DOI:
10.1039/C6RA26521A
(Communication)
RSC Adv., 2017,
7, 1062-1066
N-2-Selective gold-catalyzed alkylation of 1-sulfonyl-1,2,3-trizoles†
Received
9th November 2016
, Accepted 23rd November 2016
First published on 4th January 2017
Abstract
An efficient new method was developed to synthesise N-2-alkyl-1,2,3-trizoles via gold catalyzed alkylation of 1-sulfonyl-1,2,3-trizoles with vinyl ethers. Only N-2-isomers were obtained in these reactions. The sulfonyl group in the 1-sulfonyl-1,2,3-trizoles acted as the leaving group, which was trapped by H2O in this reaction.
1,2,3-Triazoles have found widespread applications in biological science,1 material science2 and medicinal chemistry.3 More recently, they also have been utilized as ligands in transition-metal coordination,4 and this catalytic system provided an efficient strategy for many challenging transformations.5 Because of the importance of this structural motif, many practical synthetic methods have been developed. Both thermal and Cu(I)/Ru(II)-catalyzed condensations of alkynes and azides provide an excellent approach to N-1/N-3-substituted triazoles,6 whereas the regioselective synthesis of N-2-substituted 1,2,3-triazoles remains a challenging issue. Considerable recent efforts have been made toward the preparation of N-2-aryl7 and N-2-allyl-1,2,3-triazoles8 with high N-2-selectivity. Despite these achievements, however, a general method for the preparation of N-2-alkyl-1,2,3-triazols is lacking.
The current main approach to N-2-alkyl-1,2,3-triazoles by the conversion of alkyl halides with bulky C-4- and C-5-disubstituted NH-1,2,3-triazoles limits its broader utility by the substrate's steric requirements (Scheme 1a).9 Recently, Chen's group reported a highly regioselective N-2 alkylation of NH-1,2,3-triazoles through NIS-mediated iodofunctionalization with olefins (Scheme 1b, eqn (1)).10a Our interest in developing a new strategy for the synthesis of N-2-alkyl-1,2,3-triazoles was initiated by the recent success of TsOH mediated addition of 1-sulfonyl-1,2,3-trizole to olefins (Scheme 1b, eqn (2)).10b This new strategy incorporated a labile N-1-substitutents and the mechanism was based on a carbocation intermediate. Based on these results, we want to expand this reaction to metal catalyzed transformation.
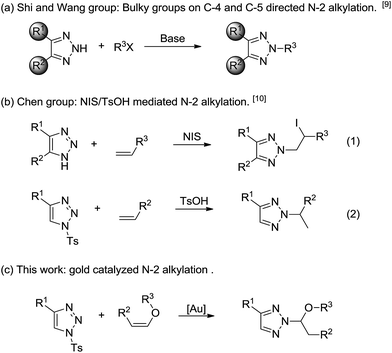 |
| Scheme 1 Strategy for selective N-2 alkylation. | |
The activation of unsaturated C–C bonds by gold complexes has led to a range of attractive and useful strategies for a variety of organic transformations due to their low toxicity and increased stability to moisture and air,11 whereas employing 1-sulfonyl-1,2,3-triazoles as the nucleophiles in gold catalyzed olefins conversion has never been explored before. In the previous studies, N-2-alkyl-substituted triazole derivatives possess a broad spectrum of antiherpetic, antiarrhythmic and antiviral activities.12 Therefore, efficient synthetic methods for the synthesis of N-2-alkyl triazoles are highly desirable. In this paper, we will report the first example of gold-catalyzed N-2 alkylation of 1-sulfonyl-1,2,3-trizoles with electronic-rich vinyl ethers (Scheme 1c).
The initial experiments were performed with 4-phenyl-1-sulfonyl-1,2,3-trizole 1a and vinyl ether 2a in the presence of IPrAuCl (5 mol%) and AgOTf (5 mol%) in 1,2-dichloroethane (DCE) at 80 °C. To our delight, the desired N-2-alkyl-1,2,3-trizole 3a was obtained in 53% yield and no N-1-coupling adduct was detected (Table 1, entry 1). In order to optimize the reaction condition, silver salts screening was first performed, in which, IPrAuCl/AgNTf2 was found to be the best silver combination (Table 1, entry 2). The catalyst's ligands were then evaluated. With Ph3PAuCl only half of the yield was obtained while JohnphosAuCl was not favored for this transformation, affording 3a in only 14% yield after 6 h (Table 1, entries 4, 5). According to the previous report of Chen's group,10b the trace amount of water is auxiliary to capture the leaving Ts group. Therefore, 2 equiv. of water was added to the reaction and 3a's yield was improved to 98% (Table 1, entry 6). Further solvent optimization identified DCE to be the best reaction medium (Table 1, entry 6). Variation of the number of equivalents of 2a from 5.0 to 3.0 lowed the conversion of 3a to 51% (Table 1, entry 11), which indicates that the excess of vinyl ether probably is necessary due to the high tendency of vinyl ethers to undergo cationic polymerization initiated by gold(I).13 Reducing the catalyst loading to 2 mol% led to a reduced reaction yield to 47% after 8 hours (Table 1, entry 12). Addition of 4 Å molecular sieves to remove the residual moisture inhibited this reaction which indicated that H2O was necessary for this N-2 alkylation reaction (Table 1, entry 13). The control experiments employing IPrAuCl and AgNTf2 separately gave no desired products (Table 1, entries 14, 15).
Table 1 Screening of the optimal conditionsa
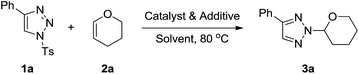
|
Entry |
Catalyst (mol%) |
Solv./additive (equiv.) |
Time (h) |
Yieldb (%) |
Unless noted, all reactions were carried out at 0.5 mmol scale in 3 mL of solvent with the addition of 5 mol% catalyst at 80 °C (1a/2a) = 1/5. Isolated yields. 3 equiv. of compound 2a were added. 100 mg 4 Å MS was added. |
1 |
IPrAuCl/AgOTf (5) |
DCE |
6.5 |
53 |
2 |
IPrAuCl/AgNTf2 (5) |
DCE |
6.5 |
61 |
3 |
IPrAuCl/AgSbF6 (5) |
DCE |
6.5 |
39 |
4 |
Ph3PAuCl/AgNTf2 (5) |
DCE |
6 |
32 |
5 |
JohnphosAuCl/AgNTf2 (5) |
DCE |
6 |
14 |
6 |
IPrAuCl/AgNTf2 (5) |
DCE/H2O (2) |
6 |
98 |
7 |
IPrAuCl/AgNTf2 (5) |
THF/H2O (2) |
6.5 |
17 |
8 |
IPrAuCl/AgNTf2 (5) |
CHCl3/H2O (2) |
6.5 |
45 |
9 |
IPrAuCl/AgNTf2 (5) |
Toluene/H2O (2) |
24 |
NR |
10 |
IPrAuCl/AgNTf2 (5) |
DCM/H2O (2) |
10 |
56 |
11c |
IPrAuCl/AgNTf2 (5) |
DCE/H2O (2) |
6 |
51 |
12 |
IPrAuCl/AgNTf2 (2) |
DCE/H2O (2) |
8 |
47 |
13d |
IPrAuCl/AgNTf2 (5) |
DCE |
24 |
NR |
14 |
IPrAuCl (5) |
DCE/H2O (2) |
24 |
NR |
15 |
AgNTf2 (5) |
DCE/H2O (2) |
24 |
NR |
With the optimized reaction conditions in hand, we examined the scope of this transformation by synthesizing a series of N-2-alkyl-1,2,3-triazoles. As shown in Table 2, various 4-aryl-substituted 1-sulfonyl-1,2,3-triazoles were explored by using vinyl ether 2a as the reactants. First, 4-phenyl-substituted 1-sulfonyl-1,2,3-triazole 1a could afford the desired N-2-alkyl-1,2,3-triazole 3a in 98% yield. 4-Alkyl and 4-methoxy phenyl substituted 1-sulfonyl-1,2,3-trizoles gave 3b–e in 74–91% yield (Table 2, entries 2–5). 4-Halogen phenyl substituted 1-sulfonyl-1,2,3-trizoles were also well tolerated, although 4-bromo phenyl substituted 1-sulfonyl-1,2,3-triazole 1g gave the corresponding product 3g in 57% yield (Table 2, entries 6–8). 2-Fluoro and 3-fluoro phenyl substituted 1-sulfonyl-1,2,3-triazoles were also tested, giving 3i and 3j in 73% and 87% yield, respectively (Table 2, entries 9 and 10). The reaction of 2-thienyl and 3-thienyl substituted 1-sulfonyl-1,2,3-triazoles 1k and 1l went smoothly, affording 3k and 3l in moderate yields (Table 2, entries 11 and 12). However, no conversion was observed for 4-alkyl-substituted 1-sulfonyl-1,2,3-trizoles, probably owing to the alkyl substituent can't stabilize the intermediate II in Scheme 3 (Table 2, entries 13 and 14). Then, N-2-alkyl reactions of 4-phenyl-substituted 1-sulfonyl-1,2,3-trizole 1a with various vinyl ether were explored. As shown in Table 3, cyclic vinyl ethers worked very well. 2-Methoxy-3,4-dihydro-2H-pyran 2b gave 4b in 81% yield, while 2,3-dihydrofuran 2c afforded 4c in 94% yield. Next, we investigated the linear vinyl ether's reactions. We found that mono-substituted and 1,2-disubstituted linear vinyl ether could be employed in this reaction and gave the desired products in moderate to good yields. Moreover, this reaction was also efficient with alpha-angelica lactone, giving 4k in 83% yield. The structure of 4k was determined according to the literature of Chen.10b However, 1,1-disubstituted vinyl ether 2l, styrene 2m, 4-tert-butyl substituted styrene 2n did not work in this transformation may be due to the larger steric effects and lower complexation with gold(I).
Table 2 Substrate scope of 1-sulfonyl-1,2,3-trizoles (1)a
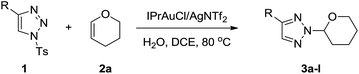
|
Entry |
Substrate 1 |
R |
Product 3 |
Yieldb (%) |
Reaction conditions: 1 (0.5 mmol), 2a (2.5 mmol), H2O (1 mmol), IPrAuCl/AgNTf2 (5 mol%), DCE (3 mL), 80 °C. Yield of isolated product. |
1 |
1a |
Phenyl |
3a |
98 |
2 |
1b |
4-MeC6H4 |
3b |
74 |
3 |
1c |
4-PrC6H4 |
3c |
84 |
4 |
1d |
4-tBuC6H4 |
3d |
86 |
5 |
1e |
4-MeOC6H4 |
3e |
91 |
6 |
1f |
4-ClC6H4 |
3f |
77 |
7 |
1g |
4-BrC6H4 |
3g |
57 |
8 |
1h |
4-FC6H4 |
3h |
99 |
9 |
1i |
2-FC6H4 |
3i |
73 |
10 |
1j |
3-FC6H4 |
3j |
87 |
11 |
1k |
2-Thienyl |
3k |
63 |
12 |
1l |
3-Thienyl |
3l |
70 |
13 |
1m |
nBu |
3m |
0 |
14 |
1n |
Cyclopentyl |
3n |
0 |
Table 3 Substrate scope of vinyl ether (2)a
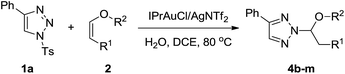
|
Reaction conditions: 1a (0.5 mmol), 2 (2.5 mmol), H2O (1 mmol), IPrAuCl/AgNTf2 (5 mol%), DCE (3 mL), 80 °C. |
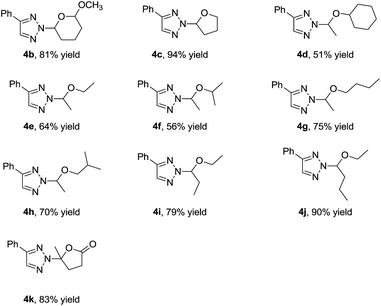 |
To gain more insight into the mechanism of this reaction, deuterium-labeling experiments were conducted. When H2O was replaced by 2.0 equiv. of D2O in the model reaction, the N-2-alkyl-1,2,3-trizole product 4e-D was isolated in 58% yield. The incorporation of deuterium at the α-position of 4e-D in a 28% ratio suggested that H2O was necessary for this N-2 alkylation reaction (Scheme 2).14 The incorporation of deuterium at the α-position of 4e-D was lowed in 5% yield may be due to the trace amount of water in the reaction system.
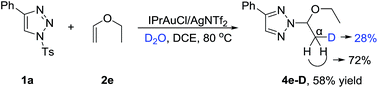 |
| Scheme 2 Deuterium-labeling experiments. | |
On the basis of previous work10b and our deuterium-labeling experiments, a plausible15 catalytic cycle is proposed in Scheme 3. Complexation of the cationic gold catalyst with vinyl ether 2a generated intermediate I, which is then attacked by the internal nitrogen of the 1-sulfonyl-1,2,3-triazole substrate 1a to give the intermediate II. Then the activated sulfur–N bond is hydrolyzed to form the alkyl gold intermediate III, which subsequently undergoes protodeauration16 to give the final N-2-alkyl-1,2,3-trizole 3a and regenerated the cationic gold catalyst.
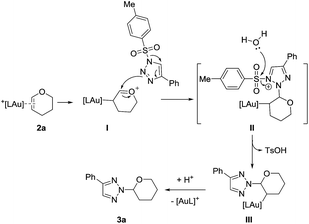 |
| Scheme 3 Proposed reaction mechanism. | |
In summary, a highly efficient gold-catalyzed N-2-selective alkylation was developed, giving the desired N-2-alkyl-1,2,3-trizoles in good yields. The sulfonyl group in the 1-sulfonyl-1,2,3-trizoles acted as the leaving group, which was trapped by H2O in this reaction. Notably, only N-2-isomers were obtained in these reactions. With the continuously growing interest in N-2-substituted 1,2,3-trizoles, we are currently studying the N-2-selective arylation, alkenylation, and allylation using this strategy and the results will be reported in due course.
Acknowledgements
This work was financially supported by the Project of Education Department of Sichuan Province (No. 16ZB0027) and the Functional Polymer Innovation Team Project, Southwest University for Nationalities (No. 14CXTD04).
Notes and references
-
(a) M. E. Hahn and T. W. Muir, Trends Biochem. Sci., 2005, 30, 26–34 CrossRef CAS PubMed;
(b) W. P. Heal, S. R. Wickramasinghe, R. J. Leatherbarrow and E. W. Tate, Org. Biomol. Chem., 2008, 6, 2308–2315 RSC;
(c) A. M. Phil, P. Schmieder, R. Kuhne and J. Rademann, Angew. Chem., Int. Ed., 2009, 48, 5042–5045 CrossRef PubMed;
(d) G. Schneider, Nat. Rev. Drug Discovery, 2010, 9, 273–276 CrossRef CAS PubMed.
-
(a) H. M. Li, F. O. Cheng, A. M. Duft and A. Adronov, J. Am. Chem. Soc., 2005, 127, 14518–14524 CrossRef CAS PubMed;
(b) D. I. Rozkiewicz, D. Janczewski, W. Verboom, B. J. Ravoo and D. N. Reinhoudt, Angew. Chem., Int. Ed., 2006, 45, 5292–5296 CrossRef CAS PubMed;
(c) M. Wyszogrodzka and R. Haag, Chem.–Eur. J., 2008, 14, 9202–9214 CrossRef CAS PubMed;
(d) T. Gadzikwa, O. K. Farha, C. D. Malliakas, M. G. Kanatzidis, J. T. Hupp and S. T. Nguyen, J. Am. Chem. Soc., 2009, 131, 13613–13615 CrossRef CAS PubMed;
(e) P. L. Golas and K. Matyjaszewski, Chem. Soc. Rev., 2010, 39, 1338–1354 RSC.
-
(a) Y. M. Chabre and R. Roy, Curr. Top. Med. Chem., 2008, 8, 1237–1285 CrossRef CAS PubMed;
(b) M. Colombo and I. Peretto, Drug Discovery Today, 2008, 13, 677–684 CrossRef CAS PubMed;
(c) R. Hanselmann, G. E. Job, G. Johnson, R. L. Lou, J. G. Martynow and M. M. Reeve, Org. Process Res. Dev., 2010, 14, 152–158 CrossRef CAS;
(d) R. Moumne, V. Larue, B. Seijo, T. Lecourt, L. Micouin and C. Tisne, Org. Biomol. Chem., 2010, 8, 1154–1159 RSC.
-
(a) A. L. Rheingold, L. M. Liable-Sands and S. Trofimenko, Angew. Chem., Int. Ed., 2000, 39, 3321–3324 CrossRef CAS;
(b) S. Trofimenko, A. L. Rheingold and C. D. Incarvito, Angew. Chem., Int. Ed., 2003, 42, 3506–3509 CrossRef CAS PubMed;
(c) T. R. Chan, R. Hilgraf, K. B. Sharpless and V. V. Fokin, Org. Lett., 2004, 6, 2853–2855 CrossRef CAS PubMed;
(d) D. Liu, W. Z. Gao, Q. Dai and X. M. Zhang, Org. Lett., 2005, 7, 4907–4910 CrossRef CAS PubMed;
(e) H. F. Duan, S. Sengupta, J. L. Petersen, N. G. Akhmedov and X. D. Shi, J. Am. Chem. Soc., 2009, 131, 12100–12102 CrossRef CAS PubMed;
(f) H. F. Duan, S. Sengupta, J. L. Petersen and X. D. Shi, Organometallics, 2009, 28, 2352–2355 CrossRef CAS;
(g) J. E. Hein, J. C. Tripp, L. B. Krasnova, K. B. Sharpless and V. V. Fokin, Angew. Chem., Int. Ed., 2009, 48, 8018–8021 CrossRef CAS PubMed;
(h) W. M. Yan, X. H. Ye, N. G. Akhmedov, J. L. Petersen and X. D. Shi, Org. Lett., 2012, 14, 2358–2361 CrossRef CAS PubMed;
(i) Y. C. Yang, A. Qin, K. Y. Zhao, D. W. Wang and X. D. Shi, Adv. Synth. Catal., 2016, 358, 1433–1439 CrossRef CAS.
- Selected examples on the applications of TA–Au, see:
(a) D. W. Wang, L. N. S. Gautam, C. Bollinger, A. Harris, M. Y. Li and X. D. Shi, Org. Lett., 2011, 13, 2618–2621 CrossRef CAS PubMed;
(b) D. W. Wang, Y. W. Zhang, R. Cai and X. D. Shi, Beilstein J. Org. Chem., 2011, 7, 1014–1020 CrossRef CAS PubMed;
(c) D. W. Wang, Y. W. Zhang, A. Harris, L. N. S. Gautam, Y. F. Chen and X. D. Shi, Adv. Synth. Catal., 2011, 353, 2584–2588 CrossRef CAS;
(d) Q. Y. Wang, S. Aparaj, N. G. Akhmedov, J. L. Petersen and X. D. Shi, Org. Lett., 2012, 14, 1334–1337 CrossRef CAS PubMed;
(e) R. Cai, W. M. Yan, M. G. Bologna, K. de Silva, Z. Ma, H. O. Finklea, J. L. Petersen, M. Y. Li and X. D. Shi, Org. Chem. Front., 2015, 2, 141–144 RSC;
(f) S. E. Motika, Q. Y. Wang, X. H. Ye and X. D. Shi, Org. Lett., 2015, 17, 290–293 CrossRef CAS PubMed;
(g) Y. C. Yang, Y. A. Shen, X. L. Wang, Y. Zhang, D. W. Wang and X. D. Shi, Tetrahedron Lett., 2016, 57, 2280–2282 CrossRef CAS.
-
(a) R. Huisgen, 1,3-Dipolar Cycloaddition Chemistry, ed. A. Padwa, Wiley, New York, 1984 Search PubMed;
(b) H. C. Kolb, M. G. Finn and K. B. Sharpless, Angew. Chem., Int. Ed., 2001, 40, 2004–2021 CrossRef CAS;
(c) V. V. Rostovtsev, L. G. Green, V. V. Fokin and K. B. Sharpless, Angew. Chem., Int. Ed., 2002, 41, 2596–2599 CrossRef CAS;
(d) P. Wu and V. V. Fokin, Aldrichimica Acta, 2007, 40, 7–17 CAS;
(e) B. C. Boren, S. Narayan, L. K. Rasmussen, L. Zhang, H. T. Zhao, Z. Y. Lin, G. C. Jia and V. V. Fokin, J. Am. Chem. Soc., 2008, 130, 8923–8930 CrossRef CAS PubMed;
(f) C. W. Tornoe, C. Christensen and M. Meldal, J. Org. Chem., 2002, 67, 3057–3064 CrossRef CAS PubMed;
(g) M. M. Majireck and S. M. Weinreb, J. Org. Chem., 2006, 71, 8680–8683 CrossRef CAS PubMed;
(h) L. Zhang, X. G. Chen, P. Xue, H. H. Y. Sun, I. D. Williams, K. B. Sharpless, V. V. Fokin and G. C. Jia, J. Am. Chem. Soc., 2005, 127, 15998–15999 CrossRef CAS PubMed.
- For examples of N2-arylation:
(a) K. S. Balachandran, I. Hiryakkanavar and M. V. George, Tetrahedron, 1975, 31, 1171–1177 CrossRef CAS;
(b) M. Taillefer, N. Xia and A. Ouali, Angew. Chem., Int. Ed., 2007, 46, 934–936 CrossRef CAS PubMed;
(c) Y. X. Liu, W. M. Yan, Y. F. Chen, J. L. Petersen and X. D. Shi, Org. Lett., 2008, 10, 5389–5392 CrossRef CAS PubMed;
(d) X. J. Wang, L. Zhang, H. Lee, N. Haddad, D. Krishnamurthy and C. H. Senanayake, Org. Lett., 2009, 11, 5026–5028 CrossRef CAS PubMed;
(e) S. Ueda, M. J. Su and S. L. Buchwald, Angew. Chem., Int. Ed., 2011, 50, 8944–8947 CrossRef CAS PubMed;
(f) J. Wen, L. L. Zhu, Q. W. Bi, Z. Q. Shen, X. X. Li, X. Li, Z. Wang and Z. L. Chen, Chem.–Eur. J., 2014, 20, 974–978 CrossRef CAS PubMed;
(g) A. B. Lopes, P. Wagner, R. de Souza, N. L. Germain, J. Uziel, J. J. Bourguignon, M. Schmitt and L. S. M. Miranda, J. Org. Chem., 2016, 81, 4540–4549 CrossRef CAS PubMed.
- For the synthesis of N2-allyl 1,2,3-triazole:
(a) S. Kamijo, T. N. Jin, Z. B. Huo and Y. Yamamoto, J. Am. Chem. Soc., 2003, 125, 7786–7787 CrossRef CAS PubMed;
(b) S. Kamijo, T. Jin, Z. B. Huo and Y. Yamamoto, J. Org. Chem., 2004, 69, 2386–2393 CrossRef CAS PubMed;
(c) W. M. Yan, Q. Y. Wang, Y. F. Chen, J. L. Petersen and X. D. Shi, Org. Lett., 2010, 12, 3308–3311 CrossRef CAS PubMed;
(d) K. Xu, N. Thieme and B. Breit, Angew. Chem., Int. Ed., 2014, 53, 7268–7271 CrossRef CAS PubMed.
-
(a) Y. F. Chen, Y. X. Liu, J. L. Petersen and X. D. Shi, Chem. Commun., 2008, 3254–3256 RSC;
(b) J. Kalisiak, K. B. Sharpless and V. V. Fokin, Org. Lett., 2008, 10, 3171–3174 CrossRef CAS PubMed;
(c) X. J. Wang, K. Sidhu, L. Zhang, S. Campbell, N. Haddad, D. C. Reeves, D. Krishnamurthy and C. H. Senanayake, Org. Lett., 2009, 11, 5490–5493 CrossRef CAS PubMed;
(d) X. J. Wang, L. Zhang, D. Krishnamurthy, C. H. Senanayake and P. Wipf, Org. Lett., 2010, 12, 4632–4635 CrossRef CAS PubMed.
-
(a) L. L. Zhu, X. Q. Xu, J. W. Shi, B. L. Chen and Z. L. Chen, J. Org. Chem., 2016, 81, 3568–3575 CrossRef CAS PubMed;
(b) J. W. Shi, L. L. Zhu, J. Wen and Z. L. Chen, Chin. J. Catal., 2016, 37, 1222–1226 CrossRef CAS.
- For selected reviews on the activition of unsaturated C–C bonds by gold complexes:
(a) A. S. K. Hashmi, Chem. Rev., 2007, 107, 3180–3211 CrossRef CAS PubMed;
(b) A. Corma, A. Leyva-Perez and M. J. Sabater, Chem. Rev., 2011, 111, 1657–1712 CrossRef CAS PubMed;
(c) H. Huang, Y. Zhou and H. Liu, Beilstein J. Org. Chem., 2011, 7, 897–936 CrossRef CAS PubMed;
(d) F. Lopez and J. L. Mascarenas, Beilstein J. Org. Chem., 2011, 7, 1075–1094 CrossRef CAS PubMed;
(e) H. Ohno, Isr. J. Chem., 2013, 53, 869–882 CrossRef CAS;
(f) G. Abbiati, E. Rossi, G. Abbiati and E. Rossi, Beilstein J. Org. Chem., 2014, 10, 481–513 CrossRef PubMed;
(g) D. Qian and J. Zhang, Chem. Soc. Rev., 2015, 44, 677–698 RSC;
(h) D. Pflasterer and A. S. K. Hashmi, Chem. Soc. Rev., 2016, 45, 1331–1367 RSC;
(i) A. M. Asiri and A. S. K. Hashmi, Chem. Soc. Rev., 2016, 45, 4471–4503 RSC.
- Examples of N-2-alkylated triazoles in biological application:
(a) O. S. Kanishchev, G. P. Gudz, Y. G. Shermolovich, N. V. Nesterova, S. D. Zagorodnya and A. V. Golovan, Nucleosides, Nucleotides Nucleic Acids, 2011, 30, 768–783 CrossRef CAS PubMed;
(b) B. E. Blass, K. Coburn, W. Lee, N. Fairweather, A. Fluxe, S. D. Wu, J. M. Janusz, M. Murawsky, G. M. Fadayel, B. Fang, M. Hare, J. Ridgeway, R. White, C. Jackson, L. Djandjighian, R. Hedges, F. C. Wireko and A. L. Ritter, Bioorg. Med. Chem. Lett., 2006, 16, 4629–4632 CrossRef CAS PubMed;
(c) M. Whiting, J. Muldoon, Y. C. Lin, S. M. Silverman, W. Lindstrom, A. J. Olson, H. C. Kolb, M. G. Finn, K. B. Sharpless, J. H. Elder and V. V. Fokin, Angew. Chem., Int. Ed., 2006, 45, 1435–1439 CrossRef CAS PubMed;
(d) C. D. Cox, M. J. Breslin, D. B. Whitman, J. D. Schreier, G. B. McGaughey, M. J. Bogusky, A. J. Roecker, S. P. Mercer, R. A. Bednar, W. Lemaire, J. G. Bruno, D. R. Reiss, C. M. Harrell, K. L. Murphy, S. L. Garson, S. M. Doran, T. Prueksaritanont, W. B. Anderson, C. Y. Tang, S. Roller, T. D. Cabalu, D. H. Cui, G. D. Hartman, S. D. Young, K. S. Koblan, C. J. Winrow, J. J. Renger and P. J. Coleman, J. Med. Chem., 2010, 53, 5320–5332 CrossRef CAS PubMed;
(e) L. Zhang, Z. B. Li, X. J. Wang, N. Yee and C. H. Senanayake, Synlett, 2012, 1052–1056, DOI:10.1055/s-0031-1290770.
-
(a) J. Urbano, A. J. Hormigo, P. de Fremont, S. P. Nolan, M. M. Diaz-Requejo and P. J. Perez, Chem. Commun., 2008, 759–761 RSC;
(b) A. S. K. Hashmi, S. Schafer, V. Goker, C. D. Eisenbach, K. Dirnberger, Z. Zhao-Karger and P. Crewdson, Aust. J. Chem., 2014, 67, 500–506 CrossRef CAS;
(c) F. Nzulu, S. Telitel, F. Stoffelbach, B. Graff, F. Morlet-Savary, J. Lalevee, L. Fensterbank, J. P. Goddard and C. Ollivier, Polym. Chem., 2015, 6, 4605–4611 RSC;
(d) F. Nzulu, A. Bontemps, J. Robert, M. Barbazanges, L. Fensterbank, J. P. Goddard, M. Malacria, C. Ollivier, M. Petit, J. Rieger and F. Stoffelbach, Macromolecules, 2014, 47, 6652–6656 CrossRef CAS.
- The extent of deuterium incorporation was determined using 1H NMR spectroscopy, see the ESI.†.
- A. S. K. Hashmi, Angew. Chem., Int. Ed., 2010, 49, 5232–5241 CrossRef CAS PubMed.
- A. S. K. Hashmi, Catal. Today, 2007, 122, 211–214 CrossRef CAS.
Footnote |
† Electronic supplementary information (ESI) available. See DOI: 10.1039/c6ra26521a |
|
This journal is © The Royal Society of Chemistry 2017 |
Click here to see how this site uses Cookies. View our privacy policy here.