DOI:
10.1039/C7RA00799J
(Paper)
RSC Adv., 2017,
7, 13072-13081
Synthesis, optical and electrochemical properties, and photovoltaic performance of a panchromatic and near-infrared (D)2–π–A type BODIPY dye with pyridyl group or cyanoacrylic acid†
Received
19th January 2017
, Accepted 21st February 2017
First published on 24th February 2017
Abstract
(D)2–π–A type boron dipyrromethene (BODIPY) dyes OMK-PY and OMK-CA bearing a pyridyl group or cyanoacrylic acid group, respectively, at the end of 8-positions on the BODIPY core, as an electron-withdrawing anchoring group to adsorb onto the TiO2 electrode and two diphenylamine–thienylcarbazole moieties as an electron-donating unit at the 3- and 5-positions on the BODIPY core, were designed and developed as a photosensitizer for dye-sensitized solar cells (DSSCs). It was found that the two BODIPY dyes adsorbed on a TiO2 film show a strong and broad absorption band in the range of 600 to 850 nm, and the onset of the absorption band reached 900 nm, that is, OMK-PY and OMK-CA possess the near-infrared (NIR) adsorption ability as well as the panchromatic adsorption ability, and good adsorption ability onto the TiO2 electrode. Moreover, cyclic voltammetry demonstrated that the two BODIPY dyes show two reversible oxidation waves, thus indicating that the redox processes of OMK-PY and OMK-CA are very stable. On the basis of the experimental results and density functional theory calculation, we propose that the (D)2–π–A BODIPY structure with two diphenylamine–thienylcarbazole moieties as strong electron-donating units at the 3- and 5-positions on the BODIPY core is an effective strategy to lead a high light-harvesting efficiency (LHE) in the range of visible light to NIR light, although the DSSCs based on (D)2–π–A type BODIPY dyes that have been developed in this current stage showed low photovoltaic performances.
Introduction
Dye-sensitized solar cells (DSSCs) employing dye-adsorbed TiO2 electrodes have made a hopeful impact on chemists, physicists, and engineers as one of the most promising new renewable photovoltaic cells in the last quarter of a century,1–8 since Grätzel and co-workers reported high-performance DSSCs employing a Ru-complex dye-adsorbed TiO2 electrode in 1991.9 In particular, much effort has been made on the development of various types of D–π–A organic dye sensitizers which are constructed of a diphenyl- or dialkylamino group as the electron donor (D) and a carboxyl group,10–16 2-(1,1-dicyanomethylene)rhodanine,17 pyridine,18–21 or 8-hydroxylquinoline22 as the electron acceptor (A) and anchor linked by π-conjugated bridges, and there has been a gradual accumulation of information about the relationship between the chemical structures and the photovoltaic performances of DSSCs. As a result, DSSCs have achieved a solar energy-to-electricity conversion yield (η) of up to 13%.11c,d However, one of the challenges to further improve the photovoltaic performances of DSSCs lies in the enhancement of light-harvesting efficiency (LHE) in the near-infrared (NIR) region (780–1000 nm) as well as visible region of the solar spectrum. Some boron dipyrromethene (BODIPY) dyes which are electrochemically modified through the introduction of electron-donating and electron-accepting groups onto the BODIPY core, possess panchromatic absorption ability because of their high photoabsorption coefficient in the visible region of the solar spectrum.23–25 Actually, Akkaya et al. have designed and developed a series of D–A-substituted BODIPY dye sensitizers, which have two triphenylamines as electron donors at the 3- and 5-positions and benzoic acid as electron acceptor at the 8-position. The D–A-substituted BODIPY dyes showed a strong and broad absorption band in the range of 650 to 850 nm with the absorption maxima at around 720–760 nm (molar extinction coefficient (ε) = 60
000–70
000 M−1 cm−1).23d However, almost all BODIPY photosensitizers reported so far show the photoabsorption band in wavelength region shorter than 700 nm.
Thus, in this work, to explore effective BODIPY dye sensitizers possessing a high LHE in the range of visible light to NIR light, we have designed and developed (D)2–π–A type BODIPY dyes OMK-PY and OMK-CA bearing pyridyl group and cyanoacrylic acid group, respectively, at the end of 8-positions on the BODIPY core, as an electron-withdrawing anchoring group to adsorb onto the TiO2 electrode and two diphenylamine–thienylcarbazole moieties as an electron-donating unit at the 3- and 5-positions on the BODIPY core (Fig. 1). Here we reveal the photophysical and electrochemical properties of the (D)2–π–A BODIPY dye sensitizers and their photovoltaic performances in DSSCs, based on photoabsorption (dye solution and dye-adsorbed TiO2 film) and electrochemical measurements (cyclic voltammetry), density functional theory (DFT) calculation, FT-IR spectroscopy of the dye-adsorbed TiO2 film, photocurrent–voltage (I–V) curves, and incident photon-to-current conversion efficiency (IPCE) spectra. We demonstrate that the (D)2–π–A type BODIPY dyes possess the NIR adsorption ability as well as the panchromatic adsorption ability.
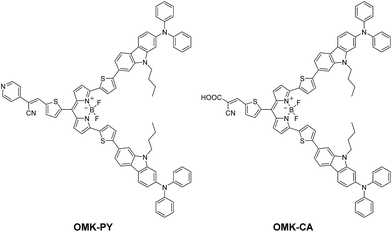 |
| Fig. 1 Chemical structures of (D)2–π–A type BODIPY dyes OMK-PY and OMK-CA. | |
Results and discussion
Synthesis
(D)2–π–A BODIPY dye sensitizers OMK-PY and OMK-CA studied in this work were synthesized according to a stepwise synthetic protocol (Scheme 1). Indeed, the (D)2–π–A BODIPY dyes were successfully prepared by using Stille coupling to introduce the electron-donating unit (two diphenylamine–thienylcarbazole moieties), and then by using Knoevenagel condensation to introduce the electron-withdrawing anchoring group (pyridyl group or cyanoacrylic acid group). Dipyrromethane 2 was prepared by condensation of compound 1 (ref. 26) with pyrrole with TFA added as a catalyst. The dichlorinated compound 3 was prepared by chlorination of 2 with N-chlorosuccinimide (NCS) followed by oxidation with DDQ. Compound 3 was reacted with NEt3 and treated with BF3–OEt2 to afford the BODIPY 4. Compound 6 was prepared by Stille coupling of 4 with stannanyl compound 5 (ref. 18g). Compound 6 is hydrolyzed by treatment with acid to generate the aldehyde 7. BODIPY dye OMK-PY was prepared by reacting 4-pyridylacetonitrile hydrochloride with the compound 7 in the presence of NEt3.27 BODIPY dye OMK-CA was prepared by typical Knoevenagel condensation of the compound 7 with cyanoacetic acid in the presence of piperidine.
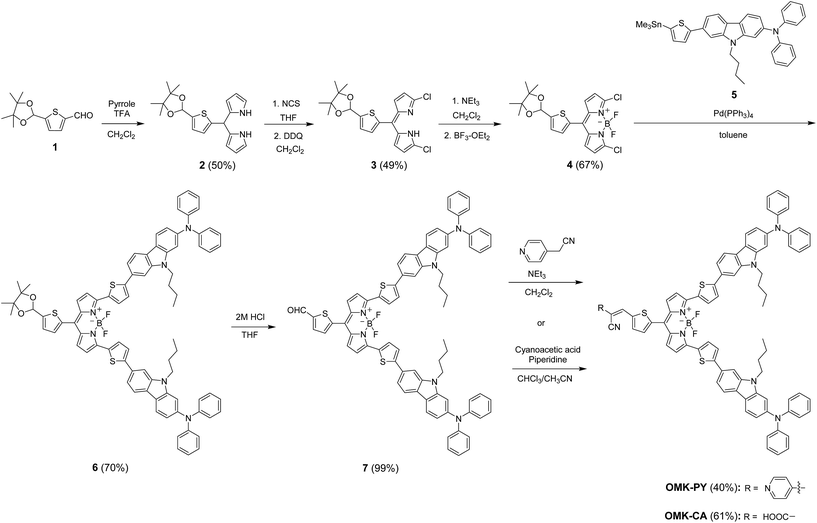 |
| Scheme 1 Synthesis of OMK-PY and OMK-CA. | |
Photophysical properties
The photoabsorption spectra of OMK-PY and OMK-CA in THF and adsorbed on the TiO2 film are shown in Fig. 2a and their optical data are summarized in Table 1. The two dyes in THF show a strong and broad absorption band in the range of 600 to 800 nm, which is assigned to the intramolecular charge-transfer (ICT) excitation from the electron donor moiety (two diphenylamine–thienylcarbazole moieties) through the BODIPY core to the electron acceptor moiety (2-(pyridin-4-yl)acrylonitrile moiety for OMK-PY and cyanoacetic acid group for OMK-CA, respectively). For OMK-PY the molar extinction coefficient (ε) at the absorption peak wavelength (λabs = 753 nm) is 95
400 M−1 cm−1, which is higher than that of OMK-CA (ε = 83
100 M−1 cm−1 at λabs = 754 nm). Moreover, for the two dyes a strong absorption peak at around 400 nm with the ε value of ca. 70
000–80
000 M−1 cm−1, which can be assigned to the ICT band due to the diphenylamine–thienylcarbazole moiety, was also observed. Interestingly, for the absorption spectra of OMK-PY and OMK-CA adsorbed on TiO2 film the longest wavelength absorption band in the range of 600 to 850 nm is significantly broadened, and the onset of the absorption band reached 900 nm, compared with that in THF. As shown in Fig. 2b, the colors of the two dyes in THF are blackish green and the color of dye-adsorbed TiO2 film is almost black. Thus, this result indicates that the (D)2–π–A type BODIPY dyes possess a good LHE in the range of visible light to NIR light and good adsorption ability onto TiO2 electrode, that is, OMK-PY and OMK-CA possess the NIR adsorption ability as well as the panchromatic adsorption ability.
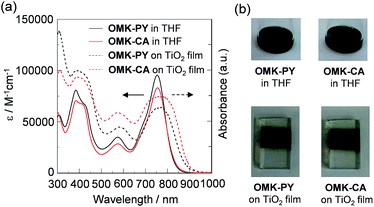 |
| Fig. 2 (a) Photoabsorption spectra of OMK-PY and OMK-CA in THF and adsorbed on TiO2 film. (b) Photographs of the dyes in THF and adsorbed on TiO2 film. | |
Table 1 Optical and electrochemical data, HOMO and LUMO energy levels, and DSSC performance parameters of OMK-PY and OMK-CA
Dye |
λabsmax/nm (εa/M−1 cm−1) |
Eox1/2b/V |
HOMOc/V |
LUMOc/V |
Moleculesd/cm−2 |
Jsce/mA cm−2 |
Voce/mV |
ffe |
ηe (%) |
In THF. Half-wave potentials versus Fc/Fc+ of oxidation (Eox1/2) for OMK-PY and OMK-CA were recorded in DMF/Bu4NClO4 (0.1 M) solution. Versus normal hydrogen electrode (NHE). Adsorption amount of dye molecules per unit area of the TiO2 electrode, when the 9 μm thick TiO2 electrode was immersed into 0.1 mM dye solution in THF. Under a simulated solar light (AM 1.5, 100 mW cm−2). |
OMK-PY |
385 (80 800), 574 (35 000), 753 (95 400) |
0.28 |
1.00 |
−0.44 |
6.9 × 1016 |
1.08 |
335 |
0.53 |
0.19 |
OMK-CA |
385 (70 600), 574 (28 500), 754 (83 100) |
0.29 |
1.01 |
−0.43 |
1.0 × 1017 |
0.68 |
319 |
0.51 |
0.11 |
Electrochemical properties
The electrochemical properties of OMK-PY and OMK-CA were determined by cyclic voltammetry (CV) in DMF containing 0.1 M tetrabutylammonium perchlorate (Bu4NClO4) (Fig. 3). The potentials were referenced to ferrocene/ferrocenium (Fc/Fc+) as the internal reference. Two oxidation waves were observed at 0.34 V and 0.48 V for OMK-PY and 0.33 V and 0.48 V for OMK-CA, corresponding to the oxidations of the BODIPY core and the diphenylamino group, respectively. The corresponding reduction wave to the first oxidation wave appeared at 0.22 V for OMK-PY and 0.25 V for OMK-CA, respectively, whereas for the two dyes the corresponding reduction wave (ca. 0.42 V) to the second oxidation wave was not clearly observed. These results show that for the two dyes the first redox process is electrochemically reversible, but the second redox process is electrochemically irreversible. The HOMO energy levels of the two dyes were evaluated from the half-wave potential for oxidation (Eox1/2 = 0.28 V for OMK-PY and 0.29 V for OMK-CA, respectively, Table 1). The HOMO energy level was 1.00 V for OMK-PY and 1.01 V for OMK-CA, respectively, versus the normal hydrogen electrode (NHE). Thus, the two dyes have comparable HOMO energy levels. This result shows that the HOMO energy levels of the two dyes are more positive than the I3−/I− redox potential (0.4 V), and thus this ensures an efficient regeneration of the oxidized dyes by electron transfer from the I3−/I− redox couple in the electrolyte. The LUMO energy level was estimated from the Eox1/2 and the onset of photoabsorption spectra (860 nm; 1.44 eV for both OMK-PY and OMK-CA) in THF. The LUMO energy level was −0.44 V for OMK-PY and −0.43 V for OMK-CA, respectively, and thus the two dyes have comparable LUMO energy levels. However, the LUMO energy levels of the two dyes are lower than the energy level (Ecb) of the conduction band (CB) of TiO2 electrode (−0.5 V). This suggests that the electron injection from the photoexcited dye to the CB of TiO2 electrode is thermodynamically difficult.
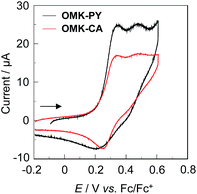 |
| Fig. 3 Cyclic voltammograms of OMK-PY and OMK-CA in DMF containing 0.1 M Bu4NClO4. The arrow denotes the direction of the potential scan. | |
Theoretical calculations
To examine the electronic structures of OMK-PY and OMK-CA, the molecular orbitals of the two dyes were calculated using density functional theory (DFT) at the B3LYP/6-31G(d,p) level.28 As shown in Fig. 4, for the two dyes the HOMO and HOMO−1 are mostly localized on the BODIPY core containing the two diphenylamine–thienylcarbazole moieties and the two diphenylamine–carbazole moieties, respectively. The LUMO is mostly localized on the BODIPY core containing thiophene-2-(pyridin-4-yl)acrylonitrile moiety for OMK-PY and thiophene–cyanoacetic acid group for OMK-CA, respectively, and the LUMO+1 is mostly localized on thiophene-2-(pyridin-4-yl)acrylonitrile moiety for OMK-PY and thiophene–cyanoacetic acid group for OMK-CA, respectively. Accordingly, the DFT calculations reveal that for both OMK-PY and OMK-CA the dye excitations upon light irradiation induce a strong ICT from the electron donor moiety (two diphenylamine–carbazole moieties) through the BODIPY core to the electron acceptor moiety (2-(pyridin-4-yl)acrylonitrile moiety for OMK-PY and cyanoacetic acid group for OMK-CA, respectively).
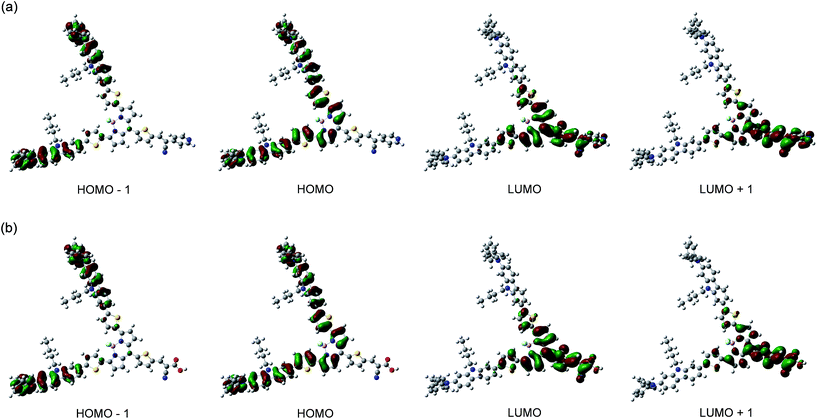 |
| Fig. 4 HOMO−1, HOMO, LUMO and LUMO+1 of (a) OMK-PY and (b) OMK-CA derived from the DFT calculations at B3LYP/6-31G(d,p) level. | |
FT-IR spectra
To elucidate the adsorption states of OMK-PY and OMK-CA on TiO2 nanoparticles, we measured the FTIR spectra of the dye powders and the dyes adsorbed on TiO2 film (Fig. 5). For the dye powder of OMK-PY the C
N stretching band of the pyridyl group was observed at around 1590 cm−1. When OMK-PY was adsorbed on the TiO2 film, the C
N stretching band was slightly shifted by 1 cm−1, to higher wavenumber compared with the dye powders, that is, the band can be assigned to the hydrogen-bonded pyridyl group to Brønsted acid sites on the TiO2 surface.18 For the dye powder of OMK-CA, the C
O stretching band of the carboxyl group was observed at around 1690 cm−1. On the other hand, in the FTIR spectrum of OMK-CA adsorbed onto TiO2 film showed that the C
O stretching bands of the carboxyl group disappeared completely; this indicates the formation of a bidentate bridging linkage between the carboxyl group of the dye and the Brønsted acid site on the TiO2 surface. Consequently, this result demonstrates that OMK-PY and OMK-CA was adsorbed on the TiO2 film through the hydrogen bond and bidentate bridging linkage, respectively, at the Brønsted-acidic sites on the TiO2 surface.
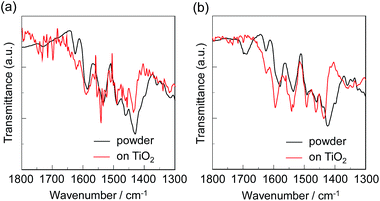 |
| Fig. 5 FTIR spectra of the dye powder and the dye-adsorbed TiO2 film. (a) OMK-PY and (b) OMK-CA. | |
Photovoltaic performances
The DSSCs based on OMK-PY and OMK-CA were fabricated by using the dye-adsorbed TiO2 electrode (9 μm), Pt-coated glass as a counter electrode, and an acetonitrile solution with iodine (0.05 M), lithium iodide (0.1 M), and 1,2-dimethyl-3-propylimidazolium iodide (0.6 M) as the electrolyte. The I–V characteristics were measured under simulated solar light (AM 1.5, 100 mW cm−2). The IPCE spectra and the I–V curves are shown in Fig. 6. The photovoltaic performance parameters (a short circuit photocurrent density (Jsc), an open-circuit photovoltage (Voc), a fill factor (ff) and a solar energy-to-electricity conversion yield (η)) are collected in Table 1. The adsorption amount (1.0 × 1017 molecules per cm2) of OMK-CA adsorbed on TiO2 electrode is larger than that of OMK-PY (6.9 × 1016 molecules per cm2). The high adsorption ability of OMK-CA relative to OMK-PY is attributed to the formation of the bidentate bridging linkage between the carboxyl group of OMK-CA and the Brønsted acid sites on the TiO2 surface. The IPCE spectra showed that the maximum IPCE values of OMK-PY and OMK-CA are 13% and 9%, respectively. It is worth mentioning here that for the two dyes the IPCE value in the range of 600 to 800 nm is notably much lower than that at around 400 nm, that is, the ICT band from the electron donor moiety through the BODIPY core to the electron acceptor moiety make little contribution to the IPCE spectra. The I–V curves show that the Jsc and η values are 1.08 mA cm−2 and 0.19% for OMK-PY and 0.68 mA cm−2 and 0.11% for OMK-CA, respectively. Accordingly, the relatively low photovoltaic performances of OMK-PY and OMK-CA are due to the low LUMO level which makes it thermodynamically difficult for the photoexcited dye to inject the electron into the CB of TiO2 electrode. In addition, the Voc value of BODIPY dye sensitizers is generally lower than that of the conventional organic dye sensitizers.23 It is assumed that the low Voc value (ca. 330 mV) for OMK-PY and OMK-CA is attributed to faster charge recombination between the injected electrons in the CB of TiO2 electrode and I3− ions in the electrolyte, arising from the approach of I3− ions to the TiO2 surface due to the electrostatic interactions between the BODIPY core and I3− ions. Consequently, the low photovoltaic performances of DSSCs based on OMK-PY and OMK-CA are attributed to the low electron-injection efficiency due to the low LUMO level and the faster charge recombination due to the BODIPY core, leading to the low Jsc and Voc values, respectively.
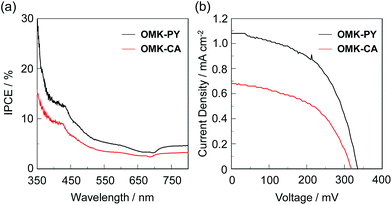 |
| Fig. 6 (a) IPCE spectra and (b) I–V curves of DSSCs based on OMK-PY and OMK-CA. | |
Conclusions
To explore effective BODIPY dye sensitizers possessing a high LHE in the range of visible light to NIR light, we have designed and developed (D)2–π–A type BODIPY dyes OMK-PY and OMK-CA bearing pyridyl group and cyanoacrylic acid group, respectively, at the end of 8-positions on the BODIPY core, as an electron-withdrawing anchoring group to adsorb onto the TiO2 electrode and two diphenylamine–thienylcarbazole moieties as an electron-donating unit at the 3- and 5-positions on the BODIPY core. It was found that for the absorption spectra of OMK-PY and OMK-CA adsorbed on TiO2 film the two dyes show a strong and broad absorption band in the range of 600 to 850 nm, and the onset of the absorption band reached 900 nm, that is, OMK-PY and OMK-CA possess the NIR adsorption ability as well as the panchromatic adsorption ability. Consequently, we demonstrate that (D)2–π–A BODIPY structure having two diphenylamine–thienylcarbazole moieties as strong electron-donating units at the 3- and 5-positions on the BODIPY core is an effective strategy to lead a high LHE in the range of visible light to NIR light, although the DSSCs based on (D)2–π–A type BODIPY dyes that have been developed in this current stage showed low photovoltaic performances due to the low LUMO level.
Experimental
General
Melting points were measured with a Yanaco micro melting point apparatus MP model. IR spectra were recorded on a SHIMADZU IRAffinity-1 spectrometer by ATR method. High-resolution mass spectral data were acquired on a Thermo Fisher Scientific LTQ Orbitrap XL. 1H NMR and 13C NMR spectra were recorded on a Varian-400 (400 MHz) or a Varian-500 (500 MHz) FT NMR spectrometer. Absorption spectra were observed with a Shimadzu UV-3150 spectrophotometer. Cyclic voltammetry (CV) curves were recorded in DMF/Bu4NClO4 (0.1 M) solution with a three-electrode system consisting of Ag/Ag+ as reference electrode, Pt plate as working electrode, and Pt wire as counter electrode by using a AMETEK Versa STAT 4 potentiostat. The potentials were referenced to ferrocene/ferrocenium (Fc/Fc+) as the internal reference. The HOMO and LUMO energy levels of OMK-PY and OMK-CA were evaluated from the spectral analyses and the CV data. Thus, the HOMO energy level was evaluated from the Eox1/2. The LUMO energy level was estimated from the Eox1/2 and the onset of photoabsorption spectra (860 nm; 1.44 eV for both OMK-PY and OMK-CA) in THF, that is, the LUMO energy level was obtained through eq. −[E0–0 − HOMO], where E0–0 transition energy is the onset of photoabsorption spectra corresponding to the energy gap between HOMO and LUMO.
Synthesis
2,2′-((5-(4,4,5,5-Tetramethyl-1,3-dioxolan-2-yl)thiophen-2-yl)methylene)bis(1H-pyrrole) (2). To a dichloromethane solution (40 ml) of 1 (ref. 26) (0.1 g, 0.42 mmol) and pyrrole (0.06 ml, 0.88 mmol) under an argon atmosphere was added trifluoroacetic acid (0.0005 ml) and stirred for 2 h at room temperature. The reaction mixture was diluted with water, and then the solution was extracted with dichloromethane. The dichloromethane extract was dried over MgSO4, filtrated, and concentrated. The residue was chromatographed on silica gel (dichloromethane–ethyl acetate = 9
:
1 as eluent) to give 2 (0.07 g, yield 50%) as a yellow viscous solid; IR (ATR):
= 3358, 2976, 1670, 1553 cm−1; 1H NMR (500 MHz, CDCl3) δ = 1.29 (d, 12H), 5.71 (s, 1H), 6.04 (br, 2H), 6.12 (s, 1H), 6.14–6.16 (m, 2H), 6.69–6.70 (m, 2H), 6.74 (d, J = 3.6 Hz, 1H), 7.00 (d, J = 3.6 Hz, 1H) ppm; 13C NMR (125 MHz, CDCl3) δ = 22.22, 24.54, 39.54, 83.17, 96.86, 107.22, 108.59, 117.54, 125.30, 126.43, 131.74, 142.59, 146.80 ppm; HRMS (ESI): m/z (%): [M + H+] calcd for C20H25N2O2S, 357.16313; found 357.16336.
(E)-2-Chloro-5-((5-chloro-2H-pyrrol-2-ylidene)(5-(4,4,5,5-tetramethyl-1,3-dioxolan-2-yl)thiophen-2-yl)methyl)-1H-pyrrole (3). To compound 2 (2.68 g, 7.52 mmol) under an argon atmosphere was added THF (40 ml) and then stirred at −75 °C. Next, a solution of N-chlorosuccinimide (2.01 g, 15.1 mmol) in THF (30 ml) was added dropwise and the solution was stirred at −75 °C for 1 h and then at room temperature for 2 h. After concentrating under reduced pressure, the resulting residue was dissolved in dichloromethane and washed with water. To the dichloromethane extract (150 ml) was added DDQ (1.71 g, 7.52 mmol) and the solution was stirred at room temperature for 2 h. After concentrating under reduced pressure, the residue was chromatographed on silica gel (dichloromethane as eluent) to give 3 (1.54 g, yield 49%) as a reddish brown solid; mp 89–91 °C; IR (ATR):
= 2967, 1560, 1420 cm−1; 1H NMR (500 MHz, CDCl3) δ = 1.33 (d, 12H), 6.21 (s, 1H), 6.29 (d, J = 4.3 Hz, 2H), 6.90 (d, J = 4.3 Hz, 2H), 7.19 (br, 2H) ppm; 13C NMR (125 MHz, CDCl3) δ = 22.20, 24.40, 83.57, 96.58, 117.18, 126.28, 130.33, 131.53, 132.40, 136.98, 138.44, 142.12, 147.64 ppm; HRMS (ESI): m/z (%): [M + H+] calcd for C20H21N2O2Cl2S, 423.06953; found 423.06921.
3,7-Dichloro-5,5-difluoro-10-(5-(4,4,5,5-tetramethyl-1,3-dioxolan-2-yl)thiophen-2-yl)-5H-dipyrrolo[1,2-c:2′,1′-f][1,3,2]diazaborinine (4). To a compound 3 (1.5 g, 3.55 mmol) under an argon atmosphere was added dichloromethane (100 ml) and triethylamine (4.72 ml, 35.5 mmol) and stirred for 30 min at room temperature. Next, BF3–OEt2 (6.25 ml, 53.3 mmol) was added dropwise and the solution was stirred at room temperature for 12 h. The reaction mixture was washed with water and extracted with dichloromethane. The dichloromethane extract was dried over MgSO4, filtrated, and concentrated. The residue was chromatographed on silica gel (dichloromethane–hexane = 1
:
2 as eluent) to give 4 (1.11 g, yield 67%) as a dark red solid; mp 185–187 °C; IR (ATR):
= 2982, 1545, 1381 cm−1; 1H NMR (500 MHz, acetone-d6) δ = 1.31 (s, 12H), 6.26 (s, 1H), 6.71 (d, J = 4.4 Hz, 2H), 7.42 (d, J = 3.8 Hz, 1H), 7.43 (d, J = 4.4 Hz, 2H), 7.65 (d, J = 3.8 Hz, 1H) ppm; 13C NMR (125 MHz, acetone-d6) δ = 22.34, 24.51, 84.17, 96.90, 119.96, 128.16, 133.17, 133.34, 133.95, 134.73, 144.76, 152.63, 153.81 ppm; HRMS (ESI): m/z (%): [M + Na+] calcd for C20H19N2O2BF2Cl2SNa, 493.04976; found 493.04974.
7,7′-((5,5-Difluoro-10-(5-(4,4,5,5-tetramethyl-1,3-dioxolan-2-yl)thiophen-2-yl)-5H-dipyrrolo[1,2-c:2′,1′-f][1,3,2]diazaborinine-3,7-diyl)bis(thiophene-5,2-diyl))bis(9-butyl-N,N-diphenyl-9H-carbazol-2-amine) (6). A solution of 4 (1.0 g, 2.13 mmol), 5 (ref. 18g) (2.71 g, 4.25 mmol), and Pd(PPh3)4 (2.94 g, 2.54 mmol) in toluene (270 ml) was stirred for 12 h at 110 °C under an argon atmosphere. After concentrating under reduced pressure, the resulting residue was dissolved in dichloromethane and washed with water. The dichloromethane extract was dried over MgSO4, filtrated, and concentrated. The residue was chromatographed on silica gel (chloroform–hexane = 1
:
2 as eluent) to give 6 (1.99 g, yield 70%) as a blackish green solid; mp 176–177 °C; IR (ATR):
= 2955, 1591, 1431 cm−1; 1H NMR (400 MHz, acetone-d6) δ = 0.88 (t, J = 7.4 Hz, 6H), 1.32–1.34 (m, 16H), 1.77–1.84 (m, 4H), 4.37 (t, J = 7.0 Hz, 4H), 6.29 (s, 1H), 6.94 (dd, J = 1.8 and 8.4 Hz, 2H), 7.04–7.08 (m, 4H), 7.13–7.14 (m, 10H), 7.23 (d, J = 1.8 Hz, 2H), 7.30–7.34 (m, 10H), 7.40 (d, J = 3.8 Hz, 1H), 7.55 (d, J = 3.7 Hz, 1H), 7.64 (dd, J = 1.5 and 8.1 Hz, 2H), 7.76 (d, J = 4.1 Hz, 2H), 7.95 (d, J = 1.2 Hz, 2H), 8.06 (d, J = 8.4 Hz, 2H), 8.15 (d, J = 8.0 Hz, 2H), 8.31 (d, J = 4.1 Hz, 2H) ppm; 13C NMR (125 MHz, acetone-d6) δ = 14.18, 21.02, 22.38, 24.60, 31.84, 43.07, 84.02, 97.12, 105.79, 106.95, 117.90, 118.44, 119.25, 121.22, 121.60, 122.04, 123.65, 124.26, 124.88, 126.15, 126.47, 127.67, 130.18, 130.79, 131.21, 132.77, 133.72, 133.91, 135.69, 137.40, 142.26, 143.43, 147.74, 149.12, 150.10, 150.32, 150.98 ppm; HRMS (ESI): m/z (%): [M + Na+] calcd for C84H73N6O2BF2S3Na, 1365.49105; found 1365.49304.
5-(3,7-Bis(5-(9-butyl-7-(diphenylamino)-9H-carbazol-2-yl)thiophen-2-yl)-5,5-difluoro-5H-dipyrrolo[1,2-c:2′,1′-f][1,3,2]diazaborinin-10yl)thiophene-2-carbaldehyde (7). To a solution of 6 (1.85 g, 1.38 mmol) in THF (20 ml) was added 2 N HCl (13.8 ml) and refluxed for 12 h. The reaction mixture was washed with water and extracted with dichloromethane. The dichloromethane extract was dried over MgSO4, filtrated, and concentrated. The residue was chromatographed on silica gel (dichloromethane–hexane = 1
:
1 as eluent) to give 7 (1.71 g, yield 99%) as a dark green solid; mp 171–172 °C; IR (ATR):
= 2953, 1665, 1585 cm−1; 1H NMR (500 MHz, acetone-d6) δ = 0.88 (t, J = 7.4 Hz, 6H), 1.29–1.36 (m, 4H), 1.78–1.83 (m, 4H), 4.37 (t, J = 6.9 Hz, 4H), 6.94 (dd, J = 1.9 and 8.4 Hz, 2H), 7.05–7.07 (m, 4H), 7.13–7.15 (m, 8H), 7.17 (d, J = 4.8 Hz, 2H), 7.23 (d, J = 1.8 Hz, 2H), 7.28–7.33 (m, 10H), 7.65 (dd, J = 1.5 and 8.1 Hz, 2H), 7.78 (d, J = 4.0 Hz, 2H), 7.81 (d, J = 3.5 Hz, 1H), 7.96 (d, J = 1.0 Hz, 2H), 8.07 (d, J = 8.4 Hz, 2H), 8.15 (d, J = 8.1 Hz, 2H), 8.18 (d, J = 3.5 Hz, 1H), 8.35 (d, J = 3.8 Hz, 2H), 10.12 (s, –CHO) ppm; 13C NMR (125 MHz, acetone-d6) δ = 14.18, 21.02, 31.85, 43.08, 105.77, 107.01, 117.91, 118.46, 119.21, 121.24, 122.07, 123.67, 124.37, 124.89, 126.29, 130.18, 130.56, 131.11, 133.53, 133.82, 137.44, 137.61, 142.25, 143.46, 143.57, 147.81, 149.10, 150.99, 151.65, 184.42 ppm (four aromatic carbon signals were not observed owing to overlapping resonances); HRMS (ESI): m/z (%): [M + H+] calcd for C76H62N6OBF2S3, 1243.42029; found 1243.42139.
3-(5-(3,7-Bis(5-(9-butyl-7-(diphenylamino)-9H-carbazol-2-yl)thiophen-2-yl)-5,5-difluoro-5H-4dipyrrolo[1,2-c:2′,1′-f][1,3,2]diazaborinin-10-yl)thiophen-2-yl)-2-(pyridin-4-yl)acrylonitrile (OMK-PY). To a solution of 4-pyridylacetonitrile hydrochloride (0.05 g, 0.322 mmol) in dichloromethane (3 ml) was added triethylamine (0.21 ml, 1.61 mmol) and stirred for 20 min at 0 °C. Next, 7 (0.4 g, 0.322 mmol) was added and the solution was stirred at room temperature for 5 days. The reaction mixture was washed with water and extracted with dichloromethane. The dichloromethane extract was dried over MgSO4, filtrated, and concentrated. The residue was chromatographed on silica gel (dichloromethane and then ethyl acetate as eluent) to give OMK-PY (0.171 g, yield 40%) as a dark green solid; mp 169–170 °C; IR (ATR):
= 2924, 1585, 1429 cm−1; 1H NMR (500 MHz, acetone-d6) δ = 0.88 (t, J = 7.4 Hz, 6H), 1.30–1.35 (m, 4H), 1.79–1.82 (m, 4H), 4.37 (t, J = 6.6 Hz, 4H), 6.94 (d, J = 8.3 Hz, 2H), 7.04–7.07 (m, 4H), 7.13–7.14 (m, 8H), 7.18 (br, 2H), 7.23 (s, 2H), 7.30–7.33 (m, 10H), 7.64 (d, J = 8.9 Hz, 2H), 7.75–7.78 (m, 5H), 7.96 (s, 2H), 8.05–8.07 (m, 3H), 8.15 (d, J = 8.9 Hz, 2H), 8.34 (br, 2H), 8.58 (s, 1H), 8.73 (d, J = 5.9 Hz, 2H) ppm; 13C NMR (125 MHz, acetone-d6) δ = 14.18, 21.02, 31.85, 43.09, 105.78, 107.01, 117.49, 117.74, 117.92, 118.46, 119.22, 120.53, 121.25, 122.07, 123.67, 124.36, 124.89, 125.99, 126.28, 129.64, 130.18, 130.51, 131.15, 133.63, 133.77, 134.27, 137.93, 142.26, 143.46, 147.80, 149.11, 151.65 ppm (seven aromatic carbon signals were not observed owing to overlapping resonances); HRMS (ESI): m/z (%): [M + H+] calcd for C85H66N8BF2S3, 1343.46282; found 1343.46300.
3-(5-(3,7-Bis(5-(9-butyl-7-(diphenylamino)-9H-carbazol-2-yl)thiophen-2-yl)-5,5-difluoro-5H-dipyrrolo[1,2-c:2′,1′-f][1,3,2]diazaborinin-10-yl)thiophen-2-yl)-2-cyanoacrylic acid (OMK-CA). A solution of 7 (0.4 g, 0.322 mmol), cyanoacetic acid (0.11 g, 0.644 mmol) and piperidine (0.032 ml, 0.322 mmol) in chloroform/acetonitrile (1
:
1, 65 ml) was refluxed for 22 h. After concentrating under reduced pressure, the resulting residue was chromatographed on silica gel (ethyl acetate and then ethyl acetate containing 5% acetic acid as eluent) to give the mixture OMK-CA and unreacted cyanoacetic acid. The mixture was dissolved in dichloromethane and washed with water. The dichloromethane extract was dried over MgSO4, filtrated, and concentrated to give OMK-CA (0.256 g, yield 61%) as a dark green solid; mp 176–179 °C; IR (ATR):
= 2922, 1688, 1578, 1424 cm−1; 1H NMR (500 MHz, DMSO-d6) δ = 0.79 (t, J = 7.5 Hz, 6H), 1.17–1.23 (m, 4H), 1.62–1.68 (m, 4H), 4.29 (t, J = 6.8 Hz, 4H), 6.87 (dd, J = 1.6 and 8.5 Hz, 2H), 7.03–7.08 (m, 12H), 7.15 (d, J = 1.5 Hz, 2H), 7.26 (d, J = 4.5 Hz, 2H), 7.29–7.33 (m, 10H), 7.60 (d, J = 9.1 Hz, 2H), 7.84 (d, J = 3.9 Hz, 1H), 7.90 (d, J = 4.1 Hz, 2H), 7.95 (s, 2H), 8.07 (d, J = 8.5 Hz, 2H), 8.13–8.15 (m, 3H), 8.24 (d, J = 4.2 Hz, 2H), 8.58 (br, –COOH) ppm; unfortunately, it was difficult to obtain the 13C NMR spectrum of OMK-CA because of its poor solubility in deuterated solvent; HRMS (ESI): m/z (%): [M+] calcd for C81H62N7O2BF2S3, 1309.41827; found 1309.42188.
Preparation of DSSCs
The TiO2 paste (JGC Catalysts and Chemicals Ltd., PST-18NR) was deposited on a fluorine-doped-tin-oxide (FTO) substrate by doctor-blading, and sintered for 50 min at 450 °C. The 9 μm thick TiO2 electrode was immersed into 0.1 mM dye solution in THF for 15 hours enough to adsorb the dye sensitizers (OMK-PY and OMK-CA). The DSSCs were fabricated by using the TiO2 electrode (0.5 × 0.5 cm2 in photoactive area) thus prepared, Pt-coated glass as a counter electrode, and a solution of 0.05 M iodine, 0.1 M lithium iodide, and 0.6 M 1,2-dimethyl-3-propylimidazolium iodide in acetonitrile as electrolyte. The photocurrent–voltage characteristics were measured using a potentiostat under a simulated solar light (AM 1.5, 100 mW cm−2). IPCE spectra were measured under monochromatic irradiation with a tungsten–halogen lamp and a monochromator. The amount of dye molecules adsorbed on TiO2 film was determined form the calibration curve by absorption spectral measurement of the concentration change of the dye solution before and after adsorption. The quantification of dye was made based on the molar extinction coefficient for λabsmax of dye in the above solution. Absorption spectra of the dyes adsorbed on TiO2 film (3 μm) were recorded on the dyes-adsorbed TiO2 film in the transmission mode with a calibrated integrating sphere system.
Acknowledgements
This work was supported by Grants-in-Aid for Scientific Research (B) from the Japan Society for the Promotion of Science (JSPS) KAKENHI Grant Number 15H03859, by Matching Planner Program (MP27115659061) from Japan Science and Technology Agency (JST) and by Yazaki Memorial Foundation for Science and Technology.
Notes and references
- A. Mishra, M. K. R. Fischer and P. Bäuerle, Angew. Chem., Int. Ed., 2009, 48, 2474 CrossRef CAS PubMed.
-
(a) Y. Ooyama and Y. Harima, Eur. J. Org. Chem., 2009, 18, 2903 CrossRef;
(b) Y. Ooyama and Y. Harima, ChemPhysChem, 2012, 13, 4032 CrossRef CAS PubMed.
-
(a) Z. Ning and H. Tian, Chem. Commun., 2009, 5483 RSC;
(b) Z. Ning, Y. Fu and H. Tian, Energy Environ. Sci., 2010, 3, 1170 RSC.
- A. Hagfeldt, G. Boschloo, L. Sun, L. Kloo and H. Pettersson, Chem. Rev., 2010, 110, 6595 CrossRef CAS PubMed.
- N. Manfredi, B. Cecconi and A. Abbotto, Eur. J. Org. Chem., 2014, 7069 CrossRef CAS.
- L. Zhang and J. M. Cole, ACS Appl. Mater. Interfaces, 2015, 7, 3427 CAS.
- C.-P. Lee, R. Y.-Y. Lin, L.-Y. Lin, C.-T. Li, T.-C. Chu, S.-S. Sun, J. T. Lin and K.-C. Ho, RSC Adv., 2015, 5, 23810 RSC.
- B. Pashaei, H. Shahroosvand, M. Graetzel and M. K. Nazeeruddin, Chem. Rev., 2016, 116, 9485 CrossRef CAS PubMed.
- B. O'Regan and M. Grätzel, Nature, 1991, 353, 737 CrossRef.
-
(a) H. Imahori, T. Umeyama and S. Ito, Acc. Chem. Res., 2009, 42, 1809 CrossRef CAS PubMed;
(b) L.-L. Li and E. W.-G. Diau, Chem. Soc. Rev., 2013, 42, 291 RSC;
(c) K. Ladomenou, T. N. Kitsopoulos, G. D. Sharma and A. G. Coutsolelos, RSC Adv., 2014, 4, 21379 RSC;
(d) T. Higashino and H. Imahori, Dalton Trans., 2015, 44, 448 RSC.
-
(a) T. Bessho, S. M. Zakeeruddin, C.-Y. Yeh, E. W.-G. Diau and M. Grätzel, Angew. Chem., Int. Ed., 2010, 49, 6646 CrossRef CAS PubMed;
(b) A. Yella, H.-W. Lee, H. N. Tsao, C. Yi, A. K. Chandiran, M. K. Nazeeruddin, E. W.-G. Diau, C.-Y. Yeh, S. M. Zakeeruddin and M. Grätzel, Science, 2011, 334, 629 CrossRef CAS PubMed;
(c) S. Mathew, A. Yella, P. Gao, R. Humphry-Baker, B. F. E. Curchod, N. Ashari-Astani, I. Tavernelli, U. Rothlisberger, M. K. Nazeeruddin and M. Grätzel, Nat. Chem., 2014, 6, 242 CrossRef CAS PubMed;
(d) A. Yella, C.-L. Mai, S. M. Zakeeruddin, S.-N. Chang, C.-H. Hsieh, C.-Y. Yeh and M. Grätzel, Angew. Chem., Int. Ed., 2014, 53, 2973 CrossRef CAS PubMed;
(e) T. Higashino, Y. Fujimori, K. Sugiura, Y. Tsuji, S. Ito and H. Imahori, Angew. Chem., Int. Ed., 2015, 54, 9052 CrossRef CAS PubMed;
(f) J. P. Hill, Angew. Chem., Int. Ed., 2016, 55, 2976 CrossRef CAS PubMed;
(g) F. Lodermeyer, R. D. Costa, J. Malig, N. Jux and D. M. Guldi, Chem.–Eur. J., 2016, 22, 7851 CrossRef CAS PubMed;
(h) G. Copley, D. Hwang, D. Kim and A. Osuka, Angew. Chem., Int. Ed., 2016, 55, 10287 CrossRef CAS PubMed.
-
(a) T. Zhang, X. Qian, P. Zhang, Y.-Z. Zhu and J.-Y. Zheng, Chem. Commun., 2015, 51, 3782 RSC;
(b) Y. Xie, Y. Tang, W. Wu, Y. Wang, J. Liu, X. Li, H. Tian and W.-H. Zhu, J. Am. Chem. Soc., 2015, 137, 14055 CrossRef CAS PubMed;
(c) K. Sirithip, N. Prachumrak, R. Rattanawan, T. Keawin, T. Sudyoadsuk, S. Namuangruk, S. Jungsuttiwong and V. Promarak, Chem.–Asian J., 2015, 10, 882 CrossRef CAS PubMed;
(d) S. Mathew, N. A. Astani, B. F. E. Curchod, J. H. Delcamp, M. Marszalek, J. Frey, U. Rothlisberger, M. K. Nazeeruddina and M. Grätzel, J. Mater. Chem. A, 2016, 4, 2332 RSC;
(e) L. Zeininger, F. Lodermeyer, R. D. Costa, D. M. Guldi and A. Hirsch, Chem. Commun., 2016, 52, 8842 RSC;
(f) J. Luo, J. Zhang, K.-W. Huang, Q. Qi, S. Dong, J. Zhang, P. Wang and J. Wu, J. Mater. Chem. A, 2016, 4, 8428 RSC;
(g) C. Li, L. Luo, D. Wu, R. Jiang, J. Lan, R. Wang, L. Huang, S. Yang and J. You, J. Mater. Chem. A, 2016, 4, 11829 RSC;
(h) Y.-C. Liu, H.-H. Chou, F.-Y. Ho, H.-J. Wei, T.-C. Wei and C.-Y. Yeh, J. Mater. Chem. A, 2016, 4, 11878 RSC.
-
(a) J.-J. Cid, J.-H. Yum, S.-R. Jang, M. K. Nazeeruddin, E. Martínez-Ferrero, E. Palomares, J. Ko, M. Grätzel and T. Torres, Angew. Chem., Int. Ed., 2007, 46, 8358 CrossRef CAS PubMed;
(b) M. Kimura, H. Nomoto, N. Masaki and S. Mori, Angew. Chem., Int. Ed., 2012, 51, 4371 CrossRef CAS PubMed;
(c) M.-E. Ragoussi, J.-J. Cid, J.-H. Yum, G. De La Torre, D. D. Censo, M. Grätzel, M. K. Nazeeruddin and T. Torres, Angew. Chem., Int. Ed., 2012, 51, 4375 CrossRef CAS PubMed;
(d) L. Yu, K. Fan, T. Duan, X. Chen, R. Li and T. Peng, ACS Sustainable Chem. Eng., 2014, 2, 718 CrossRef CAS;
(e) T. Ikeuchi, H. Nomoto, N. Masaki, M. J. Griffith, S. Mori and M. Kimura, Chem. Commun., 2014, 50, 1941 RSC;
(f) T. Ikeuchi, S. Agrawal, M. Ezoe, S. Mori and M. Kimura, Chem.–Asian J., 2015, 10, 2347 CrossRef CAS PubMed;
(g) L. Tejerina, M. V. Martínez-Díaz, M. K. Nazeeruddin and T. Torres, Chem.–Eur. J., 2016, 22, 4369 CrossRef CAS PubMed.
-
(a) X. Wang, J. Yang, H. Yu, F. Li, L. Fan, W. Sun, Y. Liu, Z. Y. Koh, J. Pan, W.-L. Yim, L. Yan and Q. Wang, Chem. Commun., 2014, 50, 3965 RSC;
(b) S.-G. Li, K.-J. Jiang, J.-H. Huang, L.-M. Yang and Y.-L. Song, Chem. Commun., 2014, 50, 4309 RSC;
(c) D. K. Panda, F. S. Goodson, S. Ray and S. Saha, Chem. Commun., 2014, 50, 5358 RSC;
(d) K. Kakiage, Y. Aoyama, T. Yano, T. Otsuka, T. Kyomen, M. Unno and M. Hanaya, Chem. Commun., 2014, 50, 6379 RSC;
(e) A. Amacher, C. Yi, J. Yang, M. P. Bircher, Y. Fu, M. Cascella, M. Grätzel, S. Decurtins and S.-X. Liu, Chem. Commun., 2014, 50, 6540 RSC;
(f) W.-I. Hung, Y.-Y. Liao, T.-H. Lee, Y.-C. Ting, J.-S. Ni, W.-S. Kao, J. T. Lin, T.-C. Wei and Y.-S. Yen, Chem. Commun., 2015, 51, 2152 RSC;
(g) X. Li, Z. Zheng, W. Jiang, W. Wu, Z. Wang and H. Tian, Chem. Commun., 2015, 51, 3590 RSC;
(h) K. Kakiage, Y. i. Aoyama, T. Yano, K. Oya, T. Kyomen and M. Hanaya, Chem. Commun., 2015, 51, 6315 RSC;
(i) L. Yang, Z. Zheng, Y. Li, W. Wu, H. Tian and Z. Wang, Chem. Commun., 2015, 51, 4842 RSC;
(j) N. Shibayama, Y. Inoue, M. Abe, S. Kajiyama, H. Ozawa, H. Miura and H. Arakawa, Chem. Commun., 2015, 51, 12795 RSC;
(k) K. Kakiage, Y. Aoyama, T. Yano, K. Oya, J. Fujisawa and M. Hanaya, Chem. Commun., 2015, 51, 15894 RSC.
-
(a) J.-S. Ni, Y.-C. Yen and J. T. Lin, Chem. Commun., 2015, 51, 17080 RSC;
(b) Y.-D. Lin, B.-Y. Ke, Y. J. Chang, P.-T. Chou, K.-L. Liau, C.-Y. Liu and T. J. Chow, J. Mater. Chem. A, 2015, 3, 16831 RSC;
(c) S. S. Soni, K. B. Fadadu, J. V. Vaghasiya, B. G. Solanki, K. K. Sonigara, A. Singh, D. Das and P. K. Iyer, J. Mater. Chem. A, 2015, 3, 21664 RSC;
(d) X. Li, Y. Hu, I. Sanchez-Molina, Y. Zhou, F. Yu, S. A. Haque, W. Wu, J. Hua, H. Tian and N. Robertson, J. Mater. Chem. A, 2015, 3, 2173 Search PubMed;
(e) Y. Hu, A. Ivaturi, M. Planells, C. L. Boldrini, A. O. Biroli and N. Robertson, J. Mater. Chem. A, 2016, 4, 2509 RSC;
(f) H. Wu, L. Yang, Y. Li, M. Zhang, J. Zhang, Y. Guo and P. Wang, J. Mater. Chem. A, 2016, 4, 519 RSC;
(g) J. Wu, G. Li, L. Zhang, G. Zhou and Z.-S. Wang, J. Mater. Chem. A, 2016, 4, 3342 RSC;
(h) J.-S. Ni, Y.-C. Yen and J. T. Lin, J. Mater. Chem. A, 2016, 4, 6553 RSC;
(i) A. J. Huckaba, A. Yella, P. Brogdon, J. S. Murphy, M. K. Nazeeruddin, M. Grätzel and J. H. Delcamp, Chem. Commun., 2016, 52, 8424 RSC;
(j) T.-Y. Li, C. Su, S. B. Akula, W.-G. Sun, H.-M. Chien and W.-R. Li, Org. Lett., 2016, 18, 3386 CrossRef CAS PubMed;
(k) Y. Gao, X. Li, Y. Hu, Y. Fan, J. Yuan, N. Robertson, J. Hua and S. R. Marder, J. Mater. Chem. A, 2016, 4, 12865 RSC.
-
(a) Z. Yao, M. Zhang, H. Wu, L. Yang, R. Li and P. Wang, J. Am. Chem. Soc., 2015, 137, 3799 CrossRef CAS PubMed;
(b) N. Zhou, K. Prabakaran, B. Lee, S. H. Chang, B. Harutyunyan, P. Guo, M. R. Butler, A. Timalsina, M. J. Bedzyk, M. A. Ratner, S. Vegiraju, S. Yau, C.-G. Wu, R. P. H. Chang, A. Facchetti, M.-C. Chen and T. J. Marks, J. Am. Chem. Soc., 2015, 137, 4414 CrossRef CAS PubMed;
(c) Y.-S. Yen, J.-S. Ni, T.-Y. Lin, W.-I. Hung, J. T. Lin and M.-C. P. Yeh, Eur. J. Org. Chem., 2015, 7367 CrossRef CAS;
(d) H. Jiang, G. Ferrara, X. Zhang, K. Oniwa, A. Islam, L. Han, Y.-J. Sun, M. Bao, N. Asao, Y. Yamamoto and T. Jin, Chem.–Eur. J., 2015, 21, 4065 CrossRef CAS PubMed;
(e) K. Matsumura, S. Yoshizaki, M. M. Maitani, Y. Wada, Y. Ogomi, S. Hayase, T. Kaiho, S. Fuse, H. Tanaka and T. Takahashi, Chem.–Eur. J., 2015, 21, 9742 CrossRef CAS PubMed;
(f) K. D. Seo, I. T. Choi and H. K. Kim, Chem.–Eur. J., 2015, 21, 14804 CrossRef CAS PubMed;
(g) B. Liu, F. Giordano, K. Pei, J.-D. Decoppet, W.-H. Zhu, S. M. Zakeeruddin and M. Grätzel, Chem.–Eur. J., 2015, 21, 18654 CrossRef CAS PubMed;
(h) Z. Yao, M. Zhang, R. Li, L. Yang, Y. Qiao and P. Wang, Angew. Chem., Int. Ed., 2015, 54, 5994 CrossRef CAS PubMed;
(i) P. Brogdon, F. Giordano, G. A. Puneky, A. Dass, S. M. Zakeeruddin, M. K. Nazeeruddin, M. Grätzel, G. S. Tschumper and J. H. Delcamp, Chem.–Eur. J., 2016, 22, 694 CrossRef CAS PubMed;
(j) S. Irie, S. Fuse, M. M. Maitani, Y. Wada, Y. Ogomi, S. Hayase, T. Kaiho, H. Masui, H. Tanaka and T. Takahashi, Chem.–Eur. J., 2016, 22, 2507 CrossRef CAS PubMed;
(k) F.-L. Guo, Z.-Q. Li, X.-P. Liu, L. Zhou, F.-T. Kong, W.-C. Chen and S.-Y. Dai, Adv. Funct. Mater., 2016, 26, 5733 CrossRef CAS.
- J. Mao, N. He, Z. Ning, Q. Zhang, F. Guo, L. Chen, W. Wu, J. Hua and H. Tian, Angew. Chem., Int. Ed., 2012, 51, 9873 CrossRef CAS PubMed.
-
(a) Y. Ooyama, S. Inoue, R. Asada, G. Ito, K. Kushimoto, K. Komaguchi, I. Imae and Y. Harima, Eur. J. Org. Chem., 2010, 92 CrossRef CAS;
(b) Y. Ooyama, S. Inoue, T. Nagano, K. Kushimoto, J. Ohshita, I. Imae, K. Komaguchi and Y. Harima, Angew. Chem., Int. Ed., 2011, 50, 7429 CrossRef CAS PubMed;
(c) Y. Ooyama, T. Nagano, S. Inoue, I. Imae, K. Komaguchi, J. Ohshita and Y. Harima, Chem.–Eur. J., 2011, 17, 14837 CrossRef CAS PubMed;
(d) Y. Ooyama, N. Yamaguchi, I. Imae, K. Komaguchi, J. Ohshita and Y. Harima, Chem. Commun., 2013, 49, 2548 RSC;
(e) Y. Ooyama, Y. Hagiwara, T. Mizumo, Y. Harima and J. Ohshita, New J. Chem., 2013, 37, 2479 RSC;
(f) Y. Ooyama, T. Sato, Y. Harima and J. Ohshita, J. Mater. Chem. A, 2014, 2, 3293 RSC;
(g) Y. Ooyama, K. Uenaka and J. Ohshita, Eur. J. Org. Chem., 2015, 3713 CrossRef CAS;
(h) Y. Ooyama, K. Uenaka, T. Kamimura, S. Ozako, M. Kanda, T. Koide and F. Tani, RSC Adv., 2016, 6, 16150 RSC;
(i) Y. Ooyama, K. Furue, T. Enoki, M. Kanda, Y. Adachi and J. Ohshita, Phys. Chem. Chem. Phys., 2016, 18, 30662 RSC;
(j) Y. Ooyama, N. Yamaguchi, J. Ohshita and Y. Harima, Phys. Chem. Chem. Phys., 2016, 18, 32992 RSC.
-
(a) Y. Harima, T. Fujita, Y. Kano, I. Imae, K. Komaguchi, Y. Ooyama and J. Ohshita, J. Phys. Chem. C, 2013, 117, 16364 CrossRef CAS;
(b) N. Shibayama, H. Ozawa, M. Abe, Y. Ooyama and H. Arakawa, Chem. Commun., 2014, 50, 6398 RSC;
(c) Y. Ooyama, K. Uenaka, T. Sato, N. Shibayama and J. Ohshita, RSC Adv., 2015, 5, 2531 RSC;
(d) J. Ohshita, Y. Adachi, D. Tanaka, M. Nakashima and Y. Ooyama, RSC Adv., 2015, 5, 36673 RSC;
(e) Y. Harima, Y. Kano, T. Fujita, I. Imae, Y. Ooyama and J. Ohshita, RSC Adv., 2015, 5, 71387 RSC;
(f) Y. Adachi, Y. Ooyama, N. Shibayama and J. Ohshita, Dalton Trans., 2016, 45, 13817 RSC.
-
(a) D. Daphnomili, G. D. Sharma, S. Biswas, T. K. R. Justin and A. G. Goutsolelos, J. Photochem. Photobiol., A, 2013, 253, 88 CrossRef CAS;
(b) J. Lu, X. Xu, Z. Li, k. Cao, J. Cui, Y. Zhang, Y. Shen, Y. Li, J. Zhu, S. Dai, W. Chjen, Y. Cheng and M. Wang, Chem.–Asian J., 2013, 8, 956 CrossRef CAS PubMed;
(c) M.-D. Zhang, H.-X. Xie, X.-H. Ju, L. Qin, Q.-X. Yang, H.-G. Zheng and X.-F. Zhou, Phys. Chem. Chem. Phys., 2013, 15, 634 RSC;
(d) D. Daphnomili, G. Landrou, P. Singh, A. Thomas, K. Yesudas, B. K. G. D. Sharma and A. G. Goutsolelos, RSC Adv., 2012, 2, 12899 RSC;
(e) L. Wang, X. yang, S. Li, M. Cheng and L. Sun, RSC Adv., 2013, 3, 13677 RSC;
(f) T. Sakurada, Y. Arai and H. Segawa, RSC Adv., 2014, 4, 13201 RSC;
(g) J. Mao, D. Wang, S.-H. Liu, Y. Hang, Y. Xu, Q. Zhang, W. Wu, P.-T. Chou and J. Hua, Asian J. Org. Chem., 2014, 3, 153 CrossRef CAS;
(h) T. Ikeuchi, S. Agrawal, M. Ezoe, S. Mori and M. Kimura, Chem.–Asian J., 2015, 10, 2347 CrossRef CAS PubMed.
-
(a) C. Stangel, A. Bagaki, P. A. Angaridis, G. Charalambidis, G. D. Sharma and A. G. Coutsolelos, Inorg. Chem., 2014, 53, 11871 CrossRef CAS PubMed;
(b) M. N. K. P. Bolisetty, C.-T. Li, K. R. J. Thomas, G. B. Bodedla and K.-C. Ho, Tetrahedron, 2014, 70, 4203 Search PubMed;
(c) D. Franchi, M. Calamante, G. Reginato, L. Zani, M. Peruzzini, M. Taddei, F. F. de Biani, R. Basosi, A. Sinicropi, D. Colonna, A. Di Carlo and A. Mordin, Tetrahedron, 2014, 70, 6285 CrossRef CAS;
(d) E. V. Verbitskiy, E. M. Cheprakova, J. O. Subbotina, A. V. Schepochkin, P. A. Slepukhin, G. L. Rusinov, V. N. Charushin, O. N. Chupakhin, N. I. Makarova, A. V. Metelitsa and V. I. Minkin, Dyes Pigm., 2014, 100, 201 CrossRef CAS;
(e) C.-L. Mai, T. Moehl, C.-H. Hsieh, J.-D. Décoppet, S. M. Zakeeruddin, M. Grätzel and C.-Y. Yeh, ACS Appl. Mater. Interfaces, 2015, 7, 14975 CrossRef CAS PubMed;
(f) H. Jia, K. Shen, X. i. Ju, M. Zhanga and H. Zheng, New J. Chem., 2016, 40, 2799 RSC;
(g) P. A. Angaridis, E. Ferentinos, G. Charalambidis, K. Ladomenou, V. Nikolaou, S. Biswas, G. D. Sharma and A. G. Coutsolelos, RSC Adv., 2016, 6, 22187 RSC;
(h) U. Meinhardt, F. Lodermeyer, T. A. Schaub, A. Kunzmann, P. O. Dral, A. C. Sale, F. Hampel, D. M. Guldi, R. D. Costa and M. Kivala, RSC Adv., 2016, 6, 67372 RSC.
- H. He, A. Gurung and L. Si, Chem. Commun., 2012, 48, 5910 RSC.
-
(a) S. Hattori, K. Ohkubo, Y. Urano, H. Sunahara, T. Nagano, Y. Wada, N. V. Tkachenko, H. Lemmetyineu and S. Fukuzumi, J. Phys. Chem. B, 2005, 109, 15368 CrossRef CAS PubMed;
(b) S. Erten-Ela, M. D. Yilmaz, B. Icli, Y. Dede, S. Icli and E. U. Akkaya, Org. Lett., 2008, 10, 3299 CrossRef CAS PubMed;
(c) S. Kolemen, Y. Cakmak, S. Erten-Ela, Y. Altay, J. Brendel, M. Thelakkat and E. U. Akkaya, Org. Lett., 2010, 12, 3812 CrossRef CAS PubMed;
(d) S. Kolemen, O. A. Bozdemir, Y. Cakmak, G. Barin, S. Erten-Ela, M. Marszalek, J.-H. Yum, S. M. Zakeeruddin, M. K. Nazeeruddin, M. Grätzel and E. U. Akkaya, Chem. Sci., 2011, 2, 949 RSC;
(e) M. Mao, J.-B. Wang, Z.-F. Xiao, S.-Y. Dai and Q.-H. Song, Dyes Pigm., 2012, 94, 224 CrossRef CAS;
(f) J.-B. Wang, X.-Q. Fang, X. Pan, S.-Y. Dai and Q.-H. Song, Chem.–Asian J., 2012, 7, 696 CrossRef CAS PubMed.
-
(a) D. Hablot, A. Islam, L. Han and R. Ziessel, ChemPlusChem, 2012, 77, 462 CrossRef CAS;
(b) C. Qin, A. Mirloup, N. Leclerc, A. Islam, A. El-Shafei, L. Han and R. Ziessel, Adv. Energy Mater., 2014, 4, 1400085 CrossRef;
(c) M. Mao and Q.-H. Song, Chem. Rec., 2016, 16, 719 CrossRef CAS PubMed;
(d) J.-F. Lefebvre, X.-Z. Sun, J. A. Calladine, M. W. George and E. A. Gibson, Chem. Commun., 2014, 50, 5258 RSC;
(e) Y. Kubo, D. Eguchi, A. Matsumoto, R. Nishiyabu, H. Yakushiji, K. Shigaki and M. Kaneko, J. Mater. Chem. A, 2014, 2, 5204 RSC;
(f) C. J. Wood, G. H. Summers and E. A. Gibson, Chem. Commun., 2015, 51, 3915 RSC;
(g) B. L. M'Sabah, M. Boucharef, J. Warnan, Y. Pellegrin, E. Blart, B. Lucas, F. Odobel and J. Bouclé, Phys. Chem. Chem. Phys., 2015, 17, 9910 RSC;
(h) Y. Çakmak, S. Kolemen, M. Buyuktemiz, Y. Dede and S. Erten-Ela, New J. Chem., 2015, 39, 4086 RSC;
(i) Z. E. Galateia, N. Agapi, N. Vasilis, G. D. Sharma and C. G. Athanassios, J. Mater. Chem. C, 2015, 3, 5652 RSC;
(j) G. H. Summers, J.-F. Lefebvre, F. A. Black, E. S. Davies, E. A. Gibson, T. Pullerits, C. J. Wood and K. Zidek, Phys. Chem. Chem. Phys., 2016, 18, 1059 RSC.
-
(a) M. Mao, X.-L. Zhang, X.-Q. Fang, G.-H. Wu, Y. Ding, X.-L. Liu, S.-Y. Dai and Q.-H. Song, Org. Electron., 2014, 15, 2079 CrossRef CAS;
(b) S. P. Singh and T. Gayathri, Eur. J. Org. Chem., 2014, 4689 CrossRef CAS;
(c) M. Mao, X. Zhang, L. Cao, Y. Tong and G. Wu, Dyes Pigm., 2015, 117, 28 CrossRef CAS;
(d) Y.-D. Lin, B.-Y. Ke, Y. J. Chang, P.-T. Chou, K.-L. Liau, C.-Y. Liu and T. J. Chow, J. Mater. Chem. A, 2015, 3, 16831 RSC;
(e) I. Gonzalez-Valls, A. Mirloup, T. Le Bahers, N. Keller, T. Cottineau, P. Sautet and V. Keller, RSC Adv., 2016, 6, 91529 RSC;
(f) N. Kaneza, J. Zhang, H. Liu, P. S. Archana, Z. Shan, M. Vasiliu, S. H. Polansky, D. A. Dixon, R. E. Adams, R. H. Schmehl, A. Gupta and S. Pan, J. Phys. Chem. C, 2016, 120, 9068 CrossRef CAS;
(g) Z. Lu, M. Liang, P. Dai, K. Miao, C. Zhang, Z. Sun and S. Xue, J. Phys. Chem. C, 2016, 120, 25657 CrossRef CAS;
(h) J. Zhang, F. Lu, S. Qi, Y. Zhao, K. Wang, B. Zhang and Y. Feng, Dyes Pigm., 2016, 128, 296 CrossRef CAS;
(i) H. Cheema, R. Younts, B. Gautam, K. Gundogdu and A. El-Shafei, Mater. Chem. Phys., 2016, 184, 57 CrossRef CAS.
- A. Mirloup, P. Retailleau and R. Ziessel, Tetrahedron Lett., 2013, 54, 4456 CrossRef CAS.
- C. D. Fiandra, M. Moccia, V. Cerullia and M. F. A. Adamo, Chem. Commun., 2016, 52, 1697 RSC.
- Both the geometry optimization and energy calculation were performed by employing the density functional theory (DFT), at the level of B3LYP/6-31G(d,p) on the Gaussian09 program package (M. J. Frisch, G. W. Trucks, H. B. Schlegel, G. E. Scuseria, M. A. Robb, J. R. Cheeseman, G. Scalmani, V. Barone, B. Mennucci, G. A. Petersson, H. Nakatsuji, M. Caricato, X. Li, H. P. Hratchian, A. F. Izmaylov, J. Bloino, G. Zheng, J. L. Sonnenberg, M. Hada, M. Ehara, K. Toyota, R. Fukuda, J. Hasegawa, M. Ishida, T. Nakajima, Y. Honda, O. Kitao, H. Nakai, T. Vreven, J. A. Montgomery Jr, J. E. Peralta, F. Ogliaro, M. Bearpark, J. J. Heyd, E. Brothers, K. N. Kudin, V. N. Staroverov, R. Kobayashi, J. Normand, K. Raghavachari, A. Rendell, J. C. Burant, S. S. Iyengar, J. Tomasi, M. Cossi, N. Rega, J. M. Millam, M. Klene, J. E. Knox, J. B. Cross, V. Bakken, C. Adamo, J. Jaramillo, R. Gomperts, R. E. Stratmann, O. Yazyev, A. J. Austin, R. Cammi, C. Pomelli, J. W. Ochterski, R. L. Martin, K. Morokuma, V. G. Zakrzewski, G. A. Voth, P. Salvador, J. J. Dannenberg, S. Dapprich, A. D. Daniels, O. Farkas, J. B. Foresman, J. V. Ortiz, J. Cioslowski and D. J. Fox, Gaussian 09, Revision A.02, Gaussian, Inc., Wallingford CT, 2009) Search PubMed.
Footnote |
† Electronic supplementary information (ESI) available. See DOI: 10.1039/c7ra00799j |
|
This journal is © The Royal Society of Chemistry 2017 |
Click here to see how this site uses Cookies. View our privacy policy here.