC)1 of two C–H bonds, which avoids the prefunctionalization of substrates, is widely recognized as one of the most direct, atom economical and clean methods for the formation of new C–C bonds. Although various oxidants including inorganic metal salts and organic oxidants are applicable, the use of molecular oxygen is quite promising because water may be the only byproduct in these reactions.2 In particular, when water was used as the only solvent, these aerobic CDC reactions in water processes can completely meet the criteria of green chemistry.3 However, examples of aerobic CDC reactions in water are quite rare.4
On the other hand, selective C–C bond formation at a remote position is a major challenge in modern organic synthesis.5 With the exploitation of the vinylogy concept,6 the vinylogous reaction has emerged as an effective strategy to tackle this challenge.7 However, so far only two examples have recently been disclosed in the literature concerning the application of the vinylogy concept in CDC reaction. In 2011, Carcía Mancheño8 realised the first example of direct γ-alkylation of α,β-unsaturated aldehydes by isochromans using oxoammonium salt as the oxidant, albeit with poor yields and selectivities. Using silyloxyfurans as the Mukaiyama-type reagents, Doyle9 found that both rhodium and iron could catalyse the oxidative indirect vinylogous Mannich reaction of tertiary anilines. From the standpoint of atom economy, the development of a novel catalytic direct vinylogous CDC reaction to efficiently synthesise target molecular bearing scaffolds of complexity is still highly desirable.
Both tetrahydroisoquinolines (THIQs)10 and isoxazoles11 are heterocyclic privileged scaffolds in pharmaceutical and natural products with a broad spectrum of interesting biological activities respectively. The reasonable assembly of isoxazole and THIQ for the synthesis of isoxazole–THIQ conjugates may provide a new platform for drug discovery. As an extension of our continuous research efforts to develop vinylogous reactions,12 we described herein the first direct vinylogous CDC reactions of 3,5-dialkyl-4-nitroisoxazoles with N-aryl THIQs. This approach provides an easy access to highly functionalized isoxazole substituted N-aryl THIQ derivatives (Fig. 1).
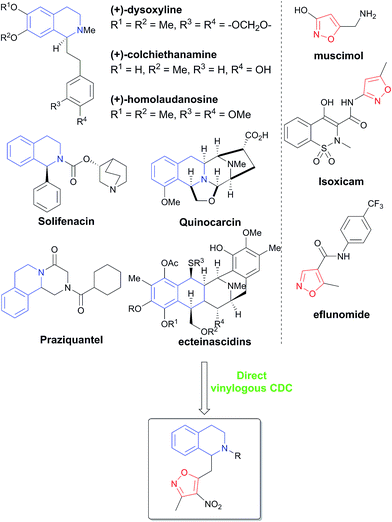 |
| Fig. 1 Selected examples of natural products and pharmaceuticals possessing tetrahydroisoquinoline and isoxazole structural framework. | |
To begin our investigation, several copper salts were initially screened to evaluate their abilities to promote the direct vinylogous CDC reaction of N-phenyl THIQ 1a with 3,5-dimethyl-4-nitroisoxazole 2 at 50 °C in THF under air conditions (Table 1, entries 1–6). Among all the copper salts tested, CuBr was proved to be the best catalyst to furnish the desired vinylogous product 3a in 65% yield. Use of organic solvent other than THF gave diminished yields (entries 7–12). To our delight, a slight improvement of yield was observed when water was used as the solvent. By elevating the reaction temperature to 80 °C, the reaction rate was increased to generate 71% of the desired product after 30 h. Using oxygen as the oxidant resulted in a similar outcome (24 h, 72% yield). Finally, air was selected as the optimal oxidant due to its natural abundance.
Table 1 Optimization of reaction conditionsa
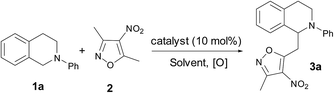
|
Entry |
Catalyst |
Solvent |
Oxidant |
Time (h) |
Yieldb (%) |
Unless otherwise noted, the reaction was performed with 0.4 mmol of 1a, 0.2 mmol of 2 and 10 mol% of catalyst in 0.4 mL solvent at 50 °C. Isolated yield. These reactions were performed at 80 °C. 5 mol% of catalyst was used. |
1 |
CuCl |
THF |
Air |
30 |
60 |
2 |
CuBr |
THF |
Air |
30 |
65 |
3 |
CuI |
THF |
Air |
30 |
14 |
4 |
CuCN |
THF |
Air |
30 |
27 |
5 |
Cu(OTf)2 |
THF |
Air |
30 |
39 |
6 |
Cu(OAc)2 |
THF |
Air |
30 |
14 |
7 |
CuBr |
DCE |
Air |
30 |
56 |
8 |
CuBr |
Toluene |
Air |
48 |
42 |
9 |
CuBr |
CH3CN |
Air |
24 |
54 |
10 |
CuBr |
EtOH |
Air |
36 |
43 |
11 |
CuBr |
DMF |
Air |
36 |
36 |
12 |
CuBr |
DMSO |
Air |
30 |
60 |
13 |
CuBr |
H2O |
Air |
48 |
67 |
14c |
CuBr |
H2O |
Air |
30 |
71 |
15c,d |
CuBr |
H2O |
Air |
48 |
50 |
16c |
CuBr |
H2O |
O2 |
24 |
72 |
Good substrate scope was observed for the copper catalysed direct vinylogous oxidative CDC addition of 3,5-dimethyl-4-nitroisoxazole to N-aryl THIQs. Aryl-substituted THIQs with electron-donating substituents in the para- and meta-positions of the phenyl ring performed well at 50 °C to give the desired products in satisfactory yields (Table 2, 3b–g), whist substrate with substituent in the ortho-position resulted in an obviously diminished yield (3h as an example). Moderate to good yields were also remained when the aryl ring bearing halogen and electron-withdrawing substituents (3i–n), albeit with a higher reaction temperature. The use of 3,5-diethyl-4-nitroisoxazole instead of 3,5-dimethyl-4-nitroisoxazole also gave the desired adduct with moderate yield (the ratio of the two diastereoisomers is 1
:
1).
Table 2 Direct vinylogous CDC reaction of N-aryltetrahydroisoquinoline with isoxazolea
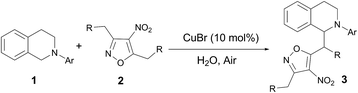
|
Unless otherwise noted, the reaction was performed with 0.4 mmol of 1, 0.2 mmol of 2 and 10 mol% of catalyst in 0.4 mL water. Yield refers to the isolated product. |
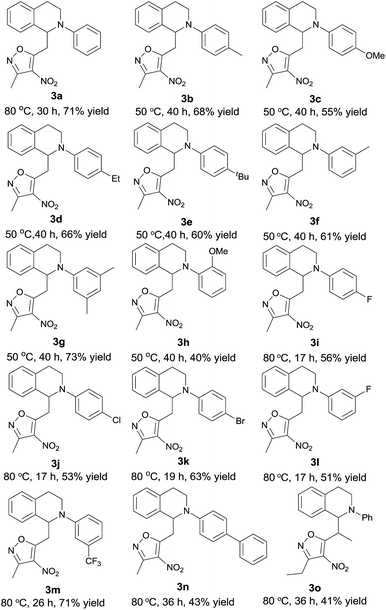 |
In addition to 4-nitroisoxazole, the direct vinylogous oxidative CDC reaction in water under air condition was also applicable to aryl methane. As shown in Scheme 1, the reaction of 2,4-dinitro-toluene13 with N-aryl THIQ 1c preceded smoothly under the similar conditions employed in Table 2 to give moderate yield of the vinylogous adduct.
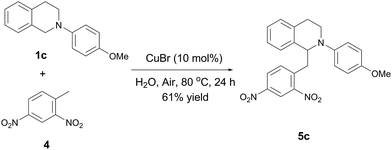 |
| Scheme 1 The vinylogous CDC reaction of 2,4-dinitro-toluene. | |
According to the mechanism for the copper catalysed CDC of THIQs clarified by Klussmann,14 a possible mechanism for the direct vinylogous CDC reaction is given in Fig. 2. The oxidation of 1 was catalysed by copper with oxygen to produce the key iminium ion intermediate I, which was trapped by nitronate anion II derived from 2, the formation of which was facilitated by copper. This vinylogous type aza-Henry addition provides the final product 3 along with H2O as the only by-product.
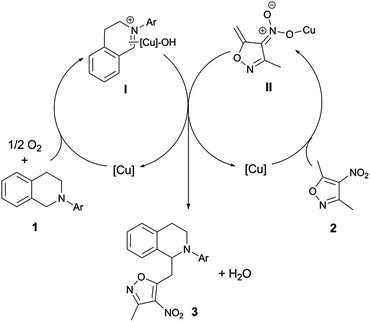 |
| Fig. 2 Possible mechanism. | |
In order to prove the synthetic potential of the current reaction, we then devoted our efforts to explore some additional transformations of the vinylogous CDC products. As shown in Scheme 2, a partial reduction of the vinylogous CDC adduct 3a was achieved using NaBH4 as the reducing agent to give isoxazoline 7 in high yield. On the other hand, the 4-nitroisoxazole core can be converted to 4-nitropyrazolyl core by treatment with hydrazine under basic condition, thus affording the pyrazol–THIQ conjugation 6 in high yield, the configuration of which was unambiguously confirmed by X-ray crystallographic analysis.15 It is noteworthy that pyrazole also represents an important scaffold in pharmaceutical and agrochemical sciences.16
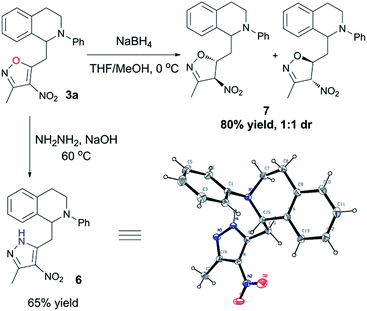 |
| Scheme 2 Transformations of the corresponding vinylogous CDC products. | |
Conclusions
In summary, we have developed a direct catalytic vinylogous cross-dehydrogenative coupling (CDC) reaction of N-aryl tetrahydroisoquinolines with vinylogous nucleophiles such as 3,5-dialkyl-4-nitroisoxazoles and 2,4-dinitro-toluene. To the best of our knowledge, this is the first example of direct vinylogous CDC reaction in water under air condition. This protocol is direct, environmentally benign and operationally simple for the synthesis of highly functionalized isoxazole substituted tetrahydroisoquinoline derivatives in moderate to good yields.
Acknowledgements
Financial support from the Jiangxi Provincial Department of Science and Technology Fund (20144BAB2130003), and National Natural Science Foundation of China (No. 51478123 and 21502021) is gratefully acknowledged.
Notes and references
- For selected reviews on CDC reactions, see:
(a) Z. Li, D. S. Bohle and C.-J. Li, Proc. Natl. Acad. Sci. U. S. A., 2006, 103, 8928 CrossRef CAS PubMed;
(b) S. I. Murahashi and D. Zhang, Chem. Soc. Rev., 2008, 37, 1490 RSC;
(c) C.-J. Li, Acc. Chem. Res., 2009, 42, 335 CrossRef CAS PubMed;
(d) W.-J. Yoo and C.-J. Li, Top. Curr. Chem., 2010, 292, 281 CrossRef PubMed;
(e) C. J. Scheuermann, Chem.–Asian J., 2010, 5, 436 CrossRef CAS PubMed;
(f) C. S. Yeung and V. M. Dong, Chem. Rev., 2011, 111, 1215 CrossRef CAS PubMed;
(g) C. Liu, H. Zhang, W. Shi and A. Lei, Chem. Rev., 2011, 111, 1780 CrossRef CAS PubMed;
(h) C.-L. Sun, B.-J. Li and Z.-J. Shi, Chem. Rev., 2011, 111, 1293 CrossRef CAS PubMed;
(i) C. Zhang, C. Tang and N. Jiao, Chem. Soc. Rev., 2012, 41, 3464 RSC;
(j) S. A. Girard, T. Knauber and C.-J. Li, Angew. Chem., Int. Ed., 2014, 53, 74 CrossRef CAS PubMed;
(k) C. Liu, J. Yuan, M. Gao, S. Tang, W. Li, R. Shi and A. Lei, Chem. Rev., 2015, 115, 12138 CrossRef CAS PubMed.
- For selected examples:
(a) S.-I. Murahashi, N. Komiya, H. Terai and T. Nakae, J. Am. Chem. Soc., 2003, 125, 15312 CrossRef CAS PubMed;
(b) O. Baslé and C.-J. Li, Chem. Commun., 2009, 4124 RSC;
(c) O. Baslé, N. Borduas, P. Dubois, J. M. Chapuzet, T. H. Chan, J. Lessard and C.-J. Li, Chem.–Eur. J., 2010, 16, 8162 CrossRef PubMed;
(d) N. Matsuyama, M. Kitahara, K. Hirano, T. Satoh and M. Miura, Org. Lett., 2010, 12, 2358 CrossRef CAS PubMed;
(e) Á. Pintér, A. Sud, D. Sureshkumar and M. Klussmann, Angew. Chem., Int. Ed., 2010, 49, 5004 CrossRef PubMed;
(f) B. Zhang, S.-K. Xiang, L.-H. Zhang, Y. Cui and N. Jiao, Org. Lett., 2011, 13, 5212 CrossRef CAS PubMed;
(g) Y. Pan, S. Wang, C. W. Kee, E. Dubuisson, Y. Yang, K. P. Loh and C.-H. Tan, Green Chem., 2011, 13, 3341 RSC;
(h) Y. Pan, C. W. Kee, L. Chen and C.-H. Tan, Green Chem., 2011, 13, 2682 RSC;
(i) C. Zhang, X. Zong, L. Zhang and N. Jiao, Org. Lett., 2012, 14, 3280 CrossRef CAS PubMed;
(j) G. Zhang, Y. Ma, S. Wang, Y. Zhang and R. Wang, J. Am. Chem. Soc., 2012, 134, 12334 CrossRef CAS PubMed;
(k) J. Xie, H. Li, J. Zhou, Y. Cheng and C. Zhu, Angew. Chem., Int. Ed., 2012, 51, 1252 CrossRef CAS PubMed;
(l) J. Dhineshkumar, M. Lamani, K. Alagiri and K. R. Prabhu, Org. Lett., 2013, 15, 1092 CrossRef CAS PubMed;
(m) A. Modak, U. Dutta, R. Kancherla, S. Maity, M. Bhadra, S. M. Mobin and D. Maiti, Org. Lett., 2014, 16, 2602 CrossRef CAS PubMed;
(n) A. Tanoue, W.-J. Yoo and S. Kobayashi, Org. Lett., 2014, 16, 2346 CrossRef CAS PubMed;
(o) F.-F. Wang, C.-P. Luo, G. Deng and L. Yang, Green Chem., 2014, 16, 2428 RSC;
(p) X. Liu, J. Zhang, S. Ma, Y. Ma and R. Wang, Chem. Commun., 2014, 50, 15714 RSC;
(q) X. Marset, J. M. Pérez and D. J. Ramón, Green Chem., 2016, 18, 826 RSC.
-
(a) P. Anastas and N. Eghbali, Chem. Soc. Rev., 2010, 39, 301 RSC;
(b) M.-O. Simon and C.-J. Li, Chem. Soc. Rev., 2012, 41, 1415 RSC.
-
(a) H.-B. Zhang, L. Liu, Y.-J. Chen, D. Wang and C.-J. Li, Eur. J. Org. Chem., 2006, 869 CrossRef;
(b) O. Baslé and C.-J. Li, Green Chem., 2007, 9, 1047 RSC;
(c) T. Nishikata and B. H. Lipshutz, Org. Lett., 2010, 12, 1972 CrossRef CAS PubMed;
(d) K. Alagiri, G. S. R. Kumara and K. R. Prabhu, Chem. Commun., 2011, 47, 11787 RSC;
(e) Q.-Y. Meng, Q. Liu, J.-J. Zhong, H.-H. Zhang, Z.-J. Li, B. Chen, C.-H. Tung and L.-Z. Wu, Org. Lett., 2012, 14, 5992 CrossRef CAS PubMed;
(f) C.-J. Wu, J.-J. Zhong, Q.-Y. Meng, T. Lei, X.-W. Gao, C.-H. Tung and L.-Z. Wu, Org. Lett., 2015, 17, 884 CrossRef CAS PubMed.
-
(a) R. Breslow, Chem. Soc. Rev., 1972, 1, 553 RSC;
(b) R. Breslow, Acc. Chem. Res., 1980, 13, 170 CrossRef CAS;
(c) H. Jiang, Ł. Albrecht and K. A. Jørgensen, Chem. Sci., 2013, 4, 2287 RSC;
(d) S. W. Smith and G. C. Fu, J. Am. Chem. Soc., 2009, 131, 14231 CrossRef CAS PubMed;
(e) S. L. Zultanski and G. C. Fu, J. Am. Chem. Soc., 2011, 133, 15362 CrossRef CAS PubMed;
(f) X.-H. Hu, J. Zhang, X.-F. Yang, Y.-H. Xu and T.-P. Loh, J. Am. Chem. Soc., 2015, 137, 3169 CrossRef CAS PubMed;
(g) L. Fu, D. M. Guptill and H. M. L. Davies, J. Am. Chem. Soc., 2016, 138, 5761 CrossRef CAS PubMed;
(h) J. A. Gurak Jr, K. S. Yang, Z. Liu and K. M. L. Engle, J. Am. Chem. Soc., 2016, 138, 5805 CrossRef PubMed;
(i) X. Chen, X. Liu and J. T. Mohr, J. Am. Chem. Soc., 2016, 138, 6364 CrossRef CAS PubMed.
- R. C. Fuson, Chem. Rev., 1935, 16, 1 CrossRef CAS.
- For selected reviews, see:
(a) G. Casiraghi, F. Zanardi, G. Appendino and G. Rassu, Chem. Rev., 2000, 100, 1929 CrossRef CAS PubMed;
(b) G. Casiraghi, L. Battistini, C. Curti, G. Rassu and F. Zanardi, Chem. Rev., 2011, 111, 3076 CrossRef CAS PubMed;
(c) I. D. Jurberg, I. Chatterjee, R. Tannert and P. Melchiorre, Chem. Commun., 2013, 49, 4869 RSC;
(d) C. Schneider and F. Abels, Org. Biomol. Chem., 2014, 12, 3531 RSC.
- H. Richter, R. Rohlmann and O. Carcía Mancheño, Chem.–Eur. J., 2011, 17, 11622 CrossRef CAS PubMed.
-
(a) M. O. Ratnikov, X. Xu and M. P. Doyle, J. Am. Chem. Soc., 2013, 135, 9475 CrossRef CAS PubMed;
(b) A. J. Catino, J. M. Nichols, B. J. Nettles and M. P. Doyle, J. Am. Chem. Soc., 2006, 128, 5648 CrossRef CAS PubMed.
-
(a) D. Jack and R. Williams, Chem. Rev., 2002, 102, 1669 CrossRef;
(b) A. Zhang, J. L. Neumeyer and R. J. Baldessarini, Chem. Rev., 2007, 107, 274 CrossRef CAS PubMed;
(c) M. Gao, D. Kong, A. Clearfield and Q.-H. Zheng, Bioorg. Med. Chem. Lett., 2006, 16, 2229 CrossRef CAS PubMed;
(d) P. Cheng, N. Huang, Z.-Y. Jiang, Q. Zhang, Y.-T. Zheng, J.-J. Chen, X.-M. Zhang and Y.-B. Ma, Bioorg. Med. Chem. Lett., 2008, 18, 2475 CrossRef CAS PubMed;
(e) T. P. Lebold, J. L. Wood, J. Deitch, M. W. Lodewyk, D. J. Tantillo and R. Sarpong, Nat. Chem., 2013, 5, 126 CrossRef CAS PubMed.
-
(a) E. V. K. F. A. Lakhvich and A. A. Akhrem, Chem. Heterocycl. Compd., 1989, 25, 359 CrossRef;
(b) J. B. Sperry and D. L. Wright, Curr. Opin. Drug Discovery Dev., 2005, 8, 723 CAS.
-
(a) Y. Zhang, Y.-L. Shao, H.-S. Xu and W. Wang, J. Org. Chem., 2011, 76, 1472 CrossRef CAS PubMed;
(b) J.-L. Zhu, Y. Zhang, C. Liu, A.-M. Zheng and W. Wang, J. Org. Chem., 2012, 77, 9813 CrossRef CAS PubMed;
(c) N. Zhu, B.-C. Ma, Y. Zhang and W. Wang, Adv. Synth. Catal., 2010, 352, 1291 CrossRef CAS;
(d) Y. Zhang, Z.-J. Li, H.-S. Xu, Y. Zhang and W. Wang, RSC Adv, 2011, 1, 389 RSC;
(e) Y. Zhang, B.-W. Wei, H. Lin, W.-R. Cui, X.-Q. Zeng and X.-L. Fan, Adv. Synth. Catal., 2015, 357, 1299 CrossRef CAS;
(f) Y. Zhang, B.-W. Wei, H. Lin, L. Zhang, J.-X. Liu, H.-Q. Luo and X.-L. Fan, Green Chem., 2015, 17, 3266 RSC;
(g) Y. Zhang, B.-W. Wei, L.-N. Zou, M.-L. Kang, H.-Q. Luo and X.-L. Fan, Tetrahedron, 2016, 72, 2742 CrossRef.
-
(a) T. Li, J. Zhu, D. Wu, X. Li, S. Wang, H. Li, J. Li and W. Wang, Chem.–Eur. J., 2013, 19, 9147 CrossRef CAS PubMed;
(b) L. D. Amico, X. Companyó, T. Naicker, T. M. Bräuer and K. A. Jørgensen, Eur. J. Org. Chem., 2013, 5262 CrossRef.
- E. Boess, C. Schmitz and M. Klussmann, J. Am. Chem. Soc., 2012, 134, 5317 CrossRef CAS PubMed.
- CCDC 1504789(6), see ESI† for details.
-
(a) N. K. Terrett, A. S. Bell, D. Brown and P. Ellis, Bioorg. Med. Chem. Lett., 1996, 6, 1819 CrossRef;
(b) W. R. Tracey, M. C. Allen, D. E. Frazier, A. A. Fossa, C. G. Johnson, R. B. Marala, D. R. Knight and A. Guzman-Perez, Cardiovasc. Drug Rev., 2003, 21, 17 CrossRef CAS PubMed;
(c) D. Havrylyuk, B. Zimenkovsky, O. Vasylenko, A. Gzella and R. Lesyk, J. Med. Chem., 2012, 55, 8630 CrossRef CAS PubMed.
Footnote |
† Electronic supplementary information (ESI) available. CCDC 1504789. For ESI and crystallographic data in CIF or other electronic format see DOI: 10.1039/c6ra26126d |
|
This journal is © The Royal Society of Chemistry 2017 |
Click here to see how this site uses Cookies. View our privacy policy here.