DOI:
10.1039/C7RA00870H
(Paper)
RSC Adv., 2017,
7, 28616-28625
Triple zirconocene/brønsted acid/CuO cooperative and relay catalysis system for tandem Mannich addition/C–C formative cyclization/oxidation†
Received
20th January 2017
, Accepted 18th May 2017
First published on 31st May 2017
Abstract
A new triple cooperative and relay catalysis system featuring the Mannich addition followed by C–C construction and oxydehydrogenation is described. The zirconocene dichloride and trimellitic acid synergic catalysis triggered the Mannich addition and C–C bond construction reactions, while CuO allowed relay catalysis for oxydehydrogenation. This novel strategy demonstrated superior activity for the synthesis of substituted quinolines from commercially available anilines, aldehydes and ketones. The corresponding substituted quinolines were synthesized with 32 examples in 90–96% yields under mild reaction conditions. A novel zirconocene–Brønsted acid complex, generated in situ and acting as an active catalyst, was validated from the mechanistic studies.
Introduction
Relay catalysis reactions are the one-pot multi-catalysis cascade reactions in which each catalytic system promotes one type of transformation in a consecutive fashion. The strategy of relay catalysis obviates the requirement of isolation and purification of products resulting from the each independent transformation. More importantly, the advent of one-pot orthogonal relay catalysis reactions made intricate organic syntheses feasible, which were inaccessible and inefficient by the classical step-wise catalysis. However, establishing such processes need to overcome a number of challenging issues, such as the chemo selectivity and the compatibility between the catalytic systems. The combined metal/metal and metal/organo catalyst dual catalysis are potential kinds of relay catalysis that has stimulated intensive interest in recent years. A number of significant reactions have been developed using metal/metal relay catalysis including Au/Ni,1 Au/Ag,2 Au/Yb,3 Au/Sc,4 Ru/Pd/Cu5 and Pd/Ag/Bi6 catalyst systems. Regarding to the metal/organo relay catalysis, the metal-Brønsted acid based systems have contributed largely.7–12 The metal catalysts such as Au, Rh, Pd, Ag and Ru are used in combination with a variety of Brønsted acids. In this context, the development of divergent relay catalysis approaches employing new catalysts is highly desirable.
The cascade reactions for the Mannich reaction followed by the C–C or C–N bond formation are of highly appealing (Scheme 1). This is because such kind of reactions could construct these fundamental bonds of organic molecules in a single step. In literature, two divergent synthetic procedures with such cascade reaction were available. In 2010, Shin13 discovered an one pot redox-pinacol-Mannich–Michael cascade reaction leading to the synthesis of 1-aminoindanes and 5,6-fused azacycles (Scheme 1, eq. a). In this reaction, the gold catalyst rendered the Mannich reaction followed by C–N formation in an intramolecular manner. Very recently, HCl,14 iodine,15 CuCl2,16 FeCl3 (ref. 17) and AgOTf18 catalyzed three components tandem reactions of anilines, aldehydes and ketones for the construction of multi-functionalized quinoline had been reported (Scheme 1, eq. b). This approach undergone intermolecular Mannich reaction followed by intramolecular C–C bond formation and oxydehydrogenation.
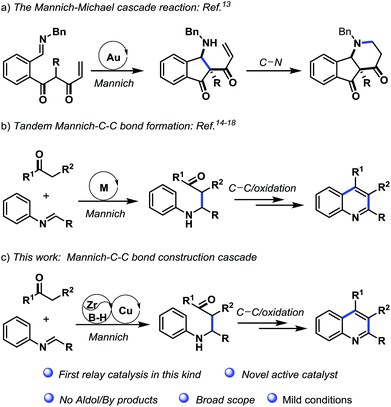 |
| Scheme 1 The reactions involving the Mannich reaction followed by C–C/C–N bond formation. | |
In this paper, combination with the advantages of cascade reaction integrating the Mannich addition and C–C/C–N bond construction, the development of new catalytic systems is highly desirable in organic synthesis. Herein, we report an unprecedented cooperative and relay catalysis by zirconocene dichloride, trimellitic acid and CuO triple catalytic system for the Mannich addition/C–C bond formation/oxydehydrogenation sequence (Scheme 1, eq. c). This triple cooperative and relay catalytic system demonstrated superior activity for synthesis of polysubstituted quinoline from arylamines, aldehydes and ketones under mild condition.
Results and discussion
We choose aniline, benzaldehyde and methyl pyruvate as substrates to optimize the conditions of cascade Mannich addition, C–C bond construction and oxydehydrogenation reaction (Table 1). At first, simple copper salts such as CuCl2, CuBr2, Cu(acac)2, Cu(OAc)2, CuSO4·5H2O, CuO, Cu(OTf)2 and Cu(ClO4)2 were used as catalysts in the presence of both Cp2TiCl2 and PhCOOH (Table 1, entries 1–8). Among these metal salts, CuO was found to be the most effective catalyst and the desired product 4aa was obtained in 31% yield (Table 1, entry 6). Other commercially available metallocene complexes such as Cp*TiCl3 and Cp2ZrCl2 were also used as Lewis acid catalysts for the three-component sequence reactions, which demonstrated that Cp2ZrCl2 was the best catalyst for this transformation (Table 1, entries 9 and 10). In light of our previous findings that salicylato–titanocene complexes and phenols–titanocene complexes function as organometallic Lewis acids,19 a selection of oxygen donor ligands such as phenol and benzenesulfonic acid were evaluated in the reaction of aniline, benzaldehyde and methyl pyruvate (Table 1, entries 11 and 12). None of them provide satisfactory results, which suggested that mono-functional acids were unsuitable for this three-component cascade reaction. With the assistance of o-aminobenzoic acid, salicylic acid and phthalic acid, the Zr catalyst was slightly activated and the tandem reaction afforded desired product with 41%, 42% and 47% yields, respectively (Table 1, entries 13–15). When glutaric acid and proline were introduced into this triple catalytic system, the desired products were obtained in 29% and 28% yields (Table 1, entries 16 and 17). The above results indicated that the aromatic diacids show up higher activity than the aliphatic diacids and amino acids. We hypothesized that higher activity of this catalytic system could be achieved by employing multifunctional acids such as 2-aminobenzene-1,4-disulfonic acid, trimellitic acid and 5-sulfosalicylic acid (Table 1, entries 18–20). To our pleased, trimellitic acid is the most potent catalyst among the three multifunctional aromatic acids, which promotes the reaction to afford the desired product in 54% yield, higher than 5-sulfosalicylic acid and 2-aminobenzene-1,4-disulfonic acid. When the reaction time extended to 2 h, the yield increases to 88%, but the yield increased slightly with further extension of the reaction time (Table 1, entry 21). After an extensive screening of the reaction parameters (see the ESI†), the best yield of 4aa was obtained when reaction was performed in i-propanol/water (3
:
1) at 60 °C.
Table 1 Catalyst and Brønsted acid screening for three-component sequence reactiona

|
Entry |
Catalyst |
Brønsted acid |
Yieldb (%) |
All reactions were conducted using the aniline (1.0 mmol), benzaldehyde (1.0 mmol), methyl pyruvate (1.0 mmol), catalyst (0.05 mmol, 5 mol%), Brønsted acid (0.05 mmol, 5 mol%), 50 °C, 1 h. Isolated yields. 50 °C, 2 h. |
1 |
Cp2TiCl2/CuCl2 |
PhCOOH |
30 |
2 |
Cp2TiCl2/CuBr2 |
PhCOOH |
28 |
3 |
Cp2TiCl2/Cu(acac)2 |
PhCOOH |
25 |
4 |
Cp2TiCl2/Cu(OAc)2 |
PhCOOH |
22 |
5 |
Cp2TiCl2/CuSO4·5H2O |
PhCOOH |
24 |
6 |
Cp2TiCl2/CuO |
PhCOOH |
31 |
7 |
Cp2TiCl2/Cu(OTf)2 |
PhCOOH |
26 |
8 |
Cp2TiCl2/Cu(ClO4)2 |
PhCOOH |
27 |
9 |
Cp*TiCl3/CuO |
PhCOOH |
29 |
10 |
Cp2ZrCl2/CuO |
PhCOOH |
34 |
11 |
Cp2ZrCl2/CuO |
PhOH |
20 |
12 |
Cp2ZrCl2/CuO |
PhSO3H |
26 |
13 |
Cp2ZrCl2/CuO |
Ph (COOH)NH2 |
41 |
14 |
Cp2ZrCl2/CuO |
Ph (COOH)OH |
42 |
15 |
Cp2ZrCl2/CuO |
Ph (COOH)2 |
47 |
16 |
Cp2ZrCl2/CuO |
(CH2)3(COOH)2 |
29 |
17 |
Cp2ZrCl2/CuO |
HNC4H7COOH |
28 |
18 |
Cp2ZrCl2/CuO |
H2NPh (SO3H)2 |
45 |
19 |
Cp2ZrCl2/CuO |
5-SO3HPhCOOH (OH) |
48 |
20 |
Cp2ZrCl2/CuO |
Ph (COOH)3 |
54 |
21 |
Cp2ZrCl2/CuO |
Ph (COOH)3 |
88c |
With the optimal reaction conditions in hand, we set out to expand the generality and scope of Cp2ZrCl2, trimellitic acid and CuO catalyzed three-component cascade reaction. 1 mmol aldehyde, 1.1 mmol of anilines and 1.5 mmol of ketones with 5 mol% of Cp2ZrCl2, 5 mol% of trimellitic acid, 5 mol% of CuO at 60 °C for 2 h were operated as the typical selection. It is found that the reaction proceeded smoothly with aldehydes and aromatic amines bearing either electron-withdrawing or donating groups (Table 2). The substituents with different electron properties has little impact on this transformation, such as anilines containing methyl (4ab), methoxyl (4ac) or fluorous (4ai) group, benzaldehydes with methyl (4ak), i-propyl (4al), t-butyl (4am) or methoxyl (4ad) group and pyruvate with methyl or ethyl group. Aniline with methyl (4ab) and methoxyl (4ac) substituents led to the excellent results (90–91%), when benzaldehyde and methyl pyruvate were used. The p-substituents of anilines were changed from methyl (4ae), i-propyl (4af), t-butyl (4ag) to methoxyl (4ah) reacted with 4-methoxybenzaldehyde and methyl pyruvate, the yields of the condensation reaction were 93–95%. Under the similar condition, treating 4-fluroaniline and benzaldehyde with methyl pyruvate afforded the substituted quinoline (4ai) in 90% yield. Benzaldehydes with methoxyl (4aj), methyl (4ak), i-propyl (4al) or t-butyl (4am) substituents obtained 92–94% yields when they reacted with 1-naphthylamine and methyl pyruvate, which provided a convenient route for the construction of tricyclic–quinolines. Aliphatic aldehydes such as cyclohexanecarbaldehyde was also readily introduced into this reaction, reacted with 1-naphthylamine or 4-methoxy aniline and methyl pyruvate afforded the desired products (4an, 4ao) in 91% and 90% yields, respectively. Heterocyclic furfuraldehyde afforded the desired product in 91% yield (4ap). 1-Naphthaldehyde reacted with aniline and methyl pyruvate or ethyl pyruvate under standard conditions afforded the multiply substituted quinolines in 92% and 91% yield (4aq and 4ar). As expected, m-substituted aniline and aldehyde (4as) produced desired quinoline in 92% yield. Gratifyingly, the sterically hindered ortho-substituted anilines (4at) still furnished the desired product in more than 90% yield.
Table 2 Zirconocene dichloride/trimellitic acid/copper oxide catalyzed three components tandem reaction of aldehydes, arylamines and pyruvatesa,b
The zirconocene dichloride/trimellitic acid/CuO cooperative and relay catalytic system was successfully applied in the three-component coupling sequence reaction of aliphatic ketones and aromatic ketones under the optimized conditions (Table 3). The reaction results had not significantly effected by aliphatic ketones. The catalytic sequence reaction of 2-butanone with benzaldehyde and 1-naphthylamine afforded the products (4bb) in 93% yields, whereas other chain ketones including 2-pentone, 2-hexanone and 2-heptanone afforded 2,4-substituted quinolines in 91–93% yield (4bd), (4be), (4bf). Cyclic ketones such as cyclohexanone (4bg) was also readily introduced into this reaction, the desired product being formed with yield of 96%. Various halogens, such as fluorine, chlorine, bromine, iodine and electron-donating groups such as methyl were all tolerated in this reaction, afforded the products with 91–94% yields (4bh, 4bi, 4bj, 4bk and 4be). This triple cooperative and relay catalytic system were also applied in the three-component cascade reaction of aromatic ketone, such as anilines, benzaldehyde reacted with acetophenone afforded 2,4-diphenylquinoline in 90% and 91% yield(4bl and 4bm), albeit with longer reaction time (12 h). To our pleased, chain aldehyde can also be used as substrate for this reaction afforded 6-methoxy-4-ethyl-2-propylquinoline in 90% yield with 12 h(4bn). More importantly, this cooperative and relay catalytic system for synthesis of substituted quinoline could be easily scaled up and the desired disubstituted quinoline was obtained in 92% yield (Scheme 2).
Table 3 Zirconocene dichloride/trimellitic acid/copper oxide catalyzed three components tandem reaction of aldehydes, arylamines and ketonesa,b
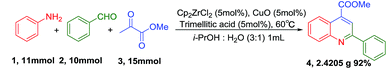 |
| Scheme 2 Scaled up for the gram scale. | |
To get more information about the reaction mechanism, several control experiments (Table 4) and parallel experiment (Scheme 3) were set up under the standard conditions. The three components cascade reaction of aniline, benzaldehyde and methyl pyruvate without catalyst or with 5 mol% CuO did not get the desired product at 60 °C for 2 h (Table 4, entries 1 and 2). In the presence of 5 mol% trimellitic acid, three-component sequence reaction afforded the desired quinoline in 14% yield (Table 4, entry 3). With addition of 5 mol% Cp2ZrCl2 in the reaction only obtained 17% yield of quinoline (Table 4, entry 4), which eliminated the possibility that i-propanol or water coordination with zirconocene dichloride released HCl promotes this reaction. With the assistance of 5 mol% Cp2ZrCl2 and 5 mol% trimellitic acid, the desired product was isolated in 44% yield (Table 4, entries 5). Adding 5 mol% CuO into this catalytic system, the yield of 4-methoxycarbonyl-2-phenylquinoline was up to 92% (Table 4, entry 6). It is clear that the high efficiency of the coupling sequence reaction should not be attributed to zirconocene dichloride, trimellitic acid or CuO individually. We proposed that the cooperation of zirconocene dichloride, trimellitic acid and the relay of CuO, resulted in the efficient cooperative and relay catalysis. The control experiments using 10 mol% HCl afforded the substituted quinolines in 10% yield, indicating HCl was not catalytic species (Scheme 3a). This control experiments and parallel experiment indicated that zirconocene dichloride and trimellitic acid demonstrated good compatibility with CuO in three components cascade reaction, which presented a new cooperative and relay catalysis system for synthesis of multiply substituted quinolines from anilines, aldehydes and ketones. N-Arylimines and methyl pyruvate under the standard condition also afforded the desired product in 92% yield (Scheme 3b), which indicated that the imine was firstly formed in this reaction, followed by Mannich addition and cyclization.
Table 4 Control experimenta
Entry |
CuO (mol%) |
Trimellitic acid (mol%) |
Cp2ZrCl2 (mol%) |
Yieldb (%) |
Reaction conditions: a mixture of aldehyde (1.0 mmol); aniline (1.1 mmol); methyl pyruvate (1.5 mmol); i-PrOH : H2O (3 : 1, 0.5 mL); all reactions were carried out at 60 °C for 2 h, ND = no detected. Isolated yield. |
1 |
— |
— |
— |
ND |
2 |
5 |
— |
— |
ND |
3 |
— |
5 |
— |
14 |
4 |
— |
— |
5 |
17 |
5 |
— |
5 |
5 |
44 |
6 |
5 |
5 |
5 |
92 |
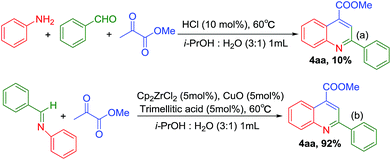 |
| Scheme 3 The paralleled experiment. | |
The interaction of zirconocene dichloride and trimellitic acid in three-component coupling sequence reaction for synthesis of substituted quinolines were investigated by 1H NMR and HRMS analyses. 1H NMR experiments, which were conducted using Cp2ZrCl2 in D2O (Fig. 1), showed that no coordination occurred and only one Cp singlet of Cp2ZrCl2 at δ = 6.49 ppm (
) was detected. When adding 1 equiv. trimellitic acid, a new zirconocene complex species II formed, which resonated at δ = 6.57 ppm (
). Intensity of the Cp singlet at δ = 6.57 didn't increase as the time went on. Adding 2 equiv. aniline into this transformation, zirconocene dichloride (I) was consumed gradually in D2O and transformed to a new zirconocene species Cp2Zr(OOC)2PhCOOH (II).20 The above conclusion was also certified by HRMS analysis, corresponding to the [II + H]+ signal at m/z 428.9921 (Fig. S3–S6†). These observations clearly demonstrated that during the reaction, zirconocene dichloride readily converted into zirconocene complexes II, and presumably it was the organometallic binary acid catalyst.
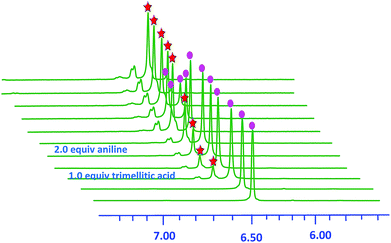 |
| Fig. 1 Partial 400 MHz 1H NMR spectra (D2O) of a mixture of Cp2ZrCl2 (1.0 equiv.) and trimellitic acid (1.0 equiv.) with PhNH2 (2.0 equiv). 6.49 ppm I [Cp2ZrCl2]; 6.57 ppm II Cp2Zr(OOC)2PhCOOH. | |
Taken 1HNMR, HRMS, control experiments and paralleled experiments together, a plausible catalytic cycle of zirconocene dichloride/trimellitic acid/CuO triple cooperative and relay catalytic system in three-component sequence reaction was illustrated in Scheme 4. Zirconocene dichloride I pre-catalyst activated by trimellitic acid and transformed to binary acid catalyst II in the presence of aniline. The incoming ketone coordinated to Zr center of II. In transition state A, enolation was accelerated as the carbonyl coordinated to oxytropic Zr and methyl formed hydrogen bond with the carboxyl oxygen of trimellitic acid. The carbon–carbon bond formation is illustrated in transition state A. The coordinated enolate then undergoes addition to the aldimine, which is activated by H+ from the other carboxyl group of trimellitic acid. This transition state show cases the cooperative nature of this binary acid catalytic system. In transition state B, the electron-rich benzene ring attacked the keto-carbonyl group to formed the intermediate dihydroquinoline. After the dihydroquinoline was released, the zirconocene catalyst II was regenerated from the transition state B by coordination of the carboxyl group again. The coordination of the dihydroquinoline and CuO may induce a combination of electron transfer and intramolecular rearrangement to give the desired product21 and Cu(0)·H2O, which should be more stable than Cu(0) due to its coordination with H2O. Active Cu(0)·H2O is facilely oxidized by oxygen in the air to restore CuO, which enters next catalytic cycle.
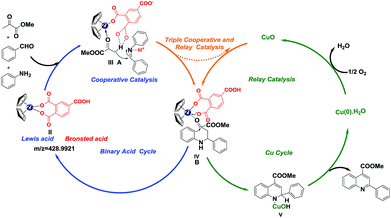 |
| Scheme 4 Proposed mechanism of zirconocene dichloride/trimellitic acid/CuO cooperative and relay catalysis for synthesis of substituted quinolines. | |
Conclusions
In summary, we have developed a triple cooperative and relay catalysis system that the combination of organometallic Lewis acids and Brønsted acids with metal were developed for three-component cascade Mannich addition/C–C formative cyclization/oxidation reaction. The green syntheses of substituted quinolines were achieved from readily available anilines, aldehydes and aromatic or aliphatic ketones in good to excellent yields. 1H NMR and HRMS analysis unveiled that Cp2Zr(OOC)2PhCOOH was catalytic specis, which significantly accelerated the condensation of aldehydes, anilines and ketones into dihydroquinolines with i-propanol and water as solvent. Meanwhile, CuO catalyzed oxydehydrogenation of dihydroquinolines to generate quinolines.
Experimental
General methods and materials
All manipulations were performed in an atmosphere of air. All reagents were purchased from the commercial approaches and used without further purification unless specified otherwise. 1H and 13C NMR spectra were recorded on a Bruker EQUINX55 (400 MHz for 1H; 101 MHz for 13C) spectrometer in CDCl3. For 1H NMR, tetramethylsilane (TMS) served as internal standard (δ = 0) and 1H NMR chemical shifts are reported in ppm downfield of tetramethylsilane and referenced to residual solvent peak (CDCl3 at 7.26 ppm) unless otherwise noted. The data are reported as follows: chemical shift, integration, multiplicity (s = singlet, d = doublet, t = triplet, q = quartet and m = multiplet), and coupling constant in Hz. For 13C NMR, CDCl3 was used as internal standard (δ = 77.0) and spectra were obtained with complete proton decoupling. HRMS (ESI) analysis was performed and (HRMS) data were reported with sodium mass/charge (m/z) ratios as values in atomic mass units. Column chromatography was performed on silica gel (230–400 mesh) and analytical thin layer chromatography was carried out using 250 μm commercial silica gel plates. Visualization of the developed chromatogram was performed by UV absorbance and stained with an iodine vapor.
Typical procedures for synthesis of substituted quinolones
A 10 mL test tube equipped with a magnetic stirrer and a septum, was charged with aldehyde (1.0 mmol), amine (1.1 mmol), and the ketone (1.5 mmol) in one portion. Cp2ZrCl2 (0.05 mmol, 5 mol%), CuO (0.05 mmol, 5 mol%) and trimellitic acid (0.05 mmol, 5 mol%) were stirred in i-PrOH
:
H2O (3
:
1) 0.5 mL. The reaction mixture was heated to 60 °C and stirred until the reaction was completed as indicated by TLC, then the reaction mixture was quenched with distilled water (5.0 mL). The aqueous phase was extracted with dichloride methane (3–5 mL), dried over Na2SO4 and concentrated in vacuo to give the crude product. The crude product was purified by flash column chromatography on neutral silica gel (ethyl acetate:petroleum ether). Full experimental details and characterization data for all quinolines product are included in the ESI.†
4-Methoxycarbonyl-2-phenylquinoline (4aa). Yield, 92%; primrose yellow solid; mp 217–218 °C; Rf 0.51 (petroleum ether/ethyl acetate = 10
:
1); IR (KBr): 1728, 1593, 1346, 1250, 1203, 1149, 771, 694 cm−1; 1H NMR (400 MHz, CDCl3) δ 8.75 (d, J = 8.5 Hz, 1H), 8.41 (s, 1H), 8.22 (s, 3H), 7.77 (s, 1H), 7.63 (s, 1H), 7.55 (s, 2H), 7.50 (d, J = 7.1 Hz, 1H), 4.07 (s, 3H). 13C NMR (101 MHz, CDCl3) δ 166.85, 156.71, 149.29, 138.81, 135.61, 130.35, 129.91, 129.74, 128.94, 127.81, 127.48, 125.43, 124.00, 120.34, 52.72. HRMS (ESI): m/z called for C17H13NO2 [M + H]+, 264.1019; found, 264.1026.
6-Methyl-4-methoxycarbonyl-2-phenylquinoline (4ab). Yield, 93%; primrose yellow solid; mp 212–213 °C; Rf 0.45 (petroleum ether/ethyl acetate = 10
:
1); IR (KBr): 1730, 1608, 1352, 1254, 1209, 1153, 775, 698 cm−1; 1H NMR (400 MHz, CDCl3) δ 8.51 (s, 1H), 8.35 (s, 1H), 8.19 (d, J = 7.3 Hz, 2H), 8.12 (d, J = 8.6 Hz, 1H), 7.61–7.57 (m, 1H), 7.54 (s, 2H), 7.47 (s, 1H), 4.06 (s, 3H), 2.58 (s, 3H). 13C NMR (101 MHz, CDCl3) δ 166.97, 155.72, 147.94, 138.90, 138.01, 134.81, 132.18, 129.99, 129.53, 128.90, 127.36, 124.24, 124.02, 120.23, 52.65, 22.13. HRMS (ESI): m/z called for C18H15NO2 [M + H]+, 278.1176; found, 278.1177.
6-Methoxy-4-methoxycarbonyl-2-phenylquinoline (4ac). Yield, 95%; primrose yellow solid; mp 215–219 °C; Rf 0.47(petroleum ether/ethyl acetate = 10
:
1); IR (KBr): 1726, 1591, 1344, 1253, 1206, 1152, 774, 697 cm−1; 1H NMR (400 MHz, CDCl3) δ 8.40 (s, 1H), 8.21 (d, J = 2.7 Hz, 1H), 8.16 (d, J = 7.5 Hz, 2H), 8.10 (d, J = 9.2 Hz, 1H), 7.52 (s, 2H), 7.42 (d, J = 2.6 Hz, 2H), 4.04 (s, 3H), 3.97 (s, 3H). 13C NMR (101 MHz, CDCl3) δ 166.91, 159.05, 154.03, 145.70, 138.92, 133.17, 131.72, 129.29, 128.88, 127.14, 125.55, 122.79, 120.69, 103.22, 55.57, 52.56. HRMS (ESI): m/z called for C17H13NO2 [M + H]+, 294.1130; found, 294.1125.
4-Methoxycarbonyl-2-[4-methoxyl]phenylquinoline (4ad). Yield, 92%; primrose yellow solid; mp 220–221 °C; Rf 0.49 (petroleum ether/ethyl acetate = 10
:
1); IR (KBr): 1731, 1596, 1349, 1253, 1206, 1152, 778, 698 cm−1; 1H NMR (400 MHz, CDCl3) δ 8.71 (d, J = 8.3 Hz, 1H), 8.36 (s, 1H), 8.18 (d, J = 8.9 Hz, 3H), 7.75 (d, J = 1.2 Hz, 1H), 7.59 (s, 1H), 7.06 (d, J = 8.8 Hz, 2H), 4.07 (s, 3H), 3.89 (s, 3H). 13C NMR (101 MHz, CDCl3) δ 166.96, 161.15, 156.27, 149.28, 135.50, 131.38, 130.10, 129.83, 128.85, 127.39, 125.40, 123.67, 119.93, 114.33, 55.42, 52.70. HRMS (ESI): m/z called for C18H15NO3 [M + H]+, 294.1125; found, 294.1130.
6-Methyl-4-methoxycarbonyl-2-[4-methoxyl]phenylquinoline (4ae). Yield, 93%; yellow solid; mp 224–225 °C; Rf 0.52 (petroleum ether/ethyl acetate = 10
:
1); IR (KBr): 1729, 1596, 1348, 1254, 1207, 1151, 773, 696 cm−1; 1H NMR (400 MHz, CDCl3) δ 8.48 (s, 1H), 8.32 (s, 1H), 8.16 (d, J = 8.8 Hz, 2H), 8.08 (d, J = 8.6 Hz, 1H), 7.59 (dd, J = 8.6, 1.6 Hz, 1H), 7.26 (s, 2H), 7.05 (d, J = 8.8 Hz, 2H), 4.07 (s, 3H), 3.90 (s, 3H), 2.58 (s, 3H). 13C NMR (101 MHz, CDCl3) δ 190.81, 167.07, 160.97, 155.31, 147.91, 137.53, 134.73, 132.07, 131.98, 131.48, 129.76, 128.69, 124.22, 123.67, 119.81, 114.31, 114.28, 55.39, 52.62, 22.08. HRMS (ESI): m/z called for C19H17NO3 [M + H]+, 308.1281; found, 308.1289.
6-i-Propyl-4-methoxycarbonyl-2-[4-methoxyl]phenylquinoline (4af). Yield, 93%; yellow solid; mp 210–211 °C; Rf 0.51 (petroleum ether/ethyl acetate = 10
:
1); IR (KBr): 1732, 1595, 1349, 1251, 1205, 1152, 774, 698 cm−1; 1H NMR (400 MHz, CDCl3) δ 8.54 (d, J = 1.4 Hz, 1H), 8.33 (s, 1H), 8.15 (s, 3H), 7.66 (d, J = 1.8 Hz, 1H), 7.05 (d, J = 8.8 Hz, 2H), 4.07 (s, 3H), 3.89 (s, 3H), 3.14 (d, J = 13.6, 6.8 Hz, 1H), 1.37 (d, J = 6.9 Hz, 6H). 13C NMR (101 MHz, CDCl3) δ 167.11, 160.97, 155.45, 148.29, 148.21, 134.92, 131.60, 130.00, 129.50, 128.72, 123.75, 121.70, 119.84, 114.29, 55.41, 52.60, 34.56, 23.88. HRMS (ESI): m/z called for C21H21NO3 [M + H]+, 336.1594; found, 336.1589.
6-t-Butyl-4-methoxycarbonyl-2-[4-methoxyl]phenylquinoline (4ag). Yield, 95%; yellow solid; mp 207–208 °C; Rf 0.50 (petroleum ether/ethyl acetate = 10
:
1); IR (KBr): 1733, 1596, 1349, 1252, 1205, 1143, 774, 698 cm−1; 1H NMR (400 MHz, CDCl3) δ 8.72 (d, J = 2.0 Hz, 1H), 8.34 (s, 1H), 8.15 (dd, J = 15.5, 8.8 Hz, 3H), 7.85 (dd, J = 8.9, 2.1 Hz, 1H), 7.05 (d, J = 8.8 Hz, 2H), 4.08 (s, 3H), 3.89 (s, 3H), 1.46 (s, 9H). 13C NMR (101 MHz, CDCl3) δ 167.12, 160.97, 155.62, 150.36, 147.90, 135.08, 131.62, 129.59, 128.73, 123.44, 120.40, 119.88, 114.29, 55.41, 52.61, 35.29, 31.22. HRMS (ESI): m/z called for C21H21NO3 [M + H]+, 350.1751; found, 350.1756.
6-Methoxy-4-methoxycarbonyl-2-[4-methoxyl]phenylquinoline (4ah). Yield, 95%; yellow solid; mp 210–211 °C; Rf 0.48 (petroleum ether/ethyl acetate = 10
:
1); IR (KBr): 1725, 1591, 1343, 1247, 1201, 1146, 767, 691 cm−1; 1H NMR (400 MHz, CDCl3) δ 8.38 (s, 1H), 8.20 (d, J = 2.3 Hz, 1H), 8.14 (d, J = 8.6 Hz, 2H), 8.08 (d, J = 9.2 Hz, 1H), 7.41 (d, J = 9.2 Hz, 1H), 7.05 (d, J = 8.6 Hz, 2H), 4.06 (s, 3H), 3.98 (s, 3H), 3.89 (s, 3H). 13C NMR (101 MHz, CDCl3) δ 167.06, 160.79, 158.78, 153.78, 145.70, 133.23, 131.59, 131.52, 128.47, 125.15, 122.65, 120.32, 114.28, 103.32, 55.57, 55.40, 52.55. HRMS (ESI): m/z called for C19H17NO4 [M + H]+, 324.1230; found, 324.1234.
6-Fluoro-4-methoxycarbonyl-2-[4-methoxyl]phenylquinoline (4ai). Yield, 90%; yellow solid; mp 230–231 °C; Rf 0.46 (petroleum ether/ethyl acetate = 10
:
1); IR (KBr): 1723, 1585, 1342, 1245, 1200, 1144, 767, 691 cm−1; 1H NMR (400 MHz, CDCl3) δ 8.87 (d, J = 8.5 Hz, 1H), 8.57 (s, 1H), 8.43 (s, 3H), 7.92 (s, 1H), 7.89 (s, 1H), 7.74 (s, 2H), 7.70 (d, J = 7.1 Hz, 1H), 4.11 (s, 3H). 13C NMR (101 MHz, CDCl3) δ 176.91, 164.83, 158.21, 148.78, 144.31, 140.25, 138.90, 138.60, 137.94, 138.83, 138.45, 134.39, 133.05, 129.33, 51.65. HRMS (ESI): m/z called for C17H12FNO2 [M + H]+, 282.1019; found, 282.1012.
4-Methoxycarbonyl-2-[4-methoxylphenyl]benzoquinoline (4aj). Yield, 94%; yellow solid; mp 213–214 °C; Rf 0.49 (petroleum ether/ethyl acetate = 10
:
1); IR (KBr): 1733, 1597, 1351, 1255, 1209, 1154, 778, 697 cm−1; 1H NMR (400 MHz, CDCl3) δ 9.48 (d, J = 7.8 Hz, 1H), 8.59 (d, J = 9.2 Hz, 1H), 8.42 (s, 1H), 8.33 (d, J = 8.6 Hz, 2H), 7.90 (d, J = 7.5 Hz, 1H), 7.85 (d, J = 9.2 Hz, 1H), 7.73 (s, 2H), 7.09 (d, J = 8.6 Hz, 2H), 4.09 (s, 3H), 3.91 (s, 3H). 13C NMR (101 MHz, CDCl3) δ 167.19, 161.03, 154.45, 147.28, 135.39, 133.48, 131.59, 131.52, 128.69, 128.52, 127.59, 126.97, 125.14, 122.37, 122.07, 119.02, 114.25, 55.40, 52.70. HRMS (ESI): m/z called for C22H17NO3 [M + H]+, 344.1281; found, 344.1286.
4-Methoxycarbonyl-2-[4-methylphenyl]benzoquinoline (4ak). Yield, 93%; yellow solid; mp 225–226 °C; Rf 0.53 (petroleum ether/ethyl acetate = 10
:
1); IR (KBr): 1736, 1598, 1352, 1254, 1208, 1155, 778, 697 cm−1; 1H NMR (400 MHz, CDCl3) δ 9.50 (d, J = 7.8 Hz, 1H), 8.60 (d, J = 9.2 Hz, 1H), 8.45 (s, 1H), 8.27 (d, J = 7.8 Hz, 2H), 7.86 (d, J = 9.2 Hz, 2H), 7.74 (s, 2H), 7.38 (d, J = 7.7 Hz, 2H), 4.09 (s, 3H), 2.47 (s, 3H). 13C NMR (101 MHz, CDCl3) δ 167.22, 154.89, 147.37, 139.75, 136.16, 135.51, 133.48, 131.66, 129.65, 128.80, 128.54, 127.61, 127.25, 127.06, 125.19, 122.36, 119.38, 52.73, 21.40. HRMS (ESI): m/z called for C22H17NO3 [M + H]+, 328.1332; found, 328.1342.
4-Methoxycarbonyl-2-[4-i-propylphenyl]benzoquinoline (4al). Yield, 92%; yellow solid; mp 229–230 °C; Rf 0.52 (petroleum ether/ethyl acetate = 10
:
1); IR (KBr): 1721, 1583, 1340, 1244, 1197, 1141, 762, 683 cm−1; 1H NMR (400 MHz, CDCl3) δ 9.50 (d, J = 8.0 Hz, 1H), 8.61 (d, J = 9.2 Hz, 1H), 8.46 (s, 1H), 8.30 (d, J = 8.2 Hz, 2H), 7.91–7.83 (m, 2H), 7.77–7.68 (m, 2H), 7.45 (d, J = 8.2 Hz, 2H), 4.08 (s, 3H), 3.04 (dt, J = 13.8, 6.9 Hz, 1H), 1.36 (d, J = 7.0 Hz, 6H). 13C NMR (101 MHz, CDCl3) δ 167.20, 154.99, 150.66, 147.39, 136.64, 135.47, 133.48, 131.68, 128.80, 128.53, 127.60, 127.43, 127.04, 125.22, 122.39, 122.37, 119.50, 52.71, 34.07, 23.97. HRMS (ESI): m/z called for C24H21NO3 [M + H]+, 356.1645; found, 356.1650.
4-Methoxycarbonyl-2-[4-t-butylphenyl]benzoquinoline (4am). Yield, 94%; primrose yellow solid; mp 215–216 °C; Rf 0.48 (petroleum ether/ethyl acetate = 10
:
1); IR (KBr): 1721, 1585, 1342, 1245, 1294, 1140, 765, 685 cm−1; 1H NMR (400 MHz, CDCl3) δ 9.51 (d, J = 7.9 Hz, 1H), 8.61 (d, J = 9.2 Hz, 1H), 8.47 (s, 1H), 8.30 (d, J = 8.4 Hz, 2H), 7.88 (s, 2H), 7.78–7.71 (m, 2H), 7.62 (d, J = 8.4 Hz, 2H), 4.09 (s, 3H), 1.43 (s, 9H). 13C NMR (101 MHz, CDCl3) δ 167.22, 154.97, 152.90, 147.42, 136.24, 135.51, 133.49, 131.69, 128.82, 128.54, 127.60, 127.16, 125.90, 125.22, 122.40, 122.37, 119.54, 52.73, 34.82, 31.33. HRMS (ESI): m/z called for C25H23NO3 [M + H]+, 370.1802; found, 370.1805.
4-Methoxycarbonyl-2-cyclohexylbenzoquinoline (4an). Yield, 91%; primrose yellow solid; mp 213–214 °C; Rf 0.47 (petroleum ether/ethyl acetate = 10
:
1); IR (KBr): 1729, 1595, 1345, 1253, 1204, 1151, 774, 698 cm−1; 1H NMR (400 MHz, CDCl3) δ 9.42 (d, J = 7.7 Hz, 1H), 8.57 (d, J = 9.2 Hz, 1H), 7.89 (s, 2H), 7.85 (d, J = 9.3 Hz, 1H), 7.71 (d, J = 1.7 Hz, 2H), 4.06 (s, 3H), 3.04 (s, 1H), 2.14 (d, J = 11.9 Hz, 2H), 1.96 (d, J = 12.9 Hz, 2H), 1.80 (d, J = 11.7 Hz, 2H), 1.53 (d, J = 12.8 Hz, 4H). 13C NMR (101 MHz, CDCl3) δ 167.41, 164.84, 135.05, 133.30, 131.54, 128.28, 127.48, 126.90, 125.07, 122.40, 120.96, 52.60, 46.99, 32.91, 26.58, 26.18. HRMS (ESI): m/z called for C21H21NO3 [M + H]+, 320.1645; found, 320.1648.
6-Methoxy-4-methoxycarbonyl-2-cyclohexylquinoline (4ao). Yield, 90%; primrose yellow solid; mp 203–204 °C; Rf 0.50 (petroleum ether/ethyl acetate = 10
:
1); IR (KBr): 1733, 1598, 1349, 1253, 1206, 1152, 775, 697 cm−1; 1H NMR (400 MHz, CDCl3) δ 8.17 (d, J = 2.7 Hz, 1H), 7.99 (d, J = 9.2 Hz, 1H), 7.85 (s, 1H), 7.37 (dd, J = 9.2, 2.8 Hz, 1H), 4.03 (s, 3H), 3.96 (s, 3H), 2.01 (s, 1H), 1.77–1.59 (m, 4H), 1.33–1.24 (m, 4H), 1.01–0.89 (m, 2H). 13C NMR (101 MHz, CDCl3) δ 167.13, 163.49, 158.51, 145.18, 133.01, 130.96, 130.88, 125.14, 122.22, 121.43, 103.32, 55.53, 52.43, 47.08, 32.81, 26.51, 26.04. HRMS (ESI): m/z called for C18H21NO3 [M + Na]+, 322.1414; found, 322.1421.
6-Methoxy-4-methoxycarbonyl-2-furylquinoline (4ap). Yield, 91%; claybank; mp 210–211 °C; Rf 0.51 (petroleum ether/ethyl acetate = 10
:
1); IR (KBr): 1720, 1583, 1340, 1243, 1194, 1142, 764, 683 cm−1; 1H NMR (400 MHz, CDCl3) δ 8.35 (s, 1H), 8.20 (d, J = 2.6 Hz, 1H), 8.06 (d, J = 9.3 Hz, 1H), 7.62 (s, 1H), 7.40 (dd, J = 9.2, 2.6 Hz, 1H), 7.19 (d, J = 3.3 Hz, 1H), 6.98 (s, 1H), 4.05 (s, 3H), 3.97 (s, 3H). 13C NMR (101 MHz, CDCl3) δ 169.16, 168.26, 166.72, 159.03, 153.30, 146.21, 145.46, 143.92, 133.20, 131.35, 125.50, 122.95, 119.44, 112.27, 109.55, 103.47, 55.59, 52.60. HRMS (ESI): m/z called for C16H13NO4 [M + H]+, 284.0917; found, 284.0922.
4-Methoxycarbonyl-2-naphthylquinoline (4aq). Yield, 92%; primrose yellow solid; mp 233–234 °C; Rf 0.47 (petroleum ether/ethyl acetate = 10
:
1); IR (KBr): 1717, 1585, 1340, 1242, 1195, 1141, 763, 685 cm−1; 1H NMR (400 MHz, CDCl3) δ 8.88 (d, J = 8.5 Hz, 1H), 8.32 (d, J = 8.4 Hz, 1H), 8.27 (s, 1H), 8.16 (d, J = 8.1 Hz, 1H), 8.02–7.96 (m, 2H), 7.85 (t, J = 7.6 Hz, 1H), 7.79–7.71 (m, 2H), 7.64 (t, J = 7.7 Hz, 1H), 7.54 (dt, J = 13.4, 6.6 Hz, 2H), 4.07 (s, 3H). 13C NMR (101 MHz, CDCl3) δ 166.73, 158.97, 149.15, 137.87, 135.19, 134.05, 131.14, 130.33, 130.07, 129.55, 128.52, 127.99, 126.87, 126.13, 125.53, 124.50, 123.87, 52.76. HRMS (ESI): m/z called for C21H15NO3 [M + H]+, 314.1176; found, 314.1180.
4-Ethoxycarbonyl-2-naphthylquinoline (4ar). Yield, 91%; yellow solid; mp 235–236 °C; Rf 0.46 (petroleum ether/ethyl acetate = 10
:
1); IR (KBr): 1726, 1589, 1343, 1247, 1201, 1145, 763, 688 cm−1; 1H NMR (400 MHz, CDCl3) δ 8.85 (d, J = 8.6 Hz, 1H), 8.29 (d, J = 8.3 Hz, 1H), 8.23 (s, 1H), 8.13 (d, J = 8.2 Hz, 1H), 8.01–7.94 (m, 2H), 7.83 (s, 1H), 7.75 (d, J = 0.9 Hz, 2H), 7.65–7.60 (m, 1H), 7.53 (d, J = 1.8 Hz, 2H), 4.54 (s, 2H), 1.46 (s, 3H). 13C NMR (101 MHz, CDCl3) δ 166.34, 158.98, 149.12, 137.94, 135.69, 134.04, 131.17, 130.32, 130.02, 129.51, 128.50, 127.94, 126.85, 126.13, 125.39, 124.30, 123.90, 61.93, 14.31. HRMS (ESI): m/z called for C21H21NO3 [M + H]+, 328.1332; found, 328.1335.
7-Methoxy-4-methoxycarbonyl-2-phenylquinoline (4as). Yield, 92%; primrose yellow solid; mp 215–216 °C; Rf 0.52 (petroleum ether/ethyl acetate = 10
:
1); IR (KBr): 1726, 1589, 1345, 1248, 1186, 1146, 769, 682 cm−1; 1H NMR (400 MHz, CDCl3) δ 8.67 (d, J = 9.3 Hz, 1H), 8.27 (s, 1H), 8.21–8.17 (m, 2H), 7.55 (s, 3H), 7.52–7.47 (m, 1H), 7.30 (d, J = 2.7 Hz, 1H), 4.07 (s, 3H), 4.00 (s, 3H). 13C NMR (101 MHz, CDCl3) δ 166.95, 160.85, 157.16, 151.27, 139.01, 135.33, 129.61, 128.90, 127.44, 126.52, 120.95, 119.26, 118.10, 108.10, 55.55, 52.67. HRMS (ESI): m/z called for C18H15NO3 [M + H]+, 294.1125; found, 294.1133.
8-Methoxy-4-methoxycarbonyl-2-phenylquinoline (4at). Yield, 90%; primrose yellow solid; mp 217–218 °C; Rf 0.49 (petroleum ether/ethyl acetate = 10
:
1); IR (KBr): 1724, 1588, 1342, 1247, 1187, 1141, 765, 684 cm−1; 1H NMR (400 MHz, CDCl3) δ 8.37 (s, 1H), 8.25 (d, J = 8.5 Hz, 1H), 8.20 (d, J = 8.3 Hz, 2H), 7.51 (s, 1H), 7.10 (d, J = 7.6 Hz, 1H), 7.04 (d, J = 8.3 Hz, 2H), 4.11 (s, 3H), 4.06 (s, 3H), 3.89 (s, 3H). 13C NMR (101 MHz, CDCl3) δ 167.08, 161.07, 155.59, 155.10, 141.29, 135.71, 131.58, 128.94, 127.54, 124.77, 120.21, 117.13, 114.27, 108.38, 56.25, 55.39, 52.69. HRMS (ESI): m/z called for C19H17NO4 [M + H]+, 323.1154; found, 323.1158.
4-Ethoxyl-2-[4-methylphenyl]benzoquinoline (4bb). Yield, 93%; primrose yellow solid; mp 221–225 °C; Rf 0.43 (petroleum ether/ethyl acetate = 10
:
1); IR (KBr): 3078, 2962, 2931, 1611, 1510, 1459 cm−1; 1H NMR (400 MHz, CDCl3) δ 9.52 (d, J = 8.0 Hz, 1H), 8.32 (d, J = 8.8 Hz, 2H), 7.91 (d, J = 4.9 Hz, 2H), 7.81 (d, J = 7.4 Hz, 4H), 7.09 (d, J = 8.8 Hz, 2H), 3.91 (s, 3H), 3.18 (s, 2H), 1.46 (s, 3H). 13C NMR (101 MHz, CDCl3) δ 160.67, 154.90, 150.16, 146.21, 133.54, 132.75, 132.34, 128.72, 127.84, 127.53, 126.65, 125.17, 123.52, 120.95, 117.52, 114.16, 55.41, 25.84, 14.64. HRMS (ESI): m/z called for C21H21NO3 [M + H]+, 336.1594; found, 336.1589. HRMS (ESI): m/z called for C22H19NO [M + H]+, 314.1539; found, 314.1542.
4-Propyl-2-[4-methylphenyl]benzoquinoline (4bc). Yield, 94%; white solid; mp 229–221 °C; Rf 0.45 (petroleum ether/ethyl acetate = 10
:
1); IR (KBr): 3076, 2957, 2929, 1597, 1498, 1456 cm−1; 1H NMR (400 MHz, CDCl3) δ 9.55 (d, J = 8.1 Hz, 1H), 8.33 (d, J = 8.8 Hz, 2H), 7.90 (s, 2H), 7.79 (s, 4H), 7.10 (d, J = 8.8 Hz, 2H), 3.91 (s, 3H), 3.11 (s, 2H), 1.87 (d, J = 7.6 Hz, 2H), 1.09 (s, 3H). 13C NMR (101 MHz, CDCl3) δ 160.68, 154.66, 148.71, 133.55, 132.72, 132.35, 128.73, 127.86, 127.55, 126.66, 125.18, 123.74, 121.16, 118.48, 114.16, 55.41, 34.90, 23.71, 14.20. HRMS (ESI): m/z called for C23H21NO [M + H]+, 328.1696; found, 328.1702.
6-Methyl-4-butyl-2-phenylquinoline (4bd). Yield, 91%; white solid; mp 222–223 °C; Rf 0.47 (petroleum ether/ethyl acetate = 10
:
1); IR (KBr): 3071, 2954, 2923, 1596, 1491, 1451 cm−1; 1H NMR (400 MHz, CDCl3) δ 7.94 (s, 2H), 7.46 (s, 2H), 7.14 (d, J = 34.7 Hz, 4H), 5.92 (s, 1H), 2.38 (s, 5H), 1.55 (s, 2H), 1.33 (s, 2H), 0.87 (s, 3H). 13C NMR (101 MHz, CDCl3) δ 188.57, 167.29, 140.31, 135.88, 135.77, 130.67, 129.71, 128.19, 126.99, 125.33, 92.55, 31.95, 30.40, 22.36, 20.94, 13.67. HRMS (ESI): m/z called for C20H21N [M + H]+, 276.1713; found, 276.1716.
5,7-Dimethyl-4-butyl-2-phenylquinoline (4be). Yield, 93%; white solid; mp 219–220 °C; Rf 0.46 (petroleum ether/ethyl acetate = 10
:
1); IR (KBr): 3078, 2961, 2929, 1563, 1498, 1457 cm−1; 1H NMR (400 MHz, CDCl3) δ 7.99 (s, 2H), 7.47 (d, J = 6.1 Hz, 2H), 6.91 (s, 1H), 6.85 (s, 2H), 5.95 (s, 1H), 2.47 (s, 2H), 2.36 (s, 6H), 1.60 (s, 2H), 1.38 (s, 2H), 0.91 (s, 3H). 13C NMR (101 MHz, CDCl3) δ 188.50, 167.03, 140.37, 138.81, 138.31, 130.69, 128.21, 127.64, 127.03, 122.89, 92.70, 31.97, 30.47, 22.38, 21.24, 13.69. HRMS (ESI): m/z called for C21H23N [M + H]+, 290.1817; found, 290.1819.
6-Methyl-4-pentyl-2-phenylquinoline (4bf). Yield, 91%; white solid; mp 215–216 °C; Rf 0.47 (petroleum ether/ethyl acetate = 10
:
1); IR (KBr): 3075, 2959, 2928, 1599, 1495, 1453 cm−1; 1H NMR (400 MHz, CDCl3) δ 7.95 (d, J = 6.5 Hz, 2H), 7.47 (s, 2H), 7.20 (d, J = 7.8 Hz, 2H), 7.09 (d, J = 7.8 Hz, 2H), 5.92 (s, 1H), 2.39 (s, 5H), 1.56 (s, 2H), 1.28 (s, 4H), 0.86 (s, 3H). 13C NMR (101 MHz, CDCl3) δ 188.57, 167.32, 140.32, 135.89, 135.78, 130.67, 129.72, 128.19, 126.99, 125.33, 92.54, 32.21, 31.39, 27.93, 22.19, 20.94, 13.81. HRMS (ESI): m/z called for C21H23N [M + H]+, 290.1817; found, 290.1819.
6-(4-Methoxyphenyl)-7,8,9,10-tetrahydrobenzophenanthridine (4bg). Yield, 96%; white solid; mp 182–183 °C; Rf 0.48 (petroleum ether/ethyl acetate = 10
:
1); IR (KBr): 3078, 2962, 2931, 1591, 1484, 1447 cm−1; 1H NMR (400 MHz, CDCl3) δ 9.37 (d, J = 7.4 Hz, 1H), 7.89 (s, 2H), 7.82 (s, 1H), 7.71–7.63 (m, 4H), 7.05 (d, J = 8.6 Hz, 2H), 3.91 (s, 3H), 3.27 (s, 2H), 2.91 (s, 2H), 2.02 (s, 2H), 1.80 (s, 2H). 13C NMR (101 MHz, CDCl3) δ 159.54, 142.11, 132.99, 130.85, 129.04, 127.43, 127.41, 126.91, 126.60, 124.94, 120.53, 113.46, 55.38, 29.13, 26.20, 22.89, 22.55. HRMS (ESI): m/z called for C24H21NO [M + H]+, 340.1696; found, 340.1708.
6-Fluoro-4-butyl-2-phenylquinoline (4bh). Yield, 91%; white solid; mp 245–246 °C; Rf 0.43 (petroleum ether/ethyl acetate = 10
:
1); IR (KBr): 3070, 2951, 2922, 1592, 1491, 1453 cm−1; 1H NMR (400 MHz, CDCl3) δ 7.96 (dd, J = 7.5, 1.7 Hz, 2H), 7.46 (s, 2H), 7.16 (d, J = 4.8 Hz, 2H), 7.08 (s, 2H), 5.95 (s, 1H), 2.37 (s, 2H), 1.52 (s, 2H), 1.32 (s, 2H), 0.86 (s, 3H). 13C NMR (101 MHz, CDCl3) δ 188.92, 167.07, 162.06, 159.61, 140.10, 134.50, 134.47, 130.86, 128.25, 127.38, 127.30, 127.04, 116.10, 92.83, 31.90, 30.34, 22.34, 13.63. HRMS (ESI): m/z called for C19H18FN [M + H]+, 280.1455; found, 280.1457.
6-Chloro-4-butyl-2-phenylquinoline (4bi). Yield, 92%; white solid; mp 237–238 °C; Rf 0.45 (petroleum ether/ethyl acetate = 10
:
1); IR (KBr): 3071, 2953, 2924, 1593, 1491, 1452 cm−1; 1H NMR (400 MHz, CDCl3) δ 7.97 (d, J = 1.3 Hz, 2H), 7.47 (s, 2H), 7.36 (s, 2H), 7.13 (s, 2H), 5.97 (s, 1H), 2.41 (s, 2H), 1.53 (s, 2H), 1.34 (s, 2H), 0.87 (s, 3H). 13C NMR (101 MHz, CDCl3) δ 189.04, 166.39, 139.99, 137.17, 131.43, 130.98, 129.30, 128.28, 127.07, 126.42, 93.37, 31.96, 30.33, 22.35, 13.68. HRMS (ESI): m/z called for C19H18ClN [M + H]+, 296.1107; found, 296.1109.
6-Bromo-4-butyl-2-phenylquinoline (4bj). Yield, 92%; white solid; mp 240–241 °C; Rf 0.47 (petroleum ether/ethyl acetate = 10
:
1); IR (KBr): 3073, 2954, 2925, 1597, 1495, 1451 cm−1; 1H NMR (400 MHz, CDCl3) δ 7.94 (dd, J = 7.9, 1.6 Hz, 2H), 7.50 (s, 4H), 7.09 (s, 2H), 5.96 (s, 1H), 2.42 (d, J = 8.0 Hz, 2H), 1.53 (d, J = 7.5 Hz, 2H), 1.34 (d, J = 7.5 Hz, 2H), 0.88 (s, 3H). 13C NMR (101 MHz, CDCl3) δ 189.12, 166.30, 139.96, 137.68, 132.28, 130.98, 128.28, 127.05, 126.74, 119.23, 93.45, 31.97, 30.34, 22.35, 13.67. HRMS (ESI): m/z called for C19H18BrN [M + H]+, 340.0696; found, 340.0693.
6-Iodo-4-butyl-2-phenylquinoline (4bk). Yield, 94%; white solid; mp 245–246 °C; Rf 0.51 (petroleum ether/ethyl acetate = 10
:
1); IR (KBr): 3075, 2959, 2928, 1599, 1495, 1453 cm−1; 1H NMR (400 MHz, CDCl3) δ 7.98–7.90 (m, 2H), 7.71 (d, J = 8.5 Hz, 2H), 7.47 (s, 2H), 6.97 (s, 2H), 5.96 (s, 1H), 2.44 (s, 2H), 1.55 (s, 2H), 1.35 (d, J = 7.5 Hz, 2H), 0.89 (s, 3H). 13C NMR (101 MHz, CDCl3) δ 189.13, 166.17, 139.95, 138.39, 138.24, 130.99, 128.27, 127.05, 126.90, 93.55, 90.04, 31.98, 30.34, 22.35, 13.67. HRMS (ESI): m/z called for C19H18IN [M + H]+, 388.0533; found, 388.0537.
2,4-Diphenylquinoline (4bl). Yield, 90%; white solid; mp 243–245 °C; Rf 0.53 (petroleum ether/ethyl acetate = 10
:
1); IR (KBr): 3055, 3028, 2920, 1589, 1546, 1488, 1444 cm−1; 1H NMR (400 MHz, CDCl3) δ 8.29 (d, J = 8.4 Hz, 1H), 8.23 (d, J = 7.3 Hz, 2H), 7.93 (d, J = 8.3 Hz, 1H), 7.85 (s, 1H), 7.75 (s, 1H), 7.58 (d, J = 1.7 Hz, 7H), 7.51–7.46 (m, 2H). 13C NMR (101 MHz, CDCl3) δ 156.87, 149.15, 148.83, 139.66, 138.41, 130.14, 129.55, 129.49, 129.32, 128.81, 128.58, 128.38, 127.58, 126.31, 125.77, 125.62, 119.33. HRMS (ESI): m/z called for C21H15N [M + H]+, 282.1277; found, 282.1279.
6-Methyl-2,4-diphenylquinoline (4bm). Yield, 91%; white solid; mp 244–247 °C; Rf 0.52 (petroleum ether/ethyl acetate = 10
:
1); IR (KBr): 3054, 2915, 1588, 1544, 1488, 1449 cm−1; 1H NMR (400 MHz, CDCl3) δ 8.26 (s, 1H), 7.99 (s, 2H), 7.71 (s, 1H), 7.41 (s, 6H), 6.94 (s, 2H), 6.72 (d, J = 7.5 Hz, 2H), 6.09 (s, 1H), 2.26 (s, 3H). 13C NMR (101 MHz, CDCl3) δ 189.40, 161.72, 139.95, 136.78, 135.90, 134.10, 133.85, 133.05, 131.17, 130.78, 129.56, 129.29, 128.48, 128.32, 127.20, 123.19, 96.54, 20.76. HRMS (ESI): m/z called for C22H17N [M + H]+, 296.1443; found, 295.1445.
6-Methoxy-4-ethyl-2-propylquinoline(4bn). Yield, 90%; yellow oil; mp 241–243 °C; Rf 0.55 (petroleum ether/ethyl acetate = 10
:
1); IR (KBr): 3052, 2913, 1586, 1542, 1487, 1448 cm−1; 1H NMR (400 MHz, CDCl3) δ 7.82 (d, J = 9.1 Hz, 1H), 7.66 (s, 1H), 7.18 (d, J = 2.6 Hz, 1H), 6.91 (d, J = 2.4 Hz, 1H), 3.80 (s, 3H), 2.83 (s, 2H), 2.71 (s, 2H), 1.74 (s, 2H), 1.23 (s, 3H), 0.97 (s, 3H). 13C NMR (101 MHz, CDCl3) δ 158.32, 156.13, 141.50, 134.59, 131.87, 128.91, 127.14, 119.73, 103.68, 54.41, 36.54, 24.17, 21.87, 13.45, 13.31.
Acknowledgements
This work was supported by the grant from National Natural Science Foundation of China (21571121, 21271124, and 21446014), and the Fundamental Research Funds for the Central Universities (No. GK201302015, No. GK201501005) for financial support, the 111 Project (B14041), the Program for Chang jiang Scholars and Innovative Research Team in University (IRT_14R33), the Project Supported by Natural Science Basic Research Plan in Shaanxi Province of China (Program No. 2015JQ2056).
Notes and references
-
(a) G. Jiang and B. List, Angew. Chem., Int. Ed., 2011, 50, 9471–9474 CrossRef CAS PubMed;
(b) R. De Vreese and M. D'hooghe, Beilstein J. Org. Chem., 2012, 8, 398–402 CrossRef CAS PubMed.
- J. Li, L. L. Lin, B. W. Hu, X. J. Lian, G. Wang, X. H. Liu and X. F. Feng, Angew. Chem., Int. Ed., 2016, 55, 6075–6078 CrossRef CAS PubMed.
- B. D. Robertson, R. E. M. Brooner and R. A. Widenhoefer, Chem.–Eur. J., 2015, 21, 5714–5717 CrossRef CAS PubMed.
- S. Zhang, Z. L. Xu, J. Jia, C. H. Tung and Z. H. Xu, Chem. Commun., 2014, 50, 12084–12087 RSC.
- G. B. Dong, P. Teo, Z. K. Wickens and R. H. Grubbs, Science, 2011, 333, 1609–1612 CrossRef CAS PubMed.
- S. Dhiman, U. K. Mishra and S. Ramasastry, Angew. Chem., Int. Ed., 2016, 55, 7737–7741 CrossRef CAS PubMed.
-
(a) Z. Y. Han, H. Xiao, X. H. Chen and L. Z. Gong, J. Am. Chem. Soc., 2009, 131, 9182–9183 CrossRef CAS PubMed;
(b) C. Wang, Z. Y. Han, H. W. Luo and L. Z. Gong, Org. Lett., 2010, 12, 2266–2269 CrossRef CAS PubMed;
(c) Z. Y. Han, D. F. Chen, Y. Y. Wang, R. Guo, P. S. Wang, C. Wang and L. Z. Gong, J. Am. Chem. Soc., 2012, 134, 6532–6535 CrossRef CAS PubMed;
(d) J. Mo, D. Eom, E. Lee and P. H. Lee, Org. Lett., 2012, 14, 3684–3687 CrossRef CAS PubMed;
(e) H. Wu, Y. P. He and L. Z. Gong, Org. Lett., 2013, 15, 460–463 CrossRef CAS PubMed;
(f) D. Qian and J. L. Zhang, Chem.–Eur. J., 2013, 19, 6984–6988 CrossRef CAS PubMed;
(g) Y. Horino, Y. Takahashi, Y. Nakashima and H. Abe, RSC Adv., 2014, 4, 6215–6218 RSC;
(h) X. Wu, M. L. Li and P. S. Wang, J. Org. Chem., 2014, 79, 419–425 CrossRef CAS PubMed;
(i) S. Dhiman and S. S. V. Ramasastry, Org. Lett., 2015, 17, 5116–5119 CrossRef CAS PubMed;
(j) Y. N. Gao, F. C. Shi, Q. Xu and M. Shi, Chem.–Eur. J., 2016, 22, 6803–6807 CrossRef CAS PubMed;
(k) F. Zhao, N. Li, Y. F. Zhu and Z. Y. Han, Org. Lett., 2016, 18, 1506–1509 CrossRef CAS PubMed.
-
(a) M. Terada and Y. Toda, Angew. Chem., Int. Ed., 2012, 51, 2093–2097 CrossRef CAS PubMed;
(b) Z. Y. Cao, Y. L. Zhao and J. Zhou, Chem. Commun., 2016, 52, 2537–2540 RSC;
(c) Y. L. Zhao, Z. Y. Cao, X. P. Zeng, J. M. Shi, Y. H. Yu and J. Zhou, Chem. Commun., 2016, 52, 3943–3946 RSC.
- G. X. Jiang and B. List, Adv. Synth. Catal., 2011, 353, 1667–1670 CrossRef CAS.
- H. Zhang, L. Zhu, S. Z. Wang and Z. J. Yao, J. Org. Chem., 2014, 79, 7063–7074 CrossRef CAS PubMed.
-
(a) K. Sorimachi and M. Terada, J. Am. Chem. Soc., 2008, 130, 14452–14453 CrossRef CAS PubMed;
(b) Q. A. Chen, D. S. Wang, Y. G. Zhou, Y. Duan, H. J. Fan, Y. Yang and Z. Zhang, J. Am. Chem. Soc., 2011, 133, 6126–6129 CrossRef CAS PubMed.
-
(a) H. Wu, Y. P. He and L. Z. Gong, Adv. Synth. Catal., 2012, 354, 975–980 CrossRef CAS;
(b) D. F. Chen, P. Y. Wu and L. Z. Gong, Org. Lett., 2013, 15, 3958–3961 CrossRef CAS PubMed;
(c) D. F. Chen, F. Zhao, Y. Hu and L. Z. Gong, Angew. Chem., Int. Ed., 2014, 53, 10763–10767 CrossRef CAS PubMed;
(d) L. Deiana, Y. Jiang, C. P. Nieto, S. Afewerki, C. Pradillos, O. Verho, C. Tai, E. V. Johnston and A. Córdova, Angew. Chem., Int. Ed., 2014, 53, 3447–3451 CrossRef CAS PubMed;
(e) D. F. Chen, Z. Y. Han, X. L. Zhou and L. Z. Gong, Acc. Chem. Res., 2014, 47, 2365–2377 CrossRef CAS PubMed;
(f) X. P. Yin, X. P. Zeng, Y. L. Liu, F. M. Liao, J. S. Yu, F. Zhou and J. Zhou, Angew. Chem., Int. Ed., 2014, 53, 13740–13745 CrossRef CAS PubMed;
(g) T. Suzuki, Ismiyarto, Y. Ishizaka, D. Y. Zhou, K. Asano and H. Sasai, Org. Lett., 2015, 17, 5176–5179 CrossRef CAS PubMed;
(h) X. L. Lian, J. Meng and Z. Y. Han, Org. Lett., 2016, 18, 4270–4273 CrossRef CAS PubMed;
(i) C. C. Pattillo, I. I. Strambeanu, P. Calleja, N. A. Vermeulen, T. Mizuno and M. C. White, J. Am. Chem. Soc., 2016, 138, 1265–1272 CrossRef CAS PubMed;
(j) M. Lesieur, Y. D. Bidal, F. Lazreg, F. Nahra and C. S. J. Cazin, ChemCatChem, 2015, 7, 2108–2112 CrossRef CAS.
- H. Yeom, Y. Lee, J. Jeong, E. So, S. Hwang, J. E. Lee, S. S. Lee and S. Shin, Angew. Chem., Int. Ed., 2010, 49, 1611–1614 CrossRef CAS PubMed.
-
(a) N. G. Kozlov and L. I. Basalaeva, Russ. J. Org. Chem., 2009, 45, 587–590 CrossRef CAS;
(b) N. G. Kozlov and L. I. Basalaeva, Russ. J. Org. Chem., 2001, 71, 250–256 CrossRef CAS.
- X. S. Wang, Q. Li, J. R. Wu, Y. L. Li, C. S. Yao and S. J. Tu, Synthesis, 2008, 12, 1902–1910 CrossRef.
- F. H. Xiao, W. Chen, Y. F. Liao and G. J. Deng, Org. Biomol. Chem., 2012, 10, 8593–8596 CAS.
- W. Wei, J. W. Wen, D. S. Yang, X. J. Sun, J. M. You, Y. R. Suo and H. Wang, Tetrahedron, 2013, 69, 10747–10751 CrossRef CAS.
- X. F. Xu, W. M. Liu, Z. Q. Wang, Y. Q. Feng, Y. L. Yan and X. Zhang, Tetrahedron Lett., 2016, 57, 226–229 CrossRef CAS.
-
(a) Y. Wu, C. Chen, G. Jia, X. Y. Zhu, H. M. Sun, G. F. Zhang, W. Q. Zhang and Z. W. Gao, Chem.–Eur. J., 2014, 20, 8530–8535 CrossRef CAS PubMed;
(b) X. Y. Zhu, C. Chen, B. X. Yu, G. F. Zhang, W. Q. Zhang and Z. W. Gao, Chem. Lett., 2014, 43, 1832–1834 CrossRef;
(c) Y. Wu, X. Wang, Y. L. Luo, J. Wang, Y. J. Jian, H. M. Sun, G. F. Zhang, W. Q. Zhang and Z. W. Gao, RSC Adv., 2016, 6, 15298–15303 RSC;
(d) X. Wang, Z. H. Wang, G. F. Zhang, W. Q. Zhang, Y. Wu and Z. W. Gao, Eur. J. Org. Chem., 2016, 3, 502–507 Search PubMed;
(e) Y. L. Luo, Y. Wu, Y. Y. Wang, H. M. Sun, Z. Y. Xie, W. Q. Zhang and Z. W. Gao, RSC Adv., 2016, 6, 66074–66077 RSC.
- M. Daigle, W. H. Bi, M. A. Legare, J. F. Morin and F. G. Fontaine, Inorg. Chem., 2015, 54, 5547–5555 CrossRef CAS PubMed.
-
(a) D. Zhan, T. B. Li, H. D. Wei, W. Weng, K. Ghandi and Q. L. Zeng, RSC Adv., 2013, 3, 9325–9329 RSC;
(b) R. Jalil, W. Voelter and M. Saeed, Tetrahedron Lett., 2004, 45, 3475–3476 CrossRef;
(c) I. E. Marko, P. R. Giles, M. Tsukazaki, S. M. Brown and C. J. Urch, Science, 1996, 274, 2044–2046 CrossRef CAS PubMed;
(d) S. Velusamy and T. Punniyamurthy, Tetrahedron Lett., 2003, 44, 8955–8957 CrossRef CAS.
Footnote |
† Electronic supplementary information (ESI) available. See DOI: 10.1039/c7ra00870h |
|
This journal is © The Royal Society of Chemistry 2017 |
Click here to see how this site uses Cookies. View our privacy policy here.