DOI:
10.1039/C6RA27516H
(Paper)
RSC Adv., 2017,
7, 6511-6522
Composition, antioxidant activities and hepatoprotective effects of the water extract of Ziziphus jujuba cv. Jinsixiaozao
Received
29th November 2016
, Accepted 9th January 2017
First published on 20th January 2017
Abstract
In this study, we aimed to evaluate the composition, antioxidant activities, and hepatoprotective effects of water extracts of Ziziphus jujuba cv. Jinsixiaozao. In the water extract, the contents of total phenolics, total flavonoids and polysaccharides were 1.43 mg of gallic acid equivalent (GAE) per g of dry plant, 0.17 mg of rutin equivalent (RE) per g of dry plant, and 3.80 mg per g of dry plant, respectively. The main components of the phenolic acids were protocatechuic acid, catechol, p-hydroxybenzoic acid, vanilla acid and p-coumaric acid (17.02, 5.87, 7.46, 2.95, 1.06 μg per g of dry plant, respectively). The results show that the water extract of jujube (WEJ) had a significant antioxidant effect which was evaluated by DPPH, ABTS, ORAC and FRAP assays. In addition, they dramatically (p < 0.01) decreased the liver index, the activities of serum hepatic AST, ALT and LDH, levels of total cholesterol (TC) and triglyceride (TG) which were induced by alcohol. The administration of mice with the WEJ significantly (p < 0.01) reduced MDA and enhanced the activities of antioxidant enzymes SOD and GSH-PX compared to the model group. WEJ pre-intake decreased subsequent alcohol-induced IL-6 and TNF-α; and reduced the nuclear factor kappa (NF-κB p65) and total nitric oxide synthase (iNOS) activities in the liver (p < 0.01). Furthermore, the pathological analyses of liver proved the preventive and therapeutic effects of the WEJ in mice. Therefore, the water extract of jujube with antioxidant activities could be used to prevent and treat liver damage and inflammation induced by alcohol.
1. Introduction
Alcoholism is considered as one of the most severe addictive diseases prevalent at present.1 After long- or short-term excessive alcohol drinking, alcoholic liver disease (ALD) may lead to serious liver injury.2 As the main site of metabolic functions, liver is the main target organ of ethanol toxicity.3 Alcohol-induced liver toxicity is due to a high concentration of alcohol in the blood and the metabolic consequences of ethanol metabolism.4 The ethanol metabolites in the body including reactive oxygen species (ROS) and acetaldehyde could deplete glutathione (GSH) and cause free radical-mediated cell apoptosis in the liver.5 Therefore, alcohol-induced oxidative stress seems to play a major role in the pathogenesis of alcoholic liver disease (ALD).6 Recently, there has been a growing focus on functional food alleviating oxidative stress and liver disease induced by alcohol.
The jujube (Ziziphus jujuba Mill), a member of the Rhamanaceae, originated in China, is widely distributed in China, Korea and Japan.7,8 Ziziphus jujuba is one of the most important economic trees in China because of drought and barren soil resistance, high-yield, rich nutrition, significant benefits of economy and ecology.9,10 Jujube has a high nutritional value and heath care function. The nutrients of jujube including flavonoid, polyphenol, polysaccharide, protein, amino acid, fat, nucleotide, triterpenes, saponin, alkaloid, mineral element, vitamin and other bioactive substances,2,11–15 contribute to antioxidant,16,17 antimicrobial,18 antitumor,19 hepatoprotective,2,20 nourish blood, soothe the nerves, boost immunity and so on.21 Especially, antioxidant activity becomes a hot research area in food science and biomedical fields recently. Therefore, at home and abroad, extensive attention has been drawn by the development and utilization of jujube in recent years. Up to date, a lot of studies on jujube pharmacological actions have been investigated. However, few research of jujube on the prevention and treatment of alcohol-induced liver damage have been reported.
In this study, we investigated the components and antioxidant activity of water extract of the jujube (WEJ). Besides, protective and therapeutic effects against acute and chronic alcoholic liver injury in mice were studied.
2. Materials and methods
2.1. Materials and chemicals
Z. jujuba cv. Jinsixiaozao was obtained from Laoling, Shandong, China. HPLC grade acetonitrile were purchased from Sinopharm Chemical Reagent Co., Ltd. (Shanghai, China). Triglyceride (TG), total cholesterol (TC), alanine aminotransferase (ALT), aspartate aminotransferase (AST), lactate dehydrogenase (LDH), total superoxide dismutase (T-SOD), glutathione peroxidase (GSH-Px), malondialdehyde (MDA) and protein assay kits were obtained from Nanjing Jiancheng Bioengineering Institute (China). iNOS and NF-κB p65 were purchased from Cell Signaling Technology (Danver, MA, USA).
2.2. Preparation of the water extract
Z. jujuba cv. Jinsixiaozao were cleaned and boiled in water and crushed with a homogenizer. After removing the jujube kernels, the slurry was transferred to the extraction tank with the addition of pectinase and cellulase and hydrolyzed at 45 °C for 2 h. The insoluble materials were removed to obtain the water extract. The water extract of jujube (WEJ) was freeze-dried and stored at 4 °C until analyzed.
2.3. Determination of total bioactive components
2.3.1. Determination of polysaccharides content. Anhydrous ethanol was slowly added to the WEJ until reaching the end concentration of 80% (v/v). Finally, the sediment was filtrated to get polysaccharides. The polysaccharides were determined colorimetrically with the phenol–sulfuric acid method. Glucose calibration solutions were set up with eight different concentrations (80–360 μg mL−1). According to the standard curve, the content of the polysaccharides were calculated.
2.3.2. Determination of total flavonoid content. Flavonoid has been extracted by ultrasonic extraction method from the WEJ. The WEJ was concentrated and added anhydrous ethanol slowly to precipitate the macromolecules. The supernatant was combined and removed ethanol using a rotary evaporator. The product was dissolved in 50% methanol to determination. The total flavonoid content of the WEJ was investigated by aluminum nitrate method. The calibration curve was prepared by diluting rutin in 50% methanol (0–60 μg mL−1). Then 0.3 mL of 5% NaNO2 solution was added to 3 mL of the sample, and after 6 min 0.3 mL of 10% Al(NO2)3 solution was added. The mixture was allowed to stand for 5 min and next 4.0 mL of 10% NaOH solution was added and the total was made up to 10 mL with distilled water. The mixture was kept at room temperature for 15 minutes. Then the maximum absorbance of the mixture was measured at 510 nm using a UV-VIS spectrophotometer.
2.3.3. Determination of phenolic compounds. The total phenolic content (TPC) of the WEJ was determined according to Folin–Ciocalteu colorimetric method by using gallic acid as standard. 0.5 mL of sample solution was mixed with 0.5 mL of Folin–Ciocalteu reagent for 5 min at room temperature and then 1.5 mL Na2CO3 (20%) was combined with the mixture. The distilled water was added to 10 mL and the mixture was incubated for 10 min at 75 °C. The absorbance was read at 760 nm. The total phenolic content was expressed as milligrams of gallic acid equivalent per milliliter of sample. Each group test was performed in three times.
2.4. HPLC-UV and HPLC-MS assay of the phenolic compounds
Analysis of phenolic compounds was performed on an HPLC system (Agilent 1260, USA). All separation was using a phenomenex luna C18 (4.6 mm × 250 mm, 5 μm) column at a column temperature of 30 °C and the injection volume was 10 μL. The mobile phase was composed of A (acetonitrile) and B (0.1% formic acid in water) with gradient elution: 0 min (5% A), 0–30 min (5–15% A), 30–50 min (15–30% A), 50–55 min (30–80% A), 55–60 min (80–5% A) was used in this study. The flow-rate was maintained at 1.0 mL min−1. The peaks of phenolic acids were detected at a wavelength of 280 nm with the spectral analysis. Identification of the phenolic compounds was achieved by comparison of retention times and spectral data with those of authentic standards. The mobile phase was filtered through a 0.45 μm membrane filter and degassed under vacuum. HPLC analyses were carried out in triplicate for each sample.
Gallic acid, protocatechuic acid, catechol, p-hydroxybenzoic acid, catechin, chlorogenic acid, vanilla acid, caffeic acid, procyanidin, epicatechol, p-coumaric acid, ferulic acid, rutin, hyperoside, isoquercitrin, kaempferol-3-O-rutinoside, quercitrin, phloretin, cinnamic acid and quercetin were used as standard compounds.
HPLC-MS analysis was conducted on a Shimadzu LC-20AD XR series HPLC system (Shimadzu, Japan) coupled to an AB Sciex API 5000 Turbo-ion-spray triple quadrupole tandem mass spectrometer (Concord, Ontario, Canada) via an electrospray ionization (ESI) source in a negative mode. The column and the applied chromatographic conditions were the same as those was used in the HPLC analysis. Full scan data were acquired by scanning from the mass range of m/z 50 to 500.
2.5. Antioxidant activity in vitro
A lot of reports confirmed that antioxidant activity plays an important role in the treatment of liver injury, so we used four different methods to determine the antioxidant activity of the WEJ. Antioxidant activity of the WEJ was evaluated by using the following assays: DPPH radical scavenging activity, Ferric Reducing Antioxidant Power assay (FRAP), ABTS radical scavenging capacity assay, oxygen radical absorbance capacity assay (ORAC). Antioxidant activity was measured by quantitative detection kit for food.
2.6. Animal experiments
Balb/c mice (weighing 18–22 g) were purchased from Beijing HFK Bioscience Co., Ltd. Mice were housed in groups of six in an aerating system with 12/12 h light/dark cycles and fed with food and water. The housing conditions were maintained at temperature of 20 ± 2 °C, relative humidity at 60 ± 5%. Mice in this experiment were acclimated to the laboratory for 1 week before experiments. All the animal study procedures were carried out by following the guidelines of “Guide to the Care and Use of Laboratory Animals” (National Research Council and Shandong University) and approved by the Ethical Committee of Experimental Animal Center of Shandong University (Shandong province, China).
2.6.1. Preventive effects of WEJ on the acute alcohol-induced liver damage. After one week environmental adaptation period, mice were divided into six groups randomly: the control, the alcohol, the low concentration of WEJ (WEJ-L + A), the middle concentration of WEJ (WEJ-M + A), the high concentration of WEJ (WEJ-H + A) and the hepatic protectant, bifendate (bifendate + A). All experiments used six mice per group. WEJ groups were treated with WEJ (100, 200 or 400 mg kg−1, p.o.) once daily for 30 continuous days before being damaged by administering alcohol. The bifendate group was given at a dose of 200 mg kg−1 (p.o.) in the same manner. While control and alcohol groups were given only the distilled water (p.o.). On the 31st day, alcohol (50%, v/v, 15 mL kg−1) orally was given to induce liver injury for a single dose each day up to 7 days except the control group. All alcohol and samples were administered to mice by gavage. On the last day, the blood was taken by removing eyeball and the mice were sacrificed. Blood samples were placed at room temperature for 30 min and centrifuged at 4000 rpm for 10 min. Centrifuged serum was stored at −80 °C for later use. Subsequently, the livers were excised from the mice, weighed, and stored for further studies as needed.
2.6.2. Treatment of WEJ on the chronic alcohol-induced liver damage. After the environmental adaptation and grouping, the control group was received distilled water orally. The alcohol group, the bifendate group (A + bifendate) and WEJ groups (A + WEJ-L, A + WEJ-M, A + WEJ-H) were administered intragastrically with alcohol (50%, v/v, 8 mL kg−1) for a single dose each day up to 4 weeks. When the liver of mice was damaged, the groups were given alcohol continuously except the control group. WEJ groups received the WEJ at a dose of 100, 200 or 400 mg kg−1 body weight respectively and the bifendate group was given a dose at 200 mg kg−1, 6 h after administration of alcohol for 4 weeks each day. This experiment lasted for 8 weeks. On the last day, the serum was examined to investigate the therapeutic effects on acute hepatic damage caused by alcohol.
2.6.3. Observation of liver and calculation of liver index. Before the liver was dissected, its color and surface were observed by visual inspection. The liver index was calculated as the percent ratio of wet liver weight to wet body weight.
Liver index (%) = (liver weight/body weight) × 100% |
2.6.4. Determination of TC in serum and TG in liver tissues. The serum TC levels were determined by using microplate reader according to the instruction provided by the commercial assay kits. Prior to the determination for the hepatic TG, liver was homogenized with absolute alcohol (w/v: 1/9) in ice-cold. After centrifugation (2500g for 10 min) at 4 °C, the supernatant was transferred into a new glass tube. The hepatic TG in the supernatant were stored and measured by enzymatic colorimetric methods using commercial kits (Nanjing Jiancheng Bioengineering Institute, Nanjing, China). The serum TC and hepatic TG levels were expressed as mmol L−1 and nmol per g prot, respectively.
2.6.5. Measurement of ALT, AST and LDH activities in serum. Activities of ALT, AST and LDH were used as biochemical markers and determined by colorimetry according to provided procedures. The colorimetry was read with enzymatic-reader using commercially available diagnostic kits according to the instructions.
2.6.6. Determination of GSH-Px, T-SOD, and MDA in liver tissues. Tissue samples were homogenized with nine times volume of ice-cold physiological saline before measuring. The supernatants were collected to determine the concentrations of total superoxide dismutase (T-SOD), malondialdehyde (MDA) and glutathione peroxidase (GSH-Px) according to instructions. Protein content was determined by protein assay kit. The levels of T-SOD, MDA and GSH-Px were normalized with protein.
2.6.7. Tests for IL-6 and TNF-α level. The levels of cytokine (IL-6 and TNF-α) in the serum was determined by colorimetry according to provided procedures. The colorimetry was read with enzymatic-reader using the mouse ELISA kit (Shanghai Westang Bio-Tech Co., Ltd., Shanghai, China) according to the instructions.
2.6.8. Western blot analysis. 200 mg of liver samples were homogenized and lysed for western blotting analysis. These samples were then centrifuged at 12
000g for 15 min at 4 °C. The supernatants were collected and the protein levels were determined by BCA protein assay kit with bovin serum albumin (BSA) as a standard. Total proteins (50 μg per lane) were separated by 8–15% SDS-PAGE, and transferred on a polyvinylidene difluoride (PVDF) membrane. After blocking with a solution containing 5% skim milk for 2 h at room temperature to prevent non-specific binding of antibody, membrane was incubated with mouse anti-iNOS, anti-NF-κB monoclonal antibody (1
:
1000) at 4 °C overnight. After washing, membranes were incubated with horseradish-peroxidase (HRP)-conjugated secondary antibody (1
:
10
000) in TBST containing 5% milk at room temperature for 2 h. Then, the membrane preparations were washed three times in TBST and visualized by enhanced chemiluminescence system (Merck Millipore, Darmstadt, Germany). The films were subsequently scanned, and the signal intensity of each band was determined by Alphalmager HP system (Cell Biosciences, Inc., Santa Clara, CA, USA).
2.6.9. Histopathological evaluation. The liver tissues were immersed in 10% formaldehyde for over 24 h and routinely processed for embedding in paraffin, cutting into sections by using a microtome, staining with hematoxylin–eosin for routine histopathological examination. Each section was photographed under a light microscope to show the degree of liver damage. All images of each sample were taken at a magnification of ×200.
2.7. Statistical analysis
Each value was expressed as mean ± SD (standard deviation) (n = 6). The statistical significance was analyzed by student's test using SPSS for mean differences among the samples. Differences between groups at p < 0.05 were considered statistically significant.
3. Results
3.1. Bioactive compositions of the extract
It is not logical to evaluate the biological activities of the extract without considering their chemistry. Biological activities of the plant species are strictly depending on the bioactive compounds occur naturally in the plant body. In order to make a healthy assessment on the biological activity potential of any plant species, it is necessary to reveal the chemical composition as well. As presented in the experimental section of this paper, the water extract of jujube was evaluated for their total phenolics, favonoids and polysaccharides (Table 1).
Table 1 Total bioactive components of WEJ (mean ± SD)
Assays |
Water extract |
Total phenolics (mg of GAE per g of dry plant) |
1.43 ± 0.12 |
Total flavonoids (mg of RE per g of dry plant) |
0.17 ± 0.05 |
Polysaccharides (mg per g of dry plant) |
3.82 ± 0.21 |
As can be seen from the Table 1, polysaccharides were found to be the most abundant compounds in the extracts. On the basis of the results, the compositions of WEJ displayed that the phenolic acids, flavonoids, polysaccharides were 1.43 mg of GAE per g of dry plant, 0.17 mg of RE per g of dry plant, and 3.80 mg per g of dry plant in the water extract, respectively.
The polyphenolic compound of WEJ was estimated by HPLC analysis. The extracts were also screened for the quantification of twenty selected compounds as following: gallic acid, protocatechuic acid, catechol, p-hydroxybenzoic acid, catechin, chlorogenic acid, vanilla acid, caffeic acid, procyanidin, epicatechol, p-coumaric acid, ferulic acid, rutin, hyperoside, isoquercitrin, kaempferol-3-O-rutinoside, quercitrin, phloretin, cinnamic acid, quercetin.
As shown in Fig. 1 and Table 2, five phenolic acids were identified by comparing the retention times and MS spectrum with the corresponding reference standards. The chemical structures of the phenolic compounds are shown in Table 3. According to data presented in the Table 2, contents found the phenolic acids of WEJ were as follows: protocatechuic acid (17.02 μg per g of dry plant), catechol (5.87 μg per g of dry plant), p-hydroxybenzoic acid (7.46 μg per g of dry plant), vanilla acid (2.95 μg per g of dry plant), p-coumaric acid (1.06 μg per g of dry plant).
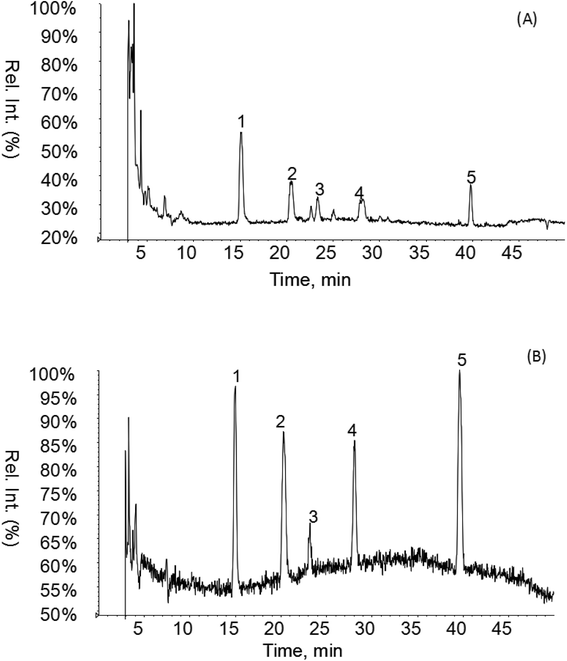 |
| Fig. 1 HPLC-MS chromatograms of the WEJ (A) and the standard (B). Peaks of standard: (1) protocatechuic acid, (2) catechol, (3) p-hydroxybenzoic acid, (4) vanilla acid, (5) p-coumaric acid. | |
Table 2 Characterization and quantities of phenolic compounds in the WEJ (mean ± SD)
No. |
Phenolics and flavonoids |
[M − H]− (m/z) |
Fragment ions |
Concentration (μg per g of dry plant) |
1 |
Gallic acid |
|
|
nd |
2 |
Protocatechuic acid |
153 |
109 |
17.02 ± 0.45 |
3 |
Catechol |
109 |
91 |
5.87 ± 0.26 |
4 |
p-Hydroxybenzoic acid |
137 |
93 |
7.46 ± 0.24 |
5 |
Catechin |
|
|
nd |
6 |
Chlorogenic acid |
|
|
nd |
7 |
Vanilla acid |
167 |
91, 108, 123, 152 |
2.95 ± 0.08 |
8 |
Caffeic acid |
|
|
nd |
9 |
Procyanidin |
|
|
nd |
10 |
Epicatechol |
|
|
nd |
11 |
p-Coumaric acid |
163 |
119 |
1.06 ± 0.02 |
12 |
Ferulic acid |
|
|
nd |
13 |
Rutin |
|
|
nd |
14 |
Hyperoside |
|
|
nd |
15 |
Isoquercitrin |
|
|
nd |
16 |
Kaempferol-3-O-rutinoside |
|
|
nd |
17 |
Quercitrin |
|
|
nd |
18 |
Phloretin |
|
|
nd |
19 |
Cinnamic acid |
|
|
nd |
20 |
Quercetin |
|
|
nd |
Table 3 Chemical structures of phenolic compounds in the WEJ
Compound |
Chemical structure |
Protocatechuic acid |
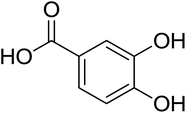 |
Catechol |
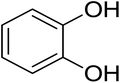 |
p-Hydroxybenzoic acid |
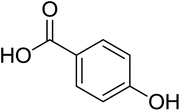 |
Vanilla acid |
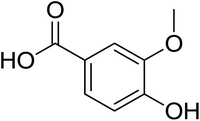 |
p-Coumaric acid |
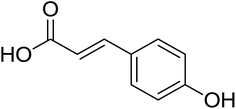 |
3.2. Antioxidant activity
It is considered that antioxidant activities of natural products could not be reasonably evaluated by a single test due to the complexity of natural products and the limitation of each antioxidant assay method.22 There are many methods for the determination of antioxidant properties, the majority of which are based on electron transfer and hydrogen atom donation reactions.23,24 After comprehensive critical assessment of the most frequently used methods, four in vitro antioxidant assays, DPPH, ABTS, ORAC and FRAP assay, differing in their working principles. The sample demonstrated notable antioxidant activity with the levels of 202.18 ± 40, 428.8 ± 40, 60.13 ± 40 and 4670 ± 33 μmol Trolox per mL in the DPPH, ABTS, ORAC and FRAP assays, respectively. The result showed that the WEJ possessed high antioxidant activity which was important to the treatment of liver injury induced by alcohol.
3.3. Body weight and liver index
Feed consumption among all groups had no significant difference. The initial and final body weights, liver weights and liver index of mice were compared and shown in Table 4. There were significant differences of the final bodyweight between the control group and the alcohol group in both prevention and treatment experiments (p < 0.05). Interestingly, administration of bifendate and high dose of WEJ inhibited this elevation significantly. The liver index in the alcohol group was remarkably increased compared to the control group (p < 0.01) and this increase was weakened by giving WEJ and bifendate (p < 0.01).
Table 4 Effects of the WEJ on the body weight, liver weight and liver indexa
Groups |
Body weight (g) |
Liver weight (g) |
Liver index (×100%) |
Initial |
Final |
Each value represents the mean ± SD, n = 6. #p < 0.05 vs. alcohol group. ##p < 0.01 vs. alcohol group. *p < 0.05 vs. control group. **p < 0.01 vs. control group. |
Prevention |
Control |
20.88 ± 1.03 |
35.85 ± 2.46 |
1.55 ± 0.12 |
4.32 |
Alcohol |
21.02 ± 1.21 |
30.23 ± 3.77* |
2.04 ± 0.11** |
6.75** |
WEJ-L + A |
20.97 ± 0.83 |
31.65 ± 3.57 |
1.96 ± 0.04 |
6.19## |
WEJ-M + A |
20.87 ± 0.50 |
36.38 ± 3.61# |
1.73 ± 0.07## |
4.76## |
WEJ-H + A |
20.60 ± 1.13 |
36.90 ± 4.53# |
1.62 ± 0.09## |
4.38## |
Bifendate + A |
21.02 ± 0.68 |
36.22 ± 1.94## |
1.67 ± 0.12## |
4.60## |
Treatment |
Control |
20.73 ± 1.28 |
43.27 ± 2.94 |
1.98 ± 0.10 |
4.58 |
Alcohol |
20.90 ± 0.97 |
37.48 ± 3.42* |
2.36 ± 0.23** |
6.31** |
A + WEJ-L |
20.93 ± 1.25 |
41.75 ± 3.34 |
2.37 ± 0.20 |
5.67 |
A + WEJ-M |
21.13 ± 0.84 |
43.15 ± 4.22# |
2.14 ± 0.13 |
4.96## |
A + WEJ-H |
21.08 ± 1.25 |
43.97 ± 2.18## |
1.97 ± 0.09## |
4.49## |
A + bifendate |
21.00 ± 1.48 |
42.45 ± 1.57## |
2.09 ± 0.23# |
4.92## |
In the prevention group, liver of control group were dark red in color, showing smooth surface and soft texture. The livers of alcohol group were larger, heavier, more pale in color than the control groups. In contrast, the color and surface of the alcohol-induced liver treated with WEJ showed a dose-dependent improvement. The livers of the group treated with the high dose of WEJ were similar to the control group. In the treatment group, the livers of alcohol group were slightly pale in color. There were no significant differences among the control group, the bifendate group and WEJ groups.
3.4. Biochemical indicators of liver function
3.4.1. The level of total cholesterol (TC) and triglycerides (TG). The hepatic steatosis is the earliest and most common alcohol-induced disorder. In the prevention group, there were no statistically significant differences on the level of TC and TG in Table 5. The levels of serum TC and hepatic TG in treatment group were presented. In treatment group, the liver contents of TC and TG (1.63 ± 0.23 mmol L−1 and 383.79 ± 20.15 nmol per g prot) were significantly increased in the alcohol group (p < 0.01), compared with the control group, which were significantly decreased in the high dose group (p < 0.01). However, in terms of the bifendate-treated group, the values of TC and TG had no statistical significance to those of the alcohol group (p > 0.05).
Table 5 Effects of the WEJ on serum TC and hepatic TGa
Groups |
Serum |
Liver |
TC (mmol L−1) |
TG (nmol per g prot) |
Each value represents the mean ± SD, n = 6. #p < 0.05 vs. alcohol group. ##p < 0.01 vs. alcohol group. *p < 0.05 vs. control group. **p < 0.01 vs. control group. |
Prevention |
Control |
1.61 ± 0.08 |
128.32 ± 14.54 |
Alcohol |
1.63 ± 0.23 |
136.02 ± 12.70 |
WEJ-L + A |
1.73 ± 0.22 |
138.52 ± 14.41 |
WEJ-M + A |
1.58 ± 0.19 |
129.71 ± 8.52 |
WEJ-H + A |
1.67 ± 0.17 |
121.98 ± 12.56 |
Bifendate + A |
1.65 ± 0.20 |
124.37 ± 8.40 |
Treatment |
Control |
1.58 ± 0.05 |
128.03 ± 15.18 |
Alcohol |
3.40 ± 0.28** |
383.79 ± 20.15** |
A + WEJ-L |
3.04 ± 0.25# |
328.03 ± 17.87## |
A + WEJ-M |
2.38 ± 0.20## |
238.03 ± 20.34## |
A + WEJ-H |
1.67 ± 0.16## |
143.74 ± 12.82## |
A + bifendate |
3.41 ± 0.22 |
368.20 ± 23.73 |
3.4.2. The levels of ALT, AST and LDH in serum. Both preventive and therapeutic effects of WEJ on the alcohol-induced hepatotoxicity were investigated. It is well known that ALT, AST and LDH are both sensitive serum chemical markers of liver damage. To evaluate the effect of the treatment with the WEJ on chronic alcohol-induced hepatoxicity and the prevention on acute alcohol-induced hepatoxicity, the serum activities of ALT, AST and LDH were obtained by colorimetry methods.The results of alcohol-induced acute liver damage and preventive effects of the WEJ and bifendate on the liver injury are shown in Fig. 2 and 3. There was a significant increase in serum of ALT, AST and LDH (34.78 ± 7.99 IU L−1, 92.17 ± 17.88 IU L−1 and 428.06 ± 51.15 U L−1) in the alcohol group (p < 0.01), whereas the values of them in the control group were only 13.26 ± 7.80 IU L−1, 36.05 ± 5.85 IU L−1 and 236.59 ± 25.37 U L−1, respectively. The prevention of the WEJ on the alcohol-injured mice decreased the levels of ALT, AST and LDH, especially when the dose of WEJ was increased to 400 mg kg−1 (p < 0.01). At the dose of 100 mg kg−1, the activities of ALT, AST and LDH were reduced to 32.68 ± 4.19 IU L−1, 73.85 ± 16.27 IU L−1 and 369.72 ± 18.82 U L−1 while the corresponding enzyme levels were decreased to 13.55 ± 8.64 IU L−1, 32.75 ± 6.28 IU L−1 and 247.68 ± 14.74 U L−1 at the dose of 400 mg kg−1, respectively, which were close to those of the control group.
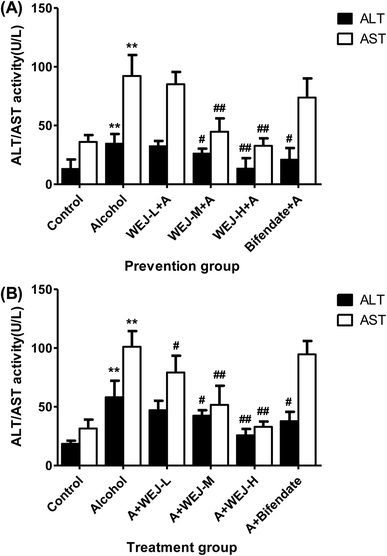 |
| Fig. 2 Preventive (A) and therapeutical (B) effects of different doses of the WEJ on serum ALT and AST activity of mice induced by alcohol. Values are expressed as means ± SD, n = 6. **p < 0.01, compared to control group. #p < 0.05, ##p < 0.01, compared to alcohol group. | |
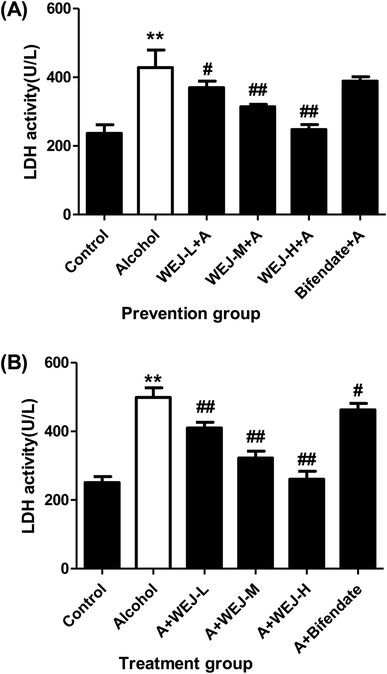 |
| Fig. 3 Preventive (A) and therapeutical (B) effects of different doses of the WEJ on serum LDH activity of mice induced by alcohol. Values are expressed as means ± SD, n = 6. **p < 0.01, compared to control group. #p < 0.05, ##p < 0.01, compared to alcohol group. | |
The therapeutic effects of the WEJ on the chronic liver damage induced by alcohol are shown in Fig. 2 and 3. The serum ALT, AST and LDH were increased from 18.50 ± 2.61 IU L−1, 31.56 ± 7.45 IU L−1 and 250.65 ± 17.59 U L−1 in the control group to 58.13 ± 14.10 IU L−1, 101.10 ± 13.36 IU L−1 and 498.67 ± 28.25 U L−1 in the alcohol group, respectively. Therefore, a significant increase in the hepatic activities of serum ALT, AST and LDH was associated with alcohol exposure (p < 0.01).
The levels of the ALT, AST and LDH induced by alcohol were significantly decreased after administration of the WEJ, especially when the dose of 400 mg kg−1 (the WEJ-H group) was administrated (p < 0.01). The ALT, AST and LDH levels of 25.69 ± 5.45 IU L−1, 32.75 ± 6.28 IU L−1 and 260.97 ± 22.97 U L−1 in the high dose group showed little differences compared with the control group. The results indicated that the WEJ was beneficial to recovery the normal function of the liver and could provide liver protection.
3.4.3. MDA levels and activities of antioxidant enzymes in the liver. The levels of MDA, T-SOD and GSH-Px are indicators of oxidative stress. In order to study the protection and treatment mechanisms of the WEJ against the liver damage induced by alcohol in vivo, the activities of MDA, T-SOD and GSH-Px in liver tissue were examined and shown in Table 6. The activities of SOD and GSH-Px showed a great diminishment (p < 0.01) while the level of MDA was increased (p < 0.01) in the alcohol group compared to those of the control group. The activities of SOD and GSH-Px in mice liver tissues had a dose-dependent rising trend both in the prevention group and the treatment group. The administration of the WEJ significantly elevated hepatic SOD and GSH-Px levels and decreased the level of MDA at the high dose (p < 0.01). The WEJ was conducive to reducing the free radical damage to the liver.
Table 6 Effects of the WEJ on T-SOD, MDA, GSH-Px activities in mice liver induced by alcohola
Groups |
T-SOD (U per mg protein) |
MDA (nmol per mg protein) |
GSH-Px (U per mg protein) |
Each value represents the mean ± SD, n = 6. #p < 0.05 vs. alcohol group. ##p < 0.01 vs. alcohol group. *p < 0.05 vs. control group. **p < 0.01 vs. control group. |
Prevention |
Control |
238.60 ± 4.81 |
2.45 ± 0.53 |
268.42 ± 9.86 |
Alcohol |
158.03 ± 8.17** |
7.48 ± 0.84** |
166.52 ± 8.99** |
WEJ-L + A |
161.70 ± 22.85 |
6.34 ± 0.68# |
181.98 ± 8.28# |
WEJ-M + A |
202.57 ± 17.83## |
4.71 ± 0.36## |
227.96 ± 11.24## |
WEJ-H + A |
228.95 ± 20.75## |
2.46 ± 0.33## |
266.12 ± 22.23## |
Bifendate + A |
198.13 ± 16.71## |
4.79 ± 0.40## |
213.65 ± 13.27## |
Treatment |
Control |
224.38 ± 5.58 |
2.61 ± 0.56 |
277.93 ± 11.36 |
Alcohol |
160.58 ± 7.62** |
8.38 ± 1.64** |
185.07 ± 8.41** |
A + WEJ-L |
171.36 ± 11.43 |
6.46 ± 1.21# |
202.76 ± 14.77# |
A + WEJ-M |
216.15 ± 17.43## |
3.67 ± 0.50## |
257.68 ± 18.30## |
A + WEJ-H |
227.33 ± 18.86## |
3.06 ± 0.47## |
291.86 ± 7.49## |
A + bifendate |
187.76 ± 7.04## |
3.62 ± 0.82## |
250.56 ± 9.97## |
3.5. Effects of WEJ on serum IL-6 and TNF-α levels
Cytokines are protein mediators of inflammation and are produced as a result of the activation of various cellular reactions. As shown in Fig. 4, the serum levels of IL-6 and TNF-α were significantly increased in alcohol-treated mice (p < 0.01) both in the prevention group and the treatment group. The intake of the WEJ significantly suppressed the release of the two cytokines IL-6 and TNF-α (p < 0.01), which might consequently partially diminish inflammatory and endothelial injury of liver.
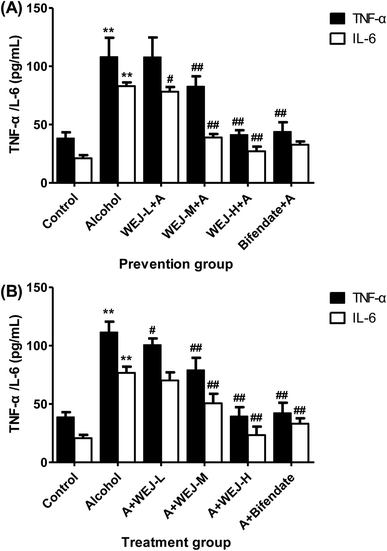 |
| Fig. 4 Preventive (A) and therapeutical (B) effects of different doses of the WEJ on serum IL-6 and TNF-α levels of mice induced by alcohol. Values are expressed as means ± SD, n = 6. **p < 0.01, compared to control group. #p < 0.05, ##p < 0.01, compared to alcohol group. | |
3.6. Western blot analysis of inflammatory related proteins
We tested the effect of the WEJ on the inhibition of inflammatory mediators by western blot analysis. Fig. 5 shows that alcohol up-regulated (p < 0.01) the expression of iNOS and NF-κB p65, whereas the WEJ dose-dependently down-regulated the expression of these inflammatory mediators (p < 0.01).
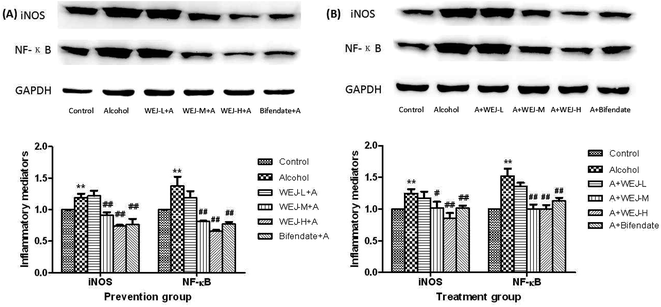 |
| Fig. 5 Preventive (A) and therapeutical (B) effects of different doses of the WEJ on hepatic protein expression of iNOS and NF-κB in mice induced by alcohol. Values are expressed as means ± SD, n = 6. **p < 0.01, compared to control group. #p < 0.05, ##p < 0.01, compared to alcohol group. | |
The high dose of WEJ treatment ameliorated the toxic effect of alcohol and reduced these inflammatory mediators to the normal level. These beneficial effects of WEJ were also found in the bifendate group. Taken together, these results strongly support the capability of WEJ to inhibit proinflammatory mediators in alcohol-induced liver injury.
3.7. Histopathological evaluation
Alcohol-induced liver damage was investigated by observing histopathological changes. The hepatic histopathology results of control, alcohol and WEJ-treated liver slices are shown in Fig. 6 and 7 from the prevention and treatment group, respectively. As shown in Fig. 6A and 7A, liver sections from the control group showed healthy hepatic cells with preserved cellular architecture, central veins and obvious hepatic cell cord. In Fig. 6B, the alcohol group of acute alcohol-induced liver damage showed multiple and extensive areas of hepatocellular necrosis and increase in inflammatory cell infiltration. However, the treatment with the WEJ showed histopathological improvement in a dose-dependent manner (Fig. 6C–E). The group administered with the high dose of WEJ in advance (Fig. 6E) showed markedly attenuated the alcohol-induced liver tissue injury and the normal liver architectures without cell necrosis and inflammatory infiltration, which restored as in the control group. As shown in Fig. 7B, liver sections of the chronic alcohol group showed macrovesicular steatosis, mild degeneration of hepatocytes and hepatic cells arranged in disorder. Compared with the alcohol group, the WEJ-treated group improved in different degree. The hepatocytes of mice treated with the high dose of WEJ (Fig. 7E) showed that fat vacuole reduced obviously and had abundant cytoplasm, which were almost normal in appearance. These results suggest that the WEJ had significant protective and therapeutic effects on impaired hepatic function and hepatic tissue caused by alcohol.
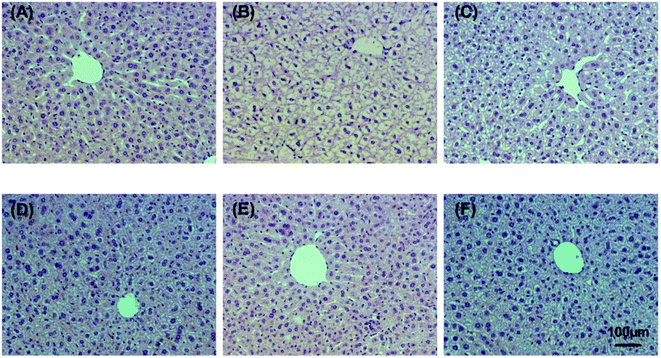 |
| Fig. 6 Histological analysis of the liver samples (hepatic tissue slice by H&E) from experimental mice in the prevention group (×200). Study groups: (A) control, (B) alcohol, (C) WEJ-L + A (100 mg kg−1), (D) WEJ-M + A (200 mg kg−1), (E) WEJ-H + A (400 mg kg−1) and (F) bifendate + A. The mice slices were prepared by using a microtome and stained with hematoxylin–eosin. | |
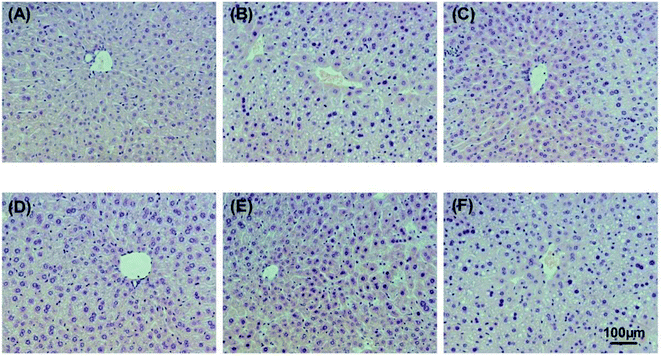 |
| Fig. 7 Histological analysis of the liver samples (hepatic tissue slice by H&E) from experimental mice in the treatment group (×200). Study groups: (A) control, (B) alcohol, (C) A + WEJ-L (100 mg kg−1), (D) A + WEJ-M (200 mg kg−1), (E) A + WEJ-H (400 mg kg−1) and (F) A + bifendate. The mice slices were prepared by using a microtome and stained with hematoxylin–eosin. | |
4. Discussion
The antioxidant properties of natural products have become hot topics for life science researchers. Intensive interest has focused on the water extract since many of them possess strong antioxidant activity. It is well known that phenolic compounds possess the characteristic of antioxidant properties, which play a vital role in the antioxidant defense mechanisms of biological systems.7,22,25,26 According to recent reports, flavonoids possess a series of biological properties, acting on biological systems as antioxidants.27–30 Polysaccharides have received an increased attention due to their antioxidant and hepatoprotective effects.20,31–36
Our results show that the WEJ was a rich source of polyphenolics, flavonoids and polysaccharides which have potential antioxidant activities. Chromatographic and mass spectrometric analyses were employed to identify the major phenolic acids present in the WEJ. There were at least five major phenolic compounds in the WEJ including protocatechuic acid, catechol, p-hydroxybenzoic acid, vanillic acid and p-cournaric acid.
There are different chemical and physical characteristics in different antioxidants. Several studies found that an individual antioxidant can be responded to different radicals or oxidant sources in different manners.24 Single assay can't definitely established the true characteristics of antioxidants in a mixture because of these multiplicities involved in the characteristics and the mechanism of antioxidants.26 Therefore, we selected several methods including DPPH radical scavenging activity, Ferric Reducing Antioxidant Power, ABTS radical cation decolourisation assay and oxygen radical absorbance capacity to study the antioxidant capacity of the WEJ. In this study, the WEJ demonstrated the notable antioxidant activity in all the in vitro tests, indicating the WEJ possessed the high antioxidant activity.
Alcohol is eliminated from the body by metabolizing in the liver mostly,37 and it will promote the incidence of ALD. Previous studies show that most of the drugs have been demonstrated to lead to varying degrees of side effects.38,39 Therefore, searching for both effective and safe components from plants as alternatives to prevent ALD is popular worldwide.
Our experiments have demonstrated significant protective and therapeutical effects of the orally consumed WEJ against hepatic damage induced by alcohol in mice. The reactive oxygen species (ROS) and acetaldehyde induce cell apoptosis and consequently damage the liver, resulting in leakages of AST, ALT, and LDH from the cells and there by leading to the elevation of the levels of enzyme activities.5 AST and ALT are the most various biomarker enzymes to evaluate of the function and integrity of liver cells and LDH is a stable cytoplasm enzyme that is present in all cells.40 Based on the testing data in this study, the levels of AST, ALT and LDH have a significant increase in the serum of alcohol-induced mice compared with the control group both in acute and chronic alcohol-induced liver injury. The results from our study in vivo declare that the WEJ inhibited the activities of AST, ALT and LDH activities in the serum, indicating the protective and therapeutic effects of the WEJ against alcohol-induced liver damage.
There are a variety of mechanisms about alcohol-induced lipid accumulation in hepatocytes. Alcohol may cause fatty acid oxidation reducing in the liver and increasing lipogenesis in hepatocytes.41 In addition, consumption of excessive alcohol in long term can affect the metabolism of TG and cholesterol. It has been reported that alcohol drinking increased hepatic lipid levels in mice.1,42 Therefore, the levels of TC and TG have been studied in involving acute or chronic alcohol-administrated mice. In the study, there was no significant change in the levels of TC and TG in mice given a large amount of alcohol in a week. In the chronic alcohol-administrated mice, which were established by alcohol gavage for 8 weeks, the alcoholic fatty liver resulted in an increase of TC and TG. Our work show that chronic alcohol consumption-induced fatty liver was alleviated by the WEJ treatment.
The MDA level is widely used as a marker of free radical-mediated lipid peroxidation damage.43 The elevated MDA level of liver suggested that enhanced enhancing peroxidation, causing tissue damage and the disability of antioxidant defense mechanisms.24 In the GSH-related antioxidant system, H2O2 and other peroxides reduced catalyzed by GSH-Px. SOD is also an significant substance which is conducive to the clearance of the superoxide anion radicals and exercise an effect on the formation of H2O2.44 Therefore, the levels of MDA, GSH-Px and SOD in hepatic tissues play important role in liver injury. In this study, alcohol leaded to decrease in the activities of T-SOD, GSH-Px in liver. Whereas, there was increased the MDA content as compared with the control group. The significantly reduced in MDA, elevated T-SOD and GSH-Px levels were found in the WEJ-treated group compared with the alcohol group.
Our results show that the WEJ could not only reduce the serum ALT, AST, LDH activities and TC and TG levels in serum and liver, but also decrease MDA in liver tissue. In addition, the increase of liver tissue SOD and GSH-Px activities of the alcohol-induced liver tissue damage were alleviated by the WEJ effectively.
It is reported that alcohol evoked the release of inflammatory cytokines such as IL-6, TNF-α of the liver, which intensified hepatic inflammation and cell apoptosis.45,46 We found that the WEJ intake effectively lowered hepatic IL-6, TNF-α production, which contributed to diminished hepatic inflammatory stress.
On the other hand, NO derived from iNOS plays pivotal roles in the pathogenesis of acute and chronic hepatic inflammation.47,48 Besides acting as inflammatory enhancers, excessive NO impairs hepatic insulin signaling.49 Thus, the agent with the capability to decrease NO production in the liver not only mitigates hepatic inflammatory injury but also avoids the occurrence of fatty liver or diabetes. Our present study found that the WEJ intake declined hepatic activity and protein expression of iNOS, which in turn limited the production of NO.
NF-κB is important signaling pathways responsible for the formation of oxidative and inflammatory reactants.50,51 It is documented that alcohol could activate these pathways, and promote the expression of inflammatory mediators such as iNOS and COX-2, which in turn accelerate the production of down-stream factors including NO, IL-6 and TNF-α in the liver.52,53 Our western blot data reveal that the WEJ intake down-regulated hepatic protein expression of NF-κB p65 in alcohol treated mice. Since NF-κB pathways had been declined, the lower hepatic expression and activity of iNOS could be explained. Consequently, it was also reasonable to observe lower hepatic levels of IL-6 and TNF-α in WEJ treated mice. These findings indicate that hepatic protective effects from dietary WEJ could be ascribed to this triterpene suppressed NF-κB pathways, which in turn decreased the production of down-stream oxidative and inflammatory factors, and finally alleviated alcohol evoked hepatotoxicity. The WEJ pre-intake led to its deposit in liver, and markedly decreased subsequent alcohol elicited acute hepatic oxidative and inflammatory stress via suppressing NF-κB pathways.
Histological examination of mice liver treated with alcohol showed significant hepatotoxicity characterization, such as hepatocellular necrosis, inflammatory cell infiltration in acute alcohol-induced mice and macrovesicular steatosis, mild degeneration of hepatocytes in chronically alcohol-induced mice. However, administration of the WEJ significantly decreased the damage in liver, suggesting that the WEJ had protection and treatment effects against alcohol-induced liver injury.
The water extract of the jujube showed a remarkable antioxidant activity in almost all test systems, which was in consistent with the phenolic compositions that had been identified. This study identified protective and therapeutic effects of the WEJ on acute and chronic alcohol-induced liver injury in mice which were dose-dependent by oral consumption. Our results reveal that WEJ prevented alcohol-induced liver injury via anti-oxidative stress and anti-inflammation. These findings might be beneficial for developing a natural agent for preventing and remedying alcohol-induced liver injury.
Acknowledgements
This work was supported by the funds from National “Major Science and Technology Project–Prevention and Treatment of AIDS, Viral Hepatitis, and Other Major Infectious Diseases” (Grant #2013ZX10005004), Major Project of Science and Technology of Shandong Province (Grant #2015ZDJS04001), Science & Technology Enterprise Technology Innovation Fund of Jiangsu Province (Grant #BC2014172), Small & Medium Enterprise Technology Innovation Project of Lianyungang City (Grant #CK1333).
References
- J. Xiang, W. Zhu, Z. Li and S. Ling, Food Funct., 2012, 3, 628–634 CAS.
- G. Liu, X. Liu, Y. Zhang, F. Zhang, T. Wei, M. Yang, K. Wang, Y. Wang, N. Liu, H. Cheng and Z. Zhao, Int. J. Biol. Macromol., 2015, 76, 169–175 CrossRef CAS PubMed.
- B. Sangeetha and S. Krishnakumari, Int. J. Pharma Bio Sci., 2010, 1, 173–185 Search PubMed.
- V. L. Massey and G. E. Arteel, Front. Physiol., 2012, 3, 193 CAS.
- S. L. Yan, H. T. Yang, H. L. Lee and M. C. Yin, Food Chem. Toxicol., 2014, 74, 149–155 CrossRef CAS PubMed.
- Y. Lu and A. I. Cederbaum, Free Radical Biol. Med., 2008, 44, 723–738 CrossRef CAS PubMed.
- N. Cheng, B. Du, Y. Wang, H. Gao, W. Cao, J. Zheng and F. Feng, Food Funct., 2014, 5, 900–908 CAS.
- H. I. Soliman, G. Abd and E. M. Hegazi, Life Sci. J., 2013, 10, 573–582 Search PubMed.
- Y. Li, C. Xu, X. Lin, B. Cui, R. Wu and X. Pang, PLoS One, 2014, 9, e106438 Search PubMed.
- Q. H. Gao, J. G. Yu, C. S. Wu, Z. S. Wang, Y. K. Wang, D. L. Zhu and M. Wang, PLoS One, 2014, 9, e88912 Search PubMed.
- C. Qu, S. Yu, H. Jin, J. Wang and L. Luo, Spectrochim. Acta, Part A, 2013, 114, 339–343 CrossRef CAS PubMed.
- A. Chi, C. Kang, Y. Zhang, L. Tang, H. Guo, H. Li and K. Zhang, Carbohydr. Polym., 2015, 122, 189–196 CrossRef CAS PubMed.
- C. F. Chen, J. F. Lee, D. Wang, C. Y. Shen, K. L. Shen and M. H. Lin, Transplant. Proc., 2010, 42, 741–743 CrossRef CAS PubMed.
- Y. Wang, F. Tang, J. Xia, T. Yu, J. Wang, R. Azhati and X. D. Zheng, Food Chem., 2011, 125, 835–840 CrossRef CAS.
- S. H. Choi, J. B. Ahn, N. Kozukue, C. E. Levin and M. Friedman, J. Agric. Food Chem., 2011, 59, 6594–6604 CrossRef CAS PubMed.
- X. Kou, Q. Chen, X. Li, M. Li, C. Kan, B. Chen, Y. Zhang and Z. Xue, Food Chem., 2015, 173, 1037–1044 CrossRef CAS PubMed.
- H.-x. Zhao, H.-s. Zhang and S.-f. Yang, Food Science and Human Wellness, 2014, 3, 183–190 CrossRef.
- F. Daneshmand, H. Zare-Zardini and L. Ebrahimi, Nat. Prod. Res., 2013, 27, 2292–2296 CrossRef CAS PubMed.
- R. Hoshyar, Z. Mohaghegh, N. Torabi and A. Abolghasemi, Asian Pac. J. Reprod., 2015, 4, 116–122 CrossRef.
- D. Wang, Y. Zhao, Y. Jiao, L. Yu, S. Yang and X. Yang, Carbohydr. Polym., 2012, 88, 1453–1459 CrossRef CAS.
- C. T. Lam, P. H. Chan, P. S. Lee, K. M. Lau, A. Y. Kong, A. G. Gong, M. L. Xu, K. Y. Lam, T. T. Dong and H Lin, J. Chromatogr. B: Anal. Technol. Biomed. Life Sci., 2015, 1026, 254–262 CrossRef PubMed.
- K. Saravanan and S. M. Aradhya, Food Funct., 2011, 2, 603–610 CAS.
- I. Siro, E. Kapolna, B. Kapolna and A. Lugasi, Appetite, 2008, 51, 456–467 CrossRef PubMed.
- R. L. Prior, X. Wu and K. Schaich, J. Agric. Food Chem., 2005, 53, 4290–4302 CrossRef CAS PubMed.
- G. Borges, W. Mullen and A. Crozier, Food Funct., 2010, 1, 73–83 CAS.
- A. K. Tiwari, K. S. Reddy, J. Radhakrishnan, D. A. Kumar, A. Zehra, S. B. Agawane and K. Madhusudana, Food Funct., 2011, 2, 521–528 CAS.
- M. Hidalgo, C. Sánchez-Moreno and S. de Pascual-Teresa, Food Chem., 2010, 121, 691–696 CrossRef CAS.
- N. Andarwulan, R. Batari, D. A. Sandrasari, B. Bolling and H. Wijaya, Food Chem., 2010, 121, 1231–1235 CrossRef CAS.
- J. C. P. Calado, P. A. Albertão, E. A. d. Oliveira, M. H. S. Letra, A. C. H. Frankland Sawaya and M. C. Marcucci, Agric. Sci., 2015, 06, 426–435 CAS.
- E. T. Olayinka, A. Ore, O. A. Adeyemo, O. S. Ola, O. O. Olotu and R. C. Echebiri, Antioxidants, 2015, 4, 304–321 CrossRef CAS PubMed.
- S. Li and N. P. Shah, Food Chem., 2014, 165, 262–270 CrossRef CAS PubMed.
- D. Qiao, C. Ke, B. Hu, J. Luo, H. Ye, Y. Sun, X. Yan and X. Zeng, Carbohydr. Polym., 2009, 78, 199–204 CrossRef CAS.
- Y.-H. Tseng, J.-H. Yang and J.-L. Mau, Food Chem., 2008, 107, 732–738 CrossRef CAS.
- D. Luo and B. Fang, Carbohydr. Polym., 2008, 72, 376–381 CrossRef CAS.
- D. Liang, Q. Zhou, W. Gong, Y. Wang, Z. Nie, H. He, J. Li, J. Wu, C. Wu and J. Zhang, J. Ethnopharmacol., 2011, 136, 316–321 CrossRef CAS PubMed.
- X. Lu, Y. Zhao, Y. Sun, S. Yang and X. Yang, Food Chem., 2013, 141, 3415–3423 CrossRef CAS PubMed.
- S. Zakhari, Alcohol Res. Health, 2006, 29, 245–254 Search PubMed.
- Y. Zhao, X. Yang, D. Ren, D. Wang and Y. Xuan, Food Funct., 2014, 5, 1771–1778 CAS.
- P. Muriel and Y. Rivera-Espinoza, J. Appl. Toxicol., 2008, 28, 93–103 CrossRef CAS PubMed.
- Y. Zhang, F. Zhang, K. Wang, G. Liu, M. Yang, Y. Luan and Z. Zhao, Chem.–Biol. Interact., 2016, 249, 71–77 CrossRef CAS PubMed.
- C. C. Tang, H. P. Huang, Y. J. Lee, Y. H. Tang and C. J. Wang, Food Chem. Toxicol., 2013, 62, 786–796 CrossRef CAS PubMed.
- O. Wang, Q. Cheng, J. Liu, Y. Wang, L. Zhao, F. Zhou and B. Ji, Food Funct., 2014, 5, 3018–3025 CAS.
- C. Chen, X. Yu, H. Lu, D. Xiao, W. Mao and L. Li, Mol. Med. Rep., 2013, 7, 401–405 CAS.
- Y. W. Hsu, C. F. Tsai, W. K. Chen and F. J. Lu, Food Chem. Toxicol., 2009, 47, 2281–2288 CrossRef CAS PubMed.
- G. Ji, Q. Yang, J. Hao, L. Guo, X. Chen, J. Hu, L. Leng and Z. Jiang, Int. Immunopharmacol., 2011, 11, 762–768 CrossRef CAS PubMed.
- A. M. Miller, H. Wang, O. Park, N. Horiguchi, F. Lafdil, P. Mukhopadhyay, A. Moh, X. Y. Fu, G. Kunos, P. Pacher and B. Gao, Alcohol.: Clin. Exp. Res., 2010, 34, 719–725 CrossRef CAS PubMed.
- R. Jayasooriya, C. H. Kang, Y. H. Cho, W. S. Ko, I. W. Choi and G. Y. Kim, Trop. J. Pharm. Res., 2011, 10, 402–411 Search PubMed.
- P. Enkhbaatar, J. Parkinson and D. L. Traber, Role of iNOS-derived Excessive Nitric Oxide in the Pathogenesis of Acute Lung Injury, Springer, New York, 2005, pp. 41–48 Search PubMed.
- J. A. J. Martyn, M. Kaneki and S. Yasuhara, Anesthesiology, 2008, 109, 137–148 CrossRef PubMed.
- H. Y. Park, G. Y. Kim, J. W. Hyun, H. J. Hwang, N. D. Kim, B. W. Kim and Y. H. Choi, Int. J. Mol. Med., 2012, 29, 1146–1152 CAS.
- E. Shankar, E. V. Vykhovanets, O. V. Vykhovanets, G. T. MacLennan, R. Singh, N. Bhaskaran, S. Shukla and S. Gupta, Prostate, 2012, 72, 233–243 CrossRef CAS PubMed.
- G. L. Tipoe, E. C. Liong, C. A. Casey, T. M. Donohue, P. K. Eagon, H. So, T. M. Leung, F. Fogt and A. A. Nanji, Alcohol.: Clin. Exp. Res., 2008, 32, 669–682 CrossRef CAS PubMed.
- J. I. Beier and C. J. McClain, Biol. Chem., 2010, 391, 1249–1264 CrossRef CAS PubMed.
|
This journal is © The Royal Society of Chemistry 2017 |
Click here to see how this site uses Cookies. View our privacy policy here.