DOI:
10.1039/C6RA28525B
(Paper)
RSC Adv., 2017,
7, 23680-23686
Design and synthesis of 1,2,3-triazole–etodolac hybrids as potent anticancer molecules†
Received
21st December 2016
, Accepted 12th April 2017
First published on 2nd May 2017
Abstract
A series of novel 1,2,3-triazole–etodolac hybrids (6a–l) were designed and synthesized as potent anti-cancer molecules. The synthesis strongly relied on Huisgen's 1,3-dipolar cycloaddition between etodolac azide 3 and substituted terminal alkynes 5a–l. The use of CH2Cl2 as a co-solvent with H2O increased the reaction rate and provided the corresponding 1,2,3-triazole–etodolac hybrids (6a–l) in excellent yields compared to other organic co-solvent systems. All the compounds were screened for their in vitro anticancer activity against human A549 cell lines and compounds 6e, 6f, 6h, 6j, and 6l were found to be the best anti-cancer molecules as compared to the marketed drug doxorubicin.
Cancer is a multi-factorial and dreadful disease characterized by uncontrolled growth of cells and spread of abnormal cells in the body.1 It is the most common disease in the modern era and causes the most deaths (one in eight deaths) worldwide. Because of enduring concern across the globe, there is an ever-increasing requirement for safe and effective anti-tumour agents to fight against cancer.2a–h
1,2,3-Triazole is one of the extensively used scaffolds in pharmaceuticals and exhibits a wide range of biological activities including significant anti-cancer profiles against many human cancer cell lines.3,4a–c,5a–f These heterocycles are bestowed with special properties like moderate dipole character, hydrogen bonding capability, rigidity, stability and can be constructed through click chemistry approach in a facile manner.6 1,2,3-Triazoles conjugated with other pharmacophores (triazole-hybrids) known to possess potent anti-cancer activities. In this context, Kamal et al. described a new class of triazole chalcone-pyrrolo[1,4]benzodiazepine (PBD) hybrids as inhibitors of cyclin D1 and NF-kB protein (Fig. 1) which showed significant G1 cell cycle arrest.3
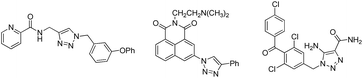 |
| Fig. 1 Few hybrid triazoles. | |
Liu et al. identified promising candidates with a broad spectrum of anti-cancer activities and low cytotoxicity against the normal cell lines HEK-293 by the synthesis of novel 1,2,3-triazole–dithiocarbamate hybrids and some of them were more potent than the well-known anti-cancer drug 5-fluorouracil4a–c (Fig. 2).
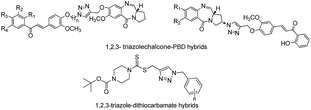 |
| Fig. 2 Hybrid anti-cancer molecules with triazole skeleton. | |
On the other hand nonsteroidal anti-inflammatory drugs (NSAIDs) are the cyclooxygenase (COX) inhibitors,7a–f and recent findings advocate that they possess anti-cancer effects through a COX-2-independent mechanism.7d Etodolac is a COX-2 inhibitor with analgesic and antipyretic properties and is approved by US FDA.7f In recent years the antitumor activity of etodolac analogues against many types of cancer, such as urogenital system cancers, Burkitt's lymphoma, multiple myeloma, chronic lymphocytic leukemia, and prostate cancer has been described.7e In view of the pharmacological significance of etodolac and triazole derivatives with reference to anticancer activity, we have attempted the synthesis of a new series of 1,2,3-triazole–etodolac hybrids and evaluated their anti-cancer properties (Fig. 3).
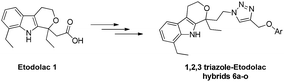 |
| Fig. 3 Synthetic strategy. | |
Results and discussions
Chemistry
The synthesis of a series of 1,2,3-triazole–etodolac hybrids (6a–l) carried out by using the Cu(I)-catalyzed Huisgen's 1,3-dipolar cycloaddition reaction is presented in Scheme 1.
 |
| Scheme 1 Proposed Cu(I)-catalyzed Huisgen's 1,3-dipolar cycloaddition reaction. | |
The synthetic strategy to achieve the novel hybrid template was initiated from the reduction of acid functionality of commercially available etodolac 1 by using LAH (lithium aluminum hydride) to give the primary alcohol 2 in 92% yield.8 The newly formed hydroxyl was transformed into azide 3 using DPPA (diphenylphosphoryl azide), TPP (triphenylphosphine) and DIAD (diisopropyl azodicarboxylate) in THF. The reaction mixture was stirred for 12 h at room temperature. The whole reaction mixture was concentrated to get the residue, which was extracted with EtOAc. The organic layer dried over anhydrous Na2SO4 filtered and evaporated. The crude reaction mixture was purified by silica gel column chromatography using EtOAc/hexane (10%) as an eluent to provide the corresponding azide 3 in 86% yield.9 Finally, the synthesis of triazole–etodolac hybrid 6a was attempted by treating the azide 3 with the terminal alkyne 5a in the presence of CuSO4·5H2O and L-sodium ascorbate in tBuOH
:
H2O (1
:
1) at room temperature and the desired compound 6a was formed in trace amount. The prolonged reaction time and high temperature also did not improve the outcome of the reaction. Later the reaction was performed in various organic co-solvents to improve the reaction outcome14 and the synthesis of 6a was successfully accomplished in 1
:
1 DCM
:
H2O solvent system10 with excellent yield (86%) and the results are summarized in Table 1 (Scheme 2).
Table 1 Identification of co-solvent for the system of 6a
Entry |
Substrate |
Product |
Solvent |
Co-solvent |
Yield (%) |
Time (h) |
1 |
5a |
6a |
H2O |
CH3CN |
46 |
12 |
2 |
5a |
6a |
H2O |
DMSO |
43 |
9 |
3 |
5a |
6a |
H2O |
THF |
54 |
10 |
4 |
5a |
6a |
H2O |
t-BuOH |
42 |
8 |
5 |
5a |
6a |
H2O |
DMF |
58 |
12 |
6 |
5a |
6a |
H2O |
DCM |
86 |
8 |
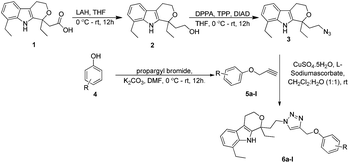 |
| Scheme 2 Synthesis of 1,2,3-triazole–etodolac hybrids 6a–l. | |
After optimizing the reaction conditions, we have preceded to synthesis the library of triazole–etodolac hybrids (6a–l) to prove the generality of the adopted synthetic procedure. For the purpose, we have synthesized desired alkynes (5a–l) by treating the commercially available phenols (4a–l) with propargyl bromide and K2CO3 (Scheme 2) in good yields.11 Finally, the Huisgen's 1,3-dipolar cycloaddition5c,12 reaction was employed to react the azide 3 and the alkynes 5a–l to furnish the hybrid molecules 6a–l in excellent yields and the results were summarized in Table 2.
Table 2 Yields for the Cu-catalyzed synthesis of 1,2,3-triazole–etodolac derivatives (6a–l)
S. No. |
1-Alkynes (5a–l) |
Product (6a–l) |
Time |
Yield (%) |
1 |
 |
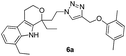 |
8 h |
86 |
2 |
 |
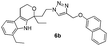 |
6 h |
92 |
3 |
 |
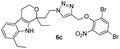 |
8 h |
87 |
4 |
 |
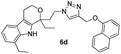 |
6 h |
92 |
5 |
 |
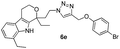 |
6 h |
89 |
6 |
 |
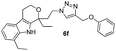 |
6 h |
93 |
7 |
 |
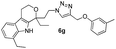 |
8 h |
92 |
8 |
 |
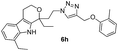 |
6 h |
93 |
9 |
 |
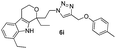 |
6 h |
90 |
10 |
 |
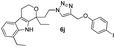 |
6 h |
94 |
11 |
 |
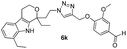 |
7 h |
92 |
12 |
 |
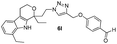 |
7 h |
94 |
Biology
Cytotoxicity was measured using the MTT [3-(4,5-dimethylthiazol-2-yl)-2,5-diphenyl tetrazolium bromide] assay, against A549 lung cancer cell lines which is a colorimetric assay.13a MTT (3-[4,5-dimethylthiazol-2-yl]-2,5-diphenyltetrazolium bromide) is a water-soluble yellow color tetrazolium salt and is converted to an insoluble purple formazan by cleavage of the tetrazolium ring by succinate dehydrogenase which is present in the mitochondria. The formazan product is impermeable to the cell membranes and therefore it accumulates in healthy cells. Since the reduction of MTT can only occur in metabolically active cells, the intensity of the purple color is a measure of the viability of the cells. The intensity can be measured by spectrophotometer after dissolving formazan crystal in DMSO.13b
The cells (5 × 103) were seeded in each well containing 0.1 mL of medium in 96 well plates. After overnight incubation at 37 °C in 5% CO2, the cells were treated with 100 μL of different test concentrations of test compounds at identical conditions with three replicates each. The cytotoxic effect of all the synthesized molecules was evaluated using doxorubicin a widely used anthracycline antibiotic and with IC50 values of 3.3 μM on A549 as the standard reference at 25 μM doses. The cell viability was assessed after 48 h, by adding 10 μL of MTT (5 mg mL−1) per well. The plates were incubated at 37 °C for additional 2–4 hours. The medium was discarded and the formazan violet crystals, which were coloured for the viable cells, were dissolved in 100 μL of DMSO. The rate of color formation was measured at 570 nm in a spectrophotometer (Spectra MAX Plus; Molecular Devices; supported by SOFT max PRO-5.4). The percent inhibition of cell viability was determined with reference to the control values (without test compound). The data were normalised with the lowest and highest values and subjected to non-linear regression analysis to obtain log (concentration) vs. normalised expression (variable slope) using graph pad prism 6. The IC50 (inhibition of cell growth) concentrations were further obtained using the graph pad prism 6.
It is apparent from the results that compounds 6e, 6f, 6h, 6j, 6l are found to be potent on lung cancer cell lines than the other (Fig. 4, Table 3)
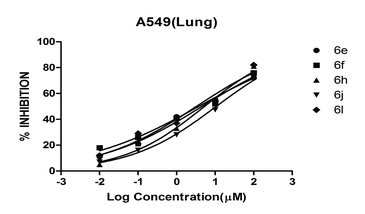 |
| Fig. 4 Log concentration–response curve of the most active compounds on A549 cancer cell lines. | |
Table 3 Determination of IC50 of selected compounds against cancer cell linesa
IC50b (μM) |
Compound |
6e |
6f |
6h |
6j |
6l |
Standard drug used for in vitro activity studies is doxorubicin and its IC50 3.3 μM. IC50 (μM) is 50% inhibitory concentration and values are the means of three experiments each done in duplicate. |
A549 |
4.50 ± 0.5 |
3.65 ± 0.4 |
5.38 ± 0.4 |
10.71 ± 0.3 |
3.29 ± 0.7 |
The test compounds with >70% inhibition on cancer cell lines were chosen for the IC50 prediction on lung cancers. It was interesting to note that compounds 6e, 6f, 6h, 6j and 6l found to possess more effect at the same dose compared to doxorubicin against A549 (lung) cancer cell line. Next, dose–response studies of active compounds were performed to calculate IC50 value against the same cell lines. Among the whole series of title compounds (6a–l), compound 6l was found to be the most active compound with an IC50 value of 3.29 ± 0.7 μM on lung cancer cell line (A549). The second-best compound identified in the series was 6f with IC50 3.65 ± 0.4 value. The IC50 values of active compounds are summarized in Table 2.
Similar scaffolds have been reported earlier for their potent anticancer activities.14a–c This prompted us to investigate the possible mechanism of the synthesized compounds by performing the molecular docking studies into the binding pocket of the human topoisomerase-II ATPase co-crystallized with AMP–PNP complex (TPII) [PDB:1ZXM].15 The water molecules, substrate cofactors were removed from the PDB file and the missing hydrogen atoms were added to the system followed by energy minimization using OPLS 2005 force field with Polak–Ribiere Conjugate Gradient (PRCG) algorithm. The ligands were built using the builder feature in Maestro and each structure was prepared for docking using Ligprep 2.7 followed by molecular mechanics energy minimization using Macromodel 10.1. These geometrically optimized structures were used for Glide (grid-based ligand docking with energetics) docking using the “Extra Precision” (XP) mode of Glide 6.0.14 As depicted in (Fig. 5b), compound 6l showed hydrophobic interactions with active site residues Tyr 64, Ile 109, Pro 111, Val 236, Ala 237, Val 240, Pro 263, Leu 257 and interaction with other active site residues such as Asp 65, Glu 66, Asp 67, Lys 233, Lys 261 were also observed. Additionally, hydrogen bonding interactions with Tyr 244 and Asn 258 were noticed (Fig. 5c). These results also indicate that the compound 6l is bound well within the binding site pocket of doxorubicin and almost similar binding pattern was identified. The hydrogen bonding interaction with active site residues Tyr 244 and Asn 258 was in common (Fig. 5d).
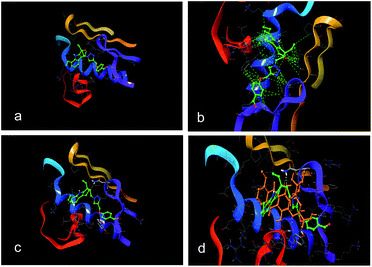 |
| Fig. 5 (a) Three-dimensional diagram displays the binding poses for compound 6l interactions at the active site of TP II. (b) Key residues within a 4.5 Å sphere of 6l in the binding pocket are shown. (c) Hydrogen bonding of 6l with Tyr 244 and Asn 258 in the active site. (d) Compound 6l overlaid with doxorubicin (orange). Yellow dotted lines represent H-bonding with amino acid backbone respectively. | |
Conclusion
A series of 1,2,3-triazole–etodolac hybrids (6a–l) have been designed and synthesized by employing Cu(I)-catalyzed Huisgen 1,3-dipolar cycloaddition reaction. The in vitro anti-cancer activity of all the synthesized compounds against human A549 cancer cell lines was evaluated and it is found that the compounds 6e, 6f, 6h, 6j, and 6l have shown promising results in comparison with the marketed drug doxorubicin. In the series, 6l has shown very good anticancer activity with an IC50 value of 3.29 ± 0.7 μM and second-best compound was 6f with an IC50 value of 3.65 ± 0.4 μM. Therefore, these compounds can serve as a promising lead candidate for further study.
Experimental
General information
The reagents and materials including etodolac were of the highest commercially available purity grade and were used without any further purification. Flash column chromatography was performed on Merck silica gel 60–120 mesh. Compounds were visualized with UV light. 1H NMR spectra were recorded on Bruker Avance 400 MHz spectrometer and 13C NMR spectra were recorded on Bruker Avance 100 MHz spectrometer in CDCl3 as a solvent, with TMS as an internal standard. Multiplicities were denoted by s (singlet), brs (broad singlet), d (doublet), t (triplet), q (quartet), dd (double doublet) and m (multiplet). IR spectra were recorded on Perkin Elmer spectrophotometer by using KBr pellets. Melting points were determined in open capillary tubes. Reactions were monitored by thin-layer chromatographic (TLC) technique using silica gel plates. Phosphate-buffered saline (PBS), antibiotic mixture, Roswell Park Memorial Institute (RPMI) 1640 medium, F-12K medium and new born calf serum (NBCS) were obtained from Hi Media Laboratories Pvt. Ltd. MTT (3-(4,5-dimethylthiazole-2-yl)-2,5-diphenyl tetrazolium bromide) was procured from Sigma-Aldrich (Bangalore, India). Cell line that was used for testing in vitro cytotoxicity included A549 derived from human lung epithelial cells (ATCC No. CCL-185), which was procured from American Type Culture Collection, Manassas, VA, USA.
2-(1,8-Diethyl-1,3,4,9-tetrahydropyrano[3,4-b]indol-1-yl)ethanol (2)
A stirred solution of LiAlH4 (0.38 g, 9.93 mmol) in dry THF (35 mL), was added to a solution of 2-(1,8-diethyl-1,3,4,9-tetrahydropyrano[3,4-b]indol-1-yl)acetic acid 1 (1.42 g, 4.96 mmol) in dry THF (14 mL) at 0 °C. The mixture was stirred overnight at room temperature. Then reaction mixture was quenched with saturated Na2SO4 solution at 0 °C, the mixture was filtered through Celite, and washed with ethyl acetate. The solvent was removed to yield pure product 2 (0.33 g, 92%).
1-(2-Azidoethyl)-1,8-diethyl-1,3,4,9-tetrahydropyrano[3,4-b]indole (3)
To a stirred solution of 2-(1,8-diethyl-1,3,4,9-tetrahydropyrano[3,4-b]indol-1-yl)ethanol 2 (0.5 g, 1.75 mmol) in THF (10 mL), were DPPA (diphenylphosphoryl azide) (3.50 mmol), DIAD (di isopropyl azodicarboxylate) (5.26 mmol) and triphenylphosphine (5.26 mmol) added at 0 °C. The reaction mixture was stirred overnight under nitrogen saturated atmosphere. After completion of reaction. The reaction mixture was poured in 100 mL water and thoroughly extracted with ethyl acetate (3 × 50 mL). The organic layer was dried over Na2SO4, filtered and evaporation under reduced pressure. The resulting residue was purified by flash chromatography using petroleum ether
:
ethyl acetate (90
:
10) as the eluent, to afford the 1-(2-azidoethyl)-1,8-diethyl-1,3,4,9-tetrahydropyrano[3,4-b]indole 3 (0.45 g, 86%) as a white solid. Chemical formula: C17H22N4O.
1,4-Dimethyl-2-(prop-2-yn-1-yloxy)benzene (5a)
A mixture of 2,5-dimethylphenol (0.5 g, 4.09 mmol), propargyl bromide (0.44 mL, 4.91 mmol) and K2CO3 (0.84 g, 6.13 mmol) was stirred in dimethylformamide (20 mL) at room temperature. After completion of the reaction (TLC monitoring), the reaction mixture was diluted with EtOAc (100 mL) and washed with water (3 × 50 mL) times. The organic phase was dried over with anhydrous Na2SO4 and concentrated to dryness in vacuo. The obtained crude crystalline was purified by column chromatography to obtain a pure white solid 5a.
1-(2-(4-((2,5-Dimethylphenoxy)methyl)-1H-1,2,3-triazol-1-yl)ethyl)-1,8-diethyl-1,3,4,9-tetrahydropyrano[3,4-b]indole (6a)
To a solution of 1,4-dimethyl-2-(prop-2-yn-1-yloxy)benzene (54 mg, 0.33 mmol) 5a and 1-(2-azidoethyl)-1,8-diethyl-1,3,4,9-tetrahydropyrano[3,4-b]indole 3 (100 mg, 0.33 mmol) in CH2Cl2 (0.7 mL) and H2O (0.7 mL) were added CuSO4·5H2O (0.08 mg, 0.0033 mmol) and sodium ascorbate (6.6 mg, 0.033 mmol). The resulting solution was stirred for 8 h at room temperature. The reaction mixture was diluted with CH2Cl2 (5 mL) and H2O (5 mL). The organic layer was separated, dried over Na2SO4, and concentrated. The residue was purified by flash column chromatography (hexane/EtOAc, 2
:
1) to give 1,2,3-triazole 6a as a pale green solid. Yield 86%; mp 140 °C; chemical formula: C28H34N4O2; 1H NMR (400 MHz, CDCl3): δ 0.92 (t, J = 7.09, 3H), 1.25 (t, J = 7.3, 3H), 1.76–1.86 (m, 1H), 1.92–2.04 (m, 1H), 2.07 (s, 3H), 2.26 (s, 3H), 2.45–255 (m, 1H), 2.56–2.65 (m, 1H), 2.74–2.88 (m, 4H), 3.95–4.05 (m, 1H), 4.08–4.20 (m, 1H), 4.20–4.35 (m, 1H), 4.43–4.52 (m, 1H), 4.72 (d, J = 12.22 Hz, 1H), 4.88 (d, J = 11.98 Hz, 1H), 6.61–6.74 (m, 2H), 6.88–7.18 (m, 2H), 7.22–7.40 (m, 3H), 8.22 (s, 1H); 13C NMR (125 MHz, CDCl3): δ 7.85, 13.68, 15.83, 21.41, 21.96, 23.91, 31.53, 38.04, 46.17, 60.47, 61.82, 75.44, 108.88, 112.44, 115.89, 119.91, 120.57, 121.41, 123.74, 126.21, 126.66, 129.79, 130.46, 135.00, 135.30, 136.57, 144.35, 156.23; HRMS: m/z [M + H]+ calcd for C28H35N4O2 459.2760, found: 459.2786; IR (KBr, cm−1): 3243, 2958, 2914, 2870, 1714, 1593, 1451, 1259, 1128, 1051, 958, 810, 739.
1,8-Diethyl-1-(2-(4-((naphthalen-2-yloxy)methyl)-1H-1,2,3-triazol-1-yl)ethyl)-1,3,4,9-tetrahydropyrano[3,4-b]indole (6b)
Pale brown solid; yield 92%; mp 190 °C; chemical formula: C30H32N4O2; 1H NMR (400 MHz, CDCl3): δ 0.96 (t, J = 7.09, 3H), 1.3 (t, J = 7.3, 3H), 1.82–1.89 (m, 1H), 1.9–2.1 (m, 1H), 2.45–2.68 (m, 2H), 2.75–3.0 (m, 4H), 3.9–4.15 (m, 2H), 4.3–4.45 (m, 2H), 4.85 (d, J = 12.22 Hz, 1H), 5.2 (d, J = 11.98 Hz, 1H), 6.84–7.15 (m, 1H), 7.18–7.29 (m, 2H), 7.3–7.6 (m, 6H), 7.75–7.95 (m, 2H), 8.2 (s, 1H); 13C NMR (100 MHz, CDCl3): δ 7.79, 13.64, 22.40, 23.85, 31.43, 37.96, 46.11, 60.38, 61.43, 75.34, 107.06, 108.81, 115.84, 118.72, 119.84, 120.46, 123.58, 123.78, 126.14, 126.37, 126.62, 126.81, 127.56, 129.05, 129.41, 129.75, 134.32, 134.95, 135.27, 156.01; HRMS: m/z [M + H]+ calcd for C30H33N4O2 481.2603, found: 481.2604; IR (KBr, cm−1): 3254, 2958, 2898, 2870, 1709, 1632, 1511, 1462, 1259, 1215, 1177, 1078, 1051, 832, 744.
1-(2-(4-((2,4-Dibromo-6-nitrophenoxy)methyl)-1H-1,2,3-triazol-1-yl)ethyl)-1,8-diethyl-1,3,4,9-tetrahydropyrano[3,4-b]indole (6c)
Brown colour solid; yield 87%; mp 141 °C; chemical formula: C26H27Br2N5O4; 1H NMR (400 MHz, CDCl3): δ 0.95 (t, J = 7.09, 3H), 1.3 (t, J = 7.3, 3H), 1.75–1.9 (m, 1H), 1.95–2.15 (m, 1H), 2.45–2.68 (m, 2H), 2.75–2.85 (m, 3H), 2.87–3.02 (m, 1H), 3.95–4.05 (m, 1H), 3.95–4.15 (m, 2H), 4.25–4.35 (m, 1H), 4.44–4.55 (m, 1H), 4.85 (d, J = 12.22 Hz, 1H), 5.15 (d, J = 11.98 Hz, 1H), 7.08–7.15 (m, 2H), 7.25–7.4 (m, 2H), 7.75–7.95 (m, 2H); 13C NMR (100 MHz, CDCl3): δ 7.88, 13.25, 21.96, 23.87, 31.55, 38.11, 46.25, 60.48, 67.72, 75.40, 108.99, 115.90, 117.26, 119.88, 120.52, 121.17, 124.80, 126.22, 126.60, 127.29, 134.98, 135.25, 140.21, 141.98, 145.61, 148.22; HRMS: m/z [M + H]+ calcd for C26H28Br2N5O4 632.0508, found: 632.0508; IR (KBr, cm−1): 3249, 3073, 2964, 2920, 1692, 1528, 1445, 1352, 1254, 1139, 1045, 969, 870, 810, 744, 728.
1,8-Diethyl-1-(2-(4-((naphthalen-1-yloxy)methyl)-1H-1,2,3-triazol-1-yl)ethyl)-1,3,4,9-tetrahydropyrano[3,4-b]indole (6d)
Pale yellow solid; yield 92%; mp 161 °C; chemical formula: C30H32N4O2; 1H NMR (400 MHz, CDCl3): δ 0.96 (t, J = 7.09, 3H), 1.3 (t, J = 7.3, 3H), 1.80–1.90 (m, 1H), 1.91–2.15 (m, 1H), 2.45–2.58 (m, 1H), 2.59–2.68 (m, 1H), 2.71–2.86 (m, 3H), 2.87–3.01 (m, 1H), 3.9–4.15 (m, 2H), 4.25–4.35 (m, 1H), 4.45–4.6 (m, 1H), 4.85 (d, J = 12.22 Hz, 1H), 5.2 (d, J = 11.98 Hz, 1H), 6.84–6.95 (m, 1H), 7.15–7.20 (m, 2H), 7.3–7.6 (m, 6H), 7.82 (m, 1H), 8.2 (s, 2H); 13C NMR (100 MHz, CDCl3): δ 7.8, 13.62, 21.90, 23.85, 31.49, 37.99, 46.12, 60.40, 61.85, 70.02, 105.13, 108.87, 115.86, 119.89, 120.49, 120.68, 121.95, 123.47, 125.15, 125.54, 125.72, 126.16, 126.38, 126.57, 127.40, 134.45, 134.95, 135.24, 153.84; HRMS: m/z [M + H]+ calcd for C30H33N4O2 481.2603, found: 481.2600; IR (KBr, cm−1): 3276, 2953, 2898, 2870, 1741, 1681, 1577, 1456, 1259, 1095, 788, 771.
1-(2-(4-((4-Bromophenoxy)methyl)-1H-1,2,3-triazol-1-yl)ethyl)-1,8-diethyl-1,3,4,9-tetrahydropyrano[3,4-b]indole (6e)
Off white solid; yield 89%; mp 132 °C; chemical formula: C26H29BrN4O2; 1H NMR (400 MHz, CDCl3): δ 0.95 (t, J = 7.09, 3H), 1.3 (t, J = 7.3, 3H), 1.74–1.89 (m, 1H), 1.91–2.15 (m, 1H), 2.40–2.15 (m, 1H), 2.52–2.67 (m, 1H), 2.70–2.85 (m, 3H), 2.85–3.01 (m, 1H), 3.95–4.15 (m, 2H), 4.3–4.45 (m, 2H), 4.55 (d, J = 12.22 Hz, 1H), 4.85 (d, J = 11.98 Hz, 1H), 6.78 (m, 2H), 7.0–7.2 (m, 1H), 7.25–7.45 (m, 5H), 8.3 (s, 1H); 13C NMR (100 MHz, CDCl3): δ 7.89, 13.19, 20.92, 23.53, 31.42, 37.55, 46.26, 61.36, 62.67, 75.34, 108.95, 113.63, 116.65, 117.82, 120.02, 121.93, 123.20, 125.63, 126.67, 130.05, 133.13, 135.27, 135.83, 157.62; HRMS: m/z [M + H]+ calcd for C26H30BrN4O2 509.1552, found: 509.1553; IR (KBr, cm−1): 3276, 2953, 2931, 2892, 1709, 1593, 1489, 1456, 1237, 1193, 963, 826, 782, 689, 585 cm−1.
1,8-Diethyl-1-(2-(4-(phenoxymethyl)-1H-1,2,3-triazol-1-yl)ethyl)-1,3,4,9-tetrahydropyrano[3,4-b]indole (6f)
Pale yellow solid. Yield 93%; mp 84 °C; chemical formula: C26H30N4O2, 1H NMR (400 MHz, CDCl3): δ 0.93 (t, J = 7.3, 3H), 1.36 (t, J = 7.3, 3H), 1.83–1.95 (m, 1H), 1.99–2.13 (m, 1H), 2.38–2.94 (m, 1H), 2.61–2.68 (m, 1H), 2.72–2.91 (m, 3H), 2.89–2.99 (m, 1H), 3.98–4.05 (m, 1H), 4.06–4.14 (m, 1H), 4.15–4.26 (m, 1H), 4.35–4.42 (m, 1H) 4.61 (d, J = 12.22 Hz, 1H), 4.93 (d, J = 11.98 Hz, 1H), 6.85–7.05 (m, 2H), 7.12–7.34 (m, 2H), 7.32–7.41 (m, 5H), 8.52 (s, 1H); 13C NMR (100 MHz, CDCl3): δ 7.83, 13.70, 22.50, 23.88, 29.73, 31.42, 37.87, 46.17, 60.47, 61.29, 75.41, 108.61, 114.70, 115.82, 119.81, 120.41, 121.60, 123.72, 126.18, 129.82, 135.06, 135.49, 143.62, 150.08, 158.16; HRMS: m/z [M + H]+ calcd for C26H31N4O2 431.2447, found: 431.2446; IR (KBr, cm−1): 3248, 2953, 2925, 1711, 1586, 1493, 1460, 1186, 968, 754, 694.
1,8-Diethyl-1-(2-(4-((m-tolyloxy)methyl)-1H-1,2,3-triazol-1-yl)ethyl)-1,3,4,9-tetrahydropyrano[3,4-b]indole (6g)
Yellow solid; yield 92%; mp 129 °C; chemical formula: C27H32N4O2: 1H NMR (400 MHz, CDCl3): δ 0.96 (t, J = 7.08, 3H), 1.36 (t, J = 7.32, 3H), 1.81–1.90 (m, 1H), 1.97–2.07 (m, 1H), 2.18 (s, 3H), 2.46–2.52 (m, 1H), 2.62–2.69 (m, 1H), 2.75–2.82 (m, 3H), 2.89–2.96 (m, 1H), 3.98–4.04 (m, 1H), 4.09–4.12 (m, 1H), 4.22–4.29 (m, 1H), 4.39–4.46 (m, 1H), 4.79 (d, J = 12.22 Hz, 1H), 5.01 (d, J = 12.22 Hz, 1H), 6.80 (d, J = 8.07 Hz, 1H), 6.86–6.90 (m, 1H), 7.01–7.35 (m, 4H), 7.36–7.45 (m, 2H), 8.56 (s, 1H); 13C NMR (100 MHz, CDCl3): δ 7.73, 13.61, 16.14, 21.85, 23.82, 31.33, 37.93, 46.13, 60.38, 61.68, 75.40, 108.64, 111.25, 115.72, 119.74, 120.36, 120.76, 123.27, 126.12, 126.64, 126.69, 126.82, 130.65, 134.94, 135.31, 144.05, 156.24; HRMS: m/z [M + H]+ calcd for C27H33N4O2 445.2603, found: 445.2603; IR (KBr, cm−1): 3215, 2958, 2920, 2893, 2871, 1739, 1689, 1591, 1498, 1460, 1378, 1241, 1050, 858, 793, 742.
1,8-Diethyl-1-(2-(4-((o-tolyloxy)methyl)-1H-1,2,3-triazol-1-yl)ethyl)-1,3,4,9-tetrahydropyrano[3,4-b]indole (6h)
Yellow solid; yield 93%; mp 130 °C; chemical formula: C27H32N4O2: 1H NMR (400 MHz, CDCl3): δ 0.95 (t, J = 7.08, 3H), 1.29 (t, J = 7.3, 3H), 1.79–1.88 (m, 1H), 1.95–2.03 (m, 1H), 2.18 (s, 3H), 2.44–2.52 (m, 1H), 2.60–2.68 (m, 1H), 2.74–2.82 (m, 3H), 2.88–2.96 (m, 1H), 3.97–4.03 (m, 1H), 4.08–4.12 (m, 1H), 4.21–4.28 (m, 1H), 4.38–4.45 (m, 1H), 4.75 (d, J = 12.22 Hz, 1H), 4.98 (d, J = 12.22 Hz, 1H), 6.81 (d, J = 7.82 Hz, 1H), 6.86–6.90 (m, 1H), 7.01–7.09 (m, 2H), 7.13 (d, J = 7.33 Hz, 2H), 7.26–7.37 (m, 2H), 8.42 (s, 1H); 13C NMR (125 MHz, CDCl3): δ 7.76, 13.60, 16.16, 21.87, 23.83, 31.39, 37.93, 46.12, 60.39, 61.70, 75.39, 108.71, 111.29, 115.76, 119.79, 120.40, 120.77, 123.28, 126.13, 126.62, 126.71, 126.84, 130.66, 134.95, 135.29, 144.09, 156.26; HRMS: m/z [M + H]+ calcd for C27H33N4O2 445.2603, found: 445.2602; IR (KBr, cm−1): 3248, 2964, 2925, 2871, 1744, 1684, 1591, 1493, 1454, 1372, 1236, 1055, 858, 787, 738.
1,8-Diethyl-1-(2-(4-((p-tolyloxy)methyl)-1H-1,2,3-triazol-1-yl)ethyl)-1,3,4,9-tetrahydropyrano[3,4-b]indole (6i)
Pale yellow solid; yield 90%; mp 140 °C; chemical formula: C27H32N4O2; 1H NMR (400 MHz, CDCl3): δ 0.95 (t, J = 7.08, 3H), 1.35 (t, J = 7.3, 3H), 1.77–1.86 (m, 1H), 1.94–2.01 (m, 1H), 2.28 (s, 3H), 2.42–2.48 (m, 1H), 2.56–2.64 (m, 1H), 2.74–2.80 (m, 3H), 2.88–2.95 (m, 1H), 3.96–4.02 (m, 1H), 4.07–4.13 (m, 1H), 4.16–4.27 (m, 1H), 4.36–4.45 (m, 1H), 4.72 (d, J = 12.22 Hz, 1H), 4.91 (d, J = 12.22 Hz, 1H), 6.78 (d, J = 8.3 Hz, 2H), 7.01–7.10 (m, 3H), 7.26–7.33 (m, 2H), 7.35 (d, J = 7.82 Hz, 1H), 8.35 (s, 1H); 13C NMR (100 MHz, CDCl3): δ 7.75, 13.60, 20.39, 22.39, 23.80, 31.37, 37.89, 46.07, 60.37, 61.45, 75.34, 108.68, 114.48 (2C), 115.75, 119.77, 120.38, 126.64, 129.71, 129.83 (2C), 130.31, 134.94, 135.30, 143.75, 155.96; HRMS: m/z [M + H]+ calcd for C27H33N4O2 445.2603, found: 445.2605; IR (KBr, cm−1): 3254, 2953, 2909, 1722, 1596, 1503, 1312, 1225, 1055, 951, 825, 787, 749, 650, 508.
1,8-Diethyl-1-(2-(4-((4-iodophenoxy)methyl)-1H-1,2,3-triazol-1-yl)ethyl)-1,3,4,9-tetrahydropyrano[3,4-b]indole (6j)
Yellow solid; yield 94%; mp 119 °C; chemical formula: C26H29IN4O2; 1H NMR (400 MHz, CDCl3): δ 0.95 (t, J = 7.09 Hz, 3H), 1.30 (t, J = 7.2 Hz, 3H), 1.76–1.85 (m, 1H), 1.93–2.01 (m, 1H), 2.43–2.50 (m, 1H), 2.55–2.63 (m, 1H), 2.72–2.79 (m, 3H), 2.87–2.94 (m, 1H), 3.94–4.00 (m, 1H), 4.07–4.11 (m, 1H), 4.20–4.27 (m, 1H), 4.37–4.44 (m, 1H), 4.64 (d, J = 12.22 Hz, 1H), 4.95 (d, J = 12.22 Hz, 1H), 6.64 (d, J = 8.8 Hz, 2H), 7.01 (d, J = 7.0 Hz, 1H), 7.07 (t, J = 7.5 Hz, 1H), 7.1 (s, 1H), 7.35 (d, J = 7.5 Hz, 1H), 7.5 (d, J = 8.80 Hz, 2H), 8.03 (s, 1H); 13C NMR (100 MHz, CDCl3): δ 7.78, 13.57, 21.89, 23.78, 31.49, 37.91, 46.03, 60.37, 61.37, 75.26, 108.83, 115.84, 117.07, 119.88, 120.47, 123.59, 126.09, 126.52, 134.90, 135.19, 138.17, 143.09, 157.94; HRMS: m/z [M + H]+ calcd for C26H30IN4O2 547.1413, found: 547.1415; IR (KBr, cm−1): 3271, 2958, 2926, 2893, 1736, 1687, 1583, 1523, 1484, 1369, 1249, 1227, 1106, 1046, 805, 745, 635, 580.
4-((1-(2-(1,8-Diethyl-1,3,4,9-tetrahydropyrano[3,4-b]indol-1-yl)ethyl)-1H-1,2,3-triazol-4-yl)methoxy)-3-methoxybenzaldehyde (6k)
Pale yellow solid. Yield 92%; mp 193 °C; chemical formula: C28H32N4O4; 1H NMR (400 MHz, CDCl3): δ 0.96 (t, J = 7.07 Hz, 3H), 1.32 (t, J = 7.3 Hz, 3H), 1.77–1.82 (m, 1H), 1.92–1.99 (m, 1H), 2.44–2.58 (m, 2H), 2.75–2.80 (m, 3H), 2.86–2.96 (m, 1H), 3.88 (s, 3H), 3.42–4.05 (m, 1H), 4.06–4.18 (m, 1H), 4.21–4.33 (m, 1H), 4.37–4.48 (m, 1H), 4.75 (d, J = 12.42 Hz, 1H), 5.08 (d, J = 12.71 Hz, 1H), 7.01–7.12 (m, 3H), 7.20 (s, 1H), 7.34–7.47 (m, 3H), 7.55 (brs, 1H), 9.83 (s, 1H); 13C NMR (100 MHz, CDCl3): δ 7.83, 13.54, 22.39, 23.79, 31.66, 37.93, 46.00, 55.95, 60.36, 62.26, 75.17, 109.09, 109.13, 112.32, 115.99, 120.05, 120.60, 124.01, 126.12, 126.37, 126.68, 130.42, 134.90, 135.09, 142.69, 149.82, 153.01, 190.88; HRMS: m/z [M + H]+ calcd for C28H33N4O4 489.2501, found: 489.2502; IR (KBr, cm−1): 3271, 2958, 2909, 2904, 2854, 1687, 1583, 1512, 1463, 1265, 1172, 1128, 1013, 854, 810, 750.
4-((1-(2-(1,8-Diethyl-1,3,4,9-tetrahydropyrano[3,4-b]indol-1-yl)ethyl)-1H-1,2,3-triazol-4-yl)methoxy)benzaldehyde (6l)
Brown solid; yield 94%; mp 123 °C; chemical formula: C27H30N4O3: 1H NMR (400 MHz, CDCl3): δ 0.95 (t, J = 7.06 Hz, 3H), 1.30 (t, J = 7.2 Hz, 3H), 1.78–1.90 (m, 1H), 1.95–2.09 (m, 1H), 2.44–2.55 (m, 1H), 2.56–2.67 (m, 1H), 2.70–2.85 (m, 3H), 2.86–3.01 (m, 1H), 3.92–4.04 (m, 1H), 4.05–4.19 (m, 1H), 4.20–4.34 (m, 1H), 4.37–4.49 (m, 1H), 4.70 (d, J = 12.22 Hz, 1H), 4.95 (d, J = 12.22 Hz, 1H), 6.95–7.09 (m, 4H), 7.16 (s, 1H), 7.36 (d, J = 7.33 Hz, 1H), 7.80 (d, J = 8.80 Hz, 2H), 8.24 (brs, 1H), 9.86 (s, 1H); 13C NMR (100 MHz, CDCl3): δ 7.78, 13.58, 22.39, 23.78, 31.44, 37.85, 46.06, 60.35, 61.47, 75.21, 108.80, 114.92 (2C), 115.86, 119.87, 120.47, 123.77, 126.09, 126.50, 130.12, 131.92 (2C), 134.90, 135.25, 142.50, 163.07, 190.82; HRMS: m/z [M + H]+ calcd for C27H31N4O3 459.2396, found: 459.2395; IR (KBr, cm−1): 3183, 2953, 2926, 2854, 1698, 1600, 1512, 1463, 1315, 1260, 1167, 1073, 1013, 865, 827, 750.
Acknowledgements
Bhaskar Kummari thankful to CSIR for awarding Senior Research Fellowship. Thankful to TEQIP II for laboratory development and PS3 LABORATORIES LLP for HPLC analysis.
References
- G. Wei, W. Luan, S. Wang, S. Cui, F. Li, Y. Liu, Y. Liu and M. Cheng, Org. Biomol. Chem., 2015, 13, 1507–1514 CAS.
-
(a) American Cancer Society, Cancer Facts & Figures 2015, American Cancer Society, Atlanta, 2015 Search PubMed;
(b) American Cancer Society, Cancer Facts & Figures 2014, American Cancer Society, Atlanta, 2014 Search PubMed;
(c) American Cancer Society, Cancer Facts & Figures 2013, American Cancer Society, Atlanta, 2013 Search PubMed;
(d) American Cancer Society, Cancer Facts & Figures 2012, American Cancer Society, Atlanta, 2012 Search PubMed;
(e) American Cancer Society, Cancer Facts & Figures 2012, American Cancer Society, Atlanta, 2011 Search PubMed;
(f) American Cancer Society, Cancer Facts & Figures 2012, American Cancer Society, Atlanta, 2010 Search PubMed;
(g) P. Anand, A. B. Kunnumakkara, C. Sundaram, K. B. Harikumar, S. T. Tharakan, O. S Lai, B. Sung and B. B. Aggarwal, Pharm. Res., 2008, 9, 2097–2116 CrossRef PubMed;
(h) Q. P. Peterson, D. C. Hsu, D. R. Goode, C. J. Novotny, R. K. Totten and P. J. Hergenrother, J. Med. Chem., 2009, 52, 5721–5731 CrossRef CAS PubMed.
- A. Kamal, S. Prabhakar, M. J. Ramaiah, P. V. Reddy, C. H. R. Reddy, A. Mallareddy, N. Shankaraiah, T. L. N. Reddy, S. N. C. V. L. Pushpavalli and M. Pal-Bhadra, Eur. J. Med. Chem., 2011, 46, 3820–3831 CrossRef CAS PubMed.
-
(a) Y. C. Duan, Y. C. Ma, E. Zhang, X. J. Shi, M. M. Wang, X. W. Ye and H. M. Liu, Eur. J. Med. Chem., 2013, 62, 11–19 CrossRef CAS PubMed;
(b) Y. C. Duan, Y. C. Zheng, X. C. Li, M. M. Wang, X. W. Ye, Y. Y. Guan, G. Z. Liu, J. X. Zheng and H. M. Liu, Eur. J. Med. Chem., 2013, 64, 99–110 CrossRef CAS PubMed;
(c) Y. C. Zheng, Y. C. Duan, J. L. Ma, R. M. Xu, X. L. Zi, W. L. Lv, M. M. Wang, X. W. Ye, S. Zhu, D. Mobley, Y. Y. Zhu, J. W. Wang, J. F. Li, Z. R. Wang, W. Zhao and H. M. Liu, J. Med. Chem., 2013, 56, 8543–8560 CrossRef CAS PubMed.
-
(a) S. Kundooru, P. Das, S. Meena, V. Kumar, M. I. Siddiqi, D. Datta and A. K. Shaw, Org. Biomol. Chem., 2015, 13, 8241–8250 RSC;
(b) N. Pokhodylo, O. Shyyka and V. Matiychuk, Sci. Pharm., 2013, 81, 663–676 CrossRef CAS PubMed;
(c) L. V. R. Reddy, P. V. Reddy, N. N. Mishra, P. K. Shukla, G. Yadav, R. Srivastava and A. K. Shaw, Carbohydr. Res., 2010, 345, 1515–1521 CrossRef CAS PubMed , references cited therein;
(d) P. Jangili, J. Kashanna, C. G. Kumar, Y. Poornachandra and B. Das, Bioorg. Med. Chem. Lett., 2014, 24, 325–327 CrossRef CAS PubMed;
(e) S. R. Mandha, S. Siliveri, M. Alla, V. R. Bommena, M. R. Bommineni and S. Balasubramanian, Bioorg. Med. Chem. Lett., 2012, 22, 5272–5278 CrossRef CAS PubMed;
(f) P. Jangili, C. G. Kumar, Y. Poornachandra and B. Das, Synthesis, 2015, 47, 653–658 CAS.
- J.-M. Xu, E. Zhang, X.-J. Shi, Y.-C. Wang, B. Yu, W.-W. Jiao, Y.-Z. Guo and H. M. Liu, Eur. J. Med. Chem., 2014, 80, 593–604 CrossRef CAS PubMed.
-
(a) R. Liu, K.-P. Xu and G.-S. Tan, Eur. J. Pharmacol., 2015, 769, 127–133 CrossRef CAS PubMed;
(b) W. Fei, W. Chen, L. Shengnan, W. Huihui, X. Shuhua and S. Guifan, Toxicol. Res., 2015, 4, 1400 RSC;
(c) W. Dempke, C. Rie, A. Grothey and H. J. Schmoll, J. Cancer Res. Clin. Oncol., 2001, 127, 411–417 CrossRef CAS PubMed;
(d) T. Kamijo, T. Sato, Y. Nagatomi and T. Kitamura, Int. J. Urol., 2001, 8, 35–39 CrossRef;
(e) M. Kobayashi, S. Nakamura, K. Shibata, N. Sahara, K. Shigeno, K. Shinjo, K. Naito and K. Ohnishi, Eur. J. Haematol, 2005, 75, 212–220 CrossRef CAS PubMed;
(f) B. W. Stewart and C. P. Wild, World Cancer Report, 2014, World Health Organization, IARC Nonserial Publication, 2014 and references cited therein Search PubMed.
- A. Alexakis, A. Tomassini and S. Leconte, Tetrahedron, 2004, 60, 9479–9484 CrossRef CAS.
- L. Rokhum and G. Bez, J. Chem. Sci., 2012, 124, 687–691 CrossRef CAS.
- B.-Y. Lee, S. R. Park, H. B. Jeon and K. S. Kim, Tetrahedron Lett., 2006, 47, 5105–5109 CrossRef CAS.
- J.-M. Schumers, J.-F. Gohy and C.-A. Fustin, Macromolecules, 2000, 33, 8629–8639 CrossRef.
-
(a) R. Huisgen, R. Grashey and J. Sauer, Chemistry of Alkenes, Interscience, New York, 1964, pp. 806–877 Search PubMed;
(b) V. V. Rostovtsev, L. G. Green, V. V. Fokin and K. B. Sharpless, Angew. Chem., Int. Ed., 2002, 41, 2596–2599 CrossRef CAS;
(c) F. Himo, T. Lovell, R. Hilgraf, V. V. Rostovtsev, L. Noodleman, K. B. Sharpless and V. V. Fokin, J. Am. Chem. Soc., 2005, 127, 210–216 CrossRef CAS PubMed.
-
(a) S. S. Chauhan, A. K. Singh, S. Meena, M. Lohani, A. Singh, R. K. Arya, S. H. Cheruvu, J. Sarkar, J. R. Gayen, D. Datta and P. M. Chauhan, Bioorg. Med. Chem. Lett., 2014, 13, 2820–2824 CrossRef PubMed;
(b) M. F. Abu Bakar, M. Mohamad, A. Rahmat, S. A. Burr and J. R. Fry, Food Chem. Toxicol., 2010, 48, 1688–1697 CrossRef CAS PubMed.
-
(a) C. Yakaiah, T. Sneha, T. Shalini, C. Srinivas, K. Anand, K. A. Niranjana, K. V. N. S. Srinivas, A. Sarfaraz, K. J. Kotesh, K. Feroz, T. Ashok and G. Paramjit, Eur. J. Med. Chem., 2015, 93, 564–573 CrossRef PubMed;
(b) F. ChengTao, W. D. Ling, Y. YuGang, L. Jian and L. ShaoHua, Med. Chem. Res., 2012, 21, 315–320 CrossRef;
(c) S. Shubhanjali, S. S. Radhey, S. Sushant Kumar, S. Ajit and K. Pankaj, J. Enzyme Inhib. Med. Chem., 2013, 28, 1192–1198 CrossRef PubMed.
- Schrodinger, Glide, Version 6.0, LLC, New York, NY, 2013 Search PubMed.
Footnote |
† Electronic supplementary information (ESI) available. See DOI: 10.1039/c6ra28525b |
|
This journal is © The Royal Society of Chemistry 2017 |
Click here to see how this site uses Cookies. View our privacy policy here.