DOI:
10.1039/C7RA01142C
(Paper)
RSC Adv., 2017,
7, 38044-38051
Wide band gap design of new chalcogenide compounds: KSrPS4 and CsBaAsS4†
Received
25th January 2017
, Accepted 19th July 2017
First published on 2nd August 2017
Abstract
Recently, the exploration of infrared nonlinear optical (IR NLO) materials has mainly focused on chalcogenide compounds. However, their practical applications are often hampered by the low laser damage thresholds (LDTs). It is known that wide band gaps can significantly enhance the LDTs of materials, and the introduction of alkali and alkaline earth cations would broaden the band gap. Accordingly, in this work two new compounds KSrPS4 and CsBaAsS4 with both alkali and alkaline earth cations were synthesized successfully. Both compounds crystallize in the space group Pnma (62) of the orthorhombic system, and the structures consist of isolated PnS4 (Pn = P, As) tetrahedra with the interstices occupied by K (or Cs) and Sr (or Ba) atoms, respectively. The band gaps of compounds were determined by different methods. The UV-visible diffuse reflectance spectra revealed that the band gaps of KSrPS4 and CsBaAsS4 are larger than 3.62 eV and 2.86 eV, respectively. The band gaps are primarily determined by the PnS4 tetrahedra.
Introduction
It is well known that coherent IR lasers have been widely applied in civil and military fields such as laser guidance, signal communication and industrial processing. A number of NLO materials have been applied to generate IR lasers. The commercial IR NLO materials are mainly focused on chalcogenide compounds, such as AgGaS2 and AgGaSe2,1,2 which have been applied in optoelectronic and thermoelectric areas because of their diverse structures and physical properties.3–8 However, these materials suffer from the low LDTs, which limits their further application in various areas. Here, the low LDT is principally caused by the relatively small band gap of the material. Materials with higher LDTs can be obtained based on broader band gaps. Numerous research has been conducted to search for novel, promising mid-IR NLO materials that exhibit broad band gaps and strong NLO responses.9–29 In our previous research for Ag3Ga3SiSe8 and AgGaSiSe4 compounds, it mentioned that the band gap of material can be enlarged through the introduction of the Ge or Si element.24,25 And compared to the band gap of AgGaSe2 (ref. 2) with 1.8 eV, the band gaps of AgGaSiSe4 (ref. 24) and Ag3Ga3SiSe8 (ref. 25) are broadened to 2.63 eV and 2.30 eV, respectively. The introduction of alkali or alkaline earth cations which usually are electropositive elements also contributes to broaden band gap of materia.26–28 For example, the band gaps of BaGa4S7 (ref. 26) and LiGaS2 (ref. 27) increased to 3.54 eV and 4.15 eV, respectively, compared to that of AgGaS2 with 2.75 eV. Those of BaGa4Se7 (ref. 28) and LiGaSe2 (ref. 27) increased to 2.64 eV and 3.34 eV, respectively, compared to that of AgGaSe2 with 1.8 eV. And with the introduction of both alkali and alkaline earth cations, the band gap of Na2BaGeS4 (ref. 29) reached 3.7 eV. Wherefore in this work, we attempted to simultaneously introduce the alkali and alkaline earth cations to broaden the band gap of material. Meanwhile the introduction of PnS4 (Pn = P, As) groups are conducive to improve the NLO properties.30 Through the combination of alkali and alkaline earth cations with the PnS4 groups, we conducted the research to the A/AL/Pn/S (A = alkali cations, AL = alkaline earth cations) system, and fortunately, the two new compounds KSrPS4 and CsBaAsS4 were successfully synthesized. The syntheses, crystal structures, electronic structures and optical properties of the materials were also investigated.
Experimental section
Synthesis
The compound KSrPS4 was prepared by combining K2S3 (prepared by stoichiometric reaction of the K (Sinopharm chemical Reagent Co., Ltd., 98%) and S (Sinopharm chemical Reagent Co., Ltd., 99.5%) elements in liquid NH3), SrS (prepared by stoichiometric reaction of Sr (Sinopharm chemical Reagent Co., Ltd., 99%) and S elements), Nd (Sinopharm chemical Reagent Co., Ltd., 99.9%), P2S5 (Sinopharm chemical Reagent Co., Ltd., 99%) and additional S (Sinopharm chemical Reagent Co., Ltd., 99.5%) in the molar ratio 2
:
1
:
1
:
1
:
6. The sample was heated in a computer-controlled furnace to 973 K, then kept there for 5 d, then cooled to 373 K at the rate of 3 K h−1. The resulting melts were washed with dimethylformamide (DMF) and acetone in turn. The product consisted of colorless transparent platelet KSrPS4. This compound was stable in dry air for several days.
The compound CsBaAsS4 was prepared by combining Cs2S3 (prepared by stoichiometric reaction of the Cs (Alfa Aesar, 99.8%) and S (Sinopharm chemical Reagent Co., Ltd., 99.5%) elements in liquid NH3), BaS (Sinopharm chemical Reagent Co., Ltd., 99%), Nd (Sinopharm chemical Reagent Co., Ltd., 99.9%), As2S3 (Sinopharm chemical Reagent Co., Ltd., 99%) and additional S (Sinopharm chemical Reagent Co., Ltd., 99.5%) in the molar ratio 2
:
0.5
:
1
:
1
:
6. The sample was heated in a computer-controlled furnace to 973 K, then kept there for 5 d, then cooled to 373 K at the rate of 3 K h−1. The resulting melts were washed with DMF and acetone in turn. The product consisted of yellow transparent platelet crystal of CsBaAsS4. This compound was stable in dry air for several days.
Energy dispersive X-ray fluorescence test
The analysis of the compounds was carried out with Shimadzu EDX-720 Energy Dispersive X-ray Fluorescence spectrometer. The tests for each compound were performed with 5 times, and the spectra of number 1 and 6, as shown in Fig. 1 (the rest of the number 2–5 and 7–10 spectra are shown in the ESI†), manifested the presence of K, Sr, P, S and Ca, Ba, As, S in the approximate molar ratio of 1
:
1
:
1
:
4 and 1
:
1
:
1
:
4, respectively.
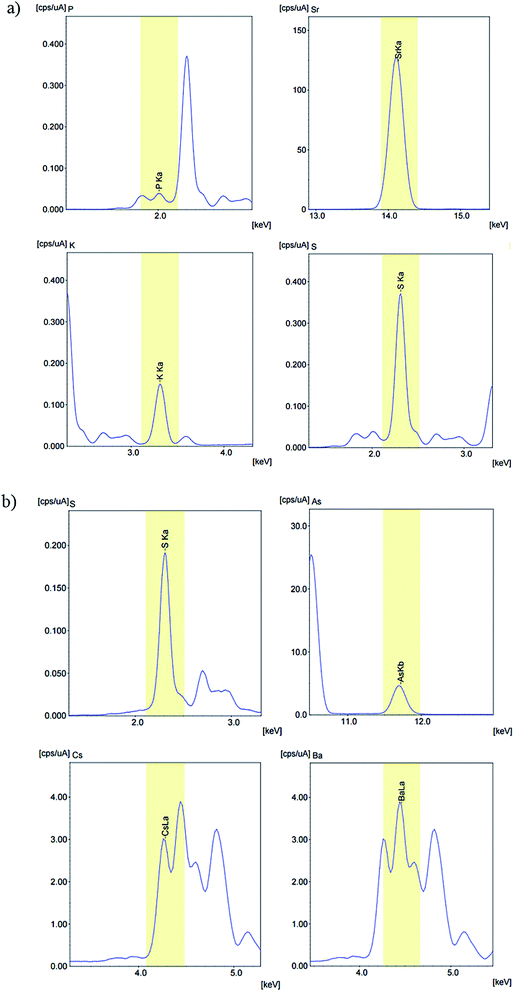 |
| Fig. 1 (a) Energy dispersive X-ray fluorescence spectra of KSrPS4, (b) energy dispersive X-ray fluorescence spectra of CsBaAsS4. | |
Single crystal data collection
Single crystal X-ray diffraction data were collected with graphite-monochromatized Mo K radiation (λ = 0.71073 Å) at 220 K on a STOE Imaging Plate Diffraction System (IPDS-1).
UV-vis diffuse reflectance test
UV-vis diffuse reflectance spectroscopy test was performed on a Shimadzu UV-3600 spectrophotometer. The samples and BaSO4 (totally reflected) were ground together at room temperature. Then the mixture was prepared as a flat specimen, and in UV/vis range, the resolution was 0.1 nm. The data were collected at 200–800 nm.
Second harmonic generation test
The optical second harmonic generation test was performed on the powder sample of KSrPS4 and CsBaAsS4 by means of the Kurtz–Perry method,31 with a 1.06 μm Q-switch laser. The samples were ground and sieved by using a series of mesh sizes in the range of 80–100 μm. Similar size of AgGaS2 was chosen as the reference.
Laser damage threshold test
The laser damage thresholds of title compounds were evaluated on powder sample with a pulsed YAG laser (1.06 μm, 10 ns, 10 Hz).32 Similar size of AgGaS2 was chosen as the reference.
Structure determination
The structure model was obtained by direct methods and was refined by full-matrix least-squares refinement based on F2 using the Shelxtl package.33 The positions were standardized with the Structure Tidy program within the Platon package.34 The final refinement converged with a residual factor of wR2 = 0.095 (all data). Technical details of the data acquisition as well as some refinement results are summarized in Table 1.
Table 1 Crystal data and structure refinement for KSrPS4 and CsBaAsS4
|
KSrPS4 |
CsBaAsS4 |
R(F) = ∑∣∣Fo∣ − ∣Fc∣∣/∑∣Fo∣ for Fo2 > 2σ(Fo2). Rw(Fo2) = {∑[w(Fo2 − Fc2)2]/∑wFo4}1/2 for all data. |
Fw (g mol−1) |
285.93 |
473.41 |
a (Å) |
16.8214(9) |
11.9066(6) |
b (Å) |
6.6274(5) |
6.9184(5) |
c (Å) |
6.5585(4) |
10.0338(5) |
V (Å3) |
731.16(8) |
826.53(8) |
Space group |
Pnma (62) |
Pnma (62) |
Z |
4 |
4 |
Index ranges |
−21 ≤ h ≤ 22 |
−15 ≤ h ≤ 15 |
−8 ≤ k ≤ 8 |
−9 ≤ k ≤ 9 |
−8 ≤ l ≤ 8 |
−13 ≤ l ≤ 12 |
Theta range |
2.42–28.08 |
2.65–28.08 |
Number of reflection collected |
873 |
1029 |
ρc (g cm−3) |
2.598 |
3.804 |
μ (cm−1) |
91.84 |
140.24 |
R(F)a |
0.0292 |
0.0309 |
Rw(Fo2)b |
0.0770 |
0.0775 |
The first-principles calculations
The first-principles calculations for the KSrPS4 and CsBaAsS4 crystals are performed by the plane-wave pseudo potential method implemented in the CASTEP package based on the density functional theory (DFT).35 The ion–electron interactions are modeled by the optimized normal-conserving pseudo potentials for all elements. The adopted density functional method is exchange-correlation (XC) functional of local density approximation (LDA).36 The kinetic energy cutoffs of 800 eV and Monkhorst–Pack k-point meshes37 with density of (2 × 3 × 2) and (1 × 3 × 3) points in the Brillouin zone are chosen for KSrPS4 and CsBaAsS4 crystals, respectively. Our tests reveal that the above computational set ups are sufficiently accurate for the present purposes.
Results and discussion
The two compounds KSrPS4 and CsBaAsS4 crystallize in space group Pnma (62) of the orthorhombic system and adopt the TlEuPS4 (ref. 38) structure type, As shown in Fig. 2. The structures consist of isolated PnS4 (Pn = P, As) tetrahedra separated by the K (or Cs) and Sr (or Ba) atoms, and the atoms locate in the tunnel formed by the tetrahedra, respectively. The asymmetric units for both compounds contain one crystallographically independent K (or Cs) atom, one independent Sr (or Ba) atom, one independent P (or As) atom and three independent S atoms.
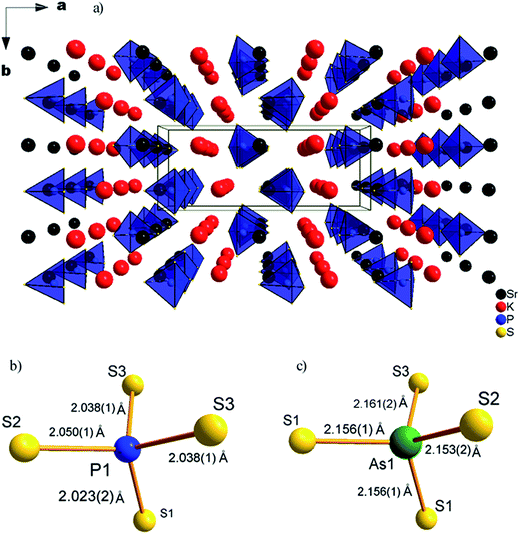 |
| Fig. 2 (a) Unit cell structure of KSrPS4, (b) PS4 tetrahedron in KSrPS4 structure, and (c) AsS4 tetrahedron in CsBaAsS4 structure. | |
For KSrPS4, K atom is coordinated to a bicapped trigonal prism of eight S atoms with K–S distances ranging from 3.336(1) to 3.603(2) Å, which is close to those of 3.2058(14)–3.5239(14) Å for K–S distances in KBiSiS4 (ref. 39) and 3.194(3)–3.601(3) Å for K–S distances in KBiGeS4.39 The Sr atom is coordinated to 9 S atoms with Sr–S distances ranging from 2.992(1) to 3.685(1) Å, which is close to those of 2.928–3.242 Å for Sr–S distances in Sr2ZnS3.40 Each P atom is bonded to four S atoms to form a slightly distorted tetrahedron, with the bond lengths ranging from 2.023(2) to 2.049(8) Å, similar to those of 2.010(1)–2.090(1) Å for P–S distances in LiZnPS4.41 Since S–S bonds are not observed in the structure, the oxidation states of 1+, 2+, 5+, 2− can be distributed to K, Sr, P and S, respectively.
For CsBaAsS4, Cs atom is bonded to 10 S atoms with Cs–S distances ranging from 3.468(2) to 3.895(1) Å, which is close to those of 3.4022(10)–4.0982(13) Å for Cs–S distances in Cs3Bi(AsS4)2.42 While Ba is coordinated to 9 S atoms with Ba–S distances ranging from 3.182(2) to 3.588(0) Å, similar to those of 2.896(2)–3.331(2) Å for Ba–S distances in Ba23Ga8Sb2S38.43 Each As atom is bonded to four S atoms to form a slightly distorted tetrahedron, with the As–S bond lengths ranging from 2.153(4) to 2.161(1) Å, which is similar to those of 1.918(3)–2.390(3) Å for As–S distances in Cs3Bi(AsS4)2 and 2.229(2)–2.266(2) Å for As–S distances in Cs3Ta2AsS11.44 Since S–S bonds are not observed in the structure, the oxidation states of 1+, 2+, 5+, 2− can be distributed to Cs, Ba, As and S, respectively.
For these compounds, the larger content of alkali cations (K, Cs) and alkaline cations (Ba, Sr) is, the sparser the PS4 and AsS4 tetrahedra connectivity will be, which can be referred to our previous work on Ba5Al2Se8 and Ba5Ga2Se8.45 And the tetrahedra would be completely separated from each other by the K (or Cs) and Sr (or Ba) atoms.
From the UV-visible diffuse reflectance spectra of the compounds in Fig. 3(a), the absorption edges are about 343 nm and 434 nm for KSrPS4 and CsBaAsS4, respectively. The band gaps of KSrPS4 and CsBaAsS4 obtained by direct extrapolation method46 with baseline tangents were 3.62 and 2.86 eV, respectively. To correctly compared the band gaps of different compounds, the measurement methods need to be consistent. And in the Fig. 3(b), the direct extrapolation method with upper tangents were carried out on the spectra, and the results showed that the band gaps of compounds are 4.23 eV and 3.25 eV, respectively. These two compounds band gaps are significantly larger than that of the commercial materials AgGaS2 (ref. 1) with 2.75 eV and AgGaSe2 (ref. 2) with 1.8 eV. The band gaps of KSrPS4 and CsBaAsS4 are also larger than that of BaGa4S7 (ref. 26) with 3.54 eV and BaGa4Se7 (ref. 28) with 2.64 eV, respectively, indicated that compounds with large band gaps were obtained. According to the single crystal growth experiment results, the colors for KSrPS4 and CsBaAsS4 crystals are colorless and yellow (see in Fig. 4), respectively, which was in good accordance with the UV-visible reflectance spectra. In the Fig. 3(c) and (d), the direct and indirect band gaps are measured by Tauc–Davis Mott expressions.47 The measurement of direct band gap indicated that KSrPS4 and CsBaAsS4 is 3.92 eV and 2.97 eV, respectively. And the measurement of indirect band gap indicated that KSrPS4 and CsBaAsS4 is 3.84 eV and 2.91 eV, respectively.
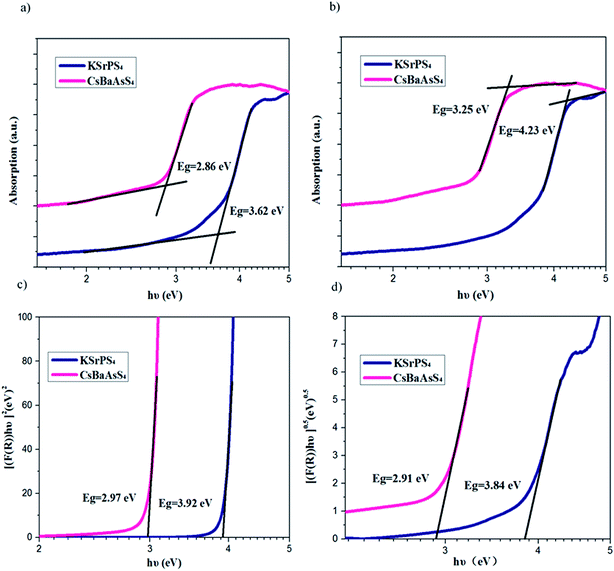 |
| Fig. 3 Diffuse spectra of KSrPS4 and CsBaAsS4: (a) the spectra with upper tangents; (b) the spectra with baseline tangents; (c) the spectra of (F(R) hυ)2 versus hυ; (d) the spectra of (F(R) hυ)0.5 versus hυ. | |
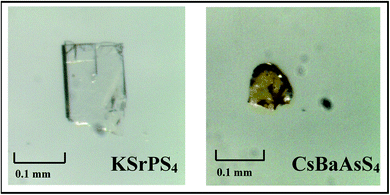 |
| Fig. 4 The single crystals of KSrPS4 and CsBaAsS4 compounds. | |
The laser damage test was performed on the powder sample with a pulsed YAG laser (1.06 μm, 10 ns, 10 Hz). AgGaS2 was served as the reference. The results showed that both the laser damage thresholds of KPSrS4 and CsBaAsS4 are above 5 times of that of AgGaS2. The optical second harmonic generation test was performed on KSrPS4 and CsBaAsS4 with a 1.06 μm Q-switch laser. The compounds KSrPS4 and CsBaAsS4 did not give any second harmonic generation signal because of their centrosymmetric structure.
The electronic band structures of the KSrPS4 and CsBaAsS4 crystals are shown in Fig. 5. The calculations with sX-LDA functional were carried out to investigate the experimental values of band gaps and the results showed the values of 3.58 eV and 2.73 eV for KSrPS4 and CsBaAsS4, respectively.
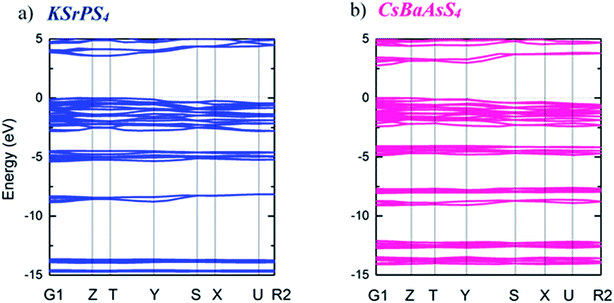 |
| Fig. 5 (a) The band structure of KSrPS4, and (b) band structure of CsBaAsS4. | |
The partial density of state (PDOS) projected on the constitutional atoms of the KSrPS4 and CsBaAsS4 are shown in Fig. 6, from which several electronic characteristics are shown: (i) the region lowered than −5 eV consist of the isolated inner-shell states with K 2s2p (or Cs 5s5p), Sr 4s4p (or Ba 5s5p), P 3s2p (or As 4s3p) and S 3s2p orbitals, which have little interaction with neighbor atoms. (ii) The upper part of the valence band (about −4 eV) is mainly composed of the p orbitals of P 3p (As 4p) and S 3p orbitals, but the extra top of the VB is occupied by the S 3p orbitals. (iii) Although all the elements contribute to the states on the bottom of conduct band, states on the bottom of conduct band mostly come from the S and P (As) atoms.
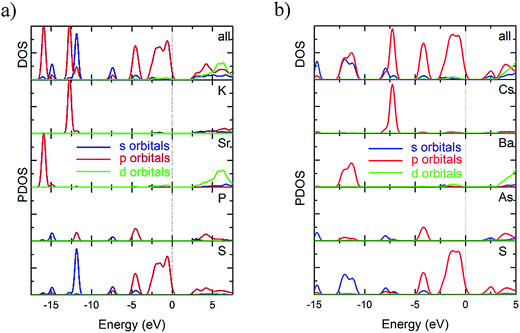 |
| Fig. 6 (a) The PDOS of KSrPS4, and (b) PDOS of CsBaAsS4 crystals. | |
In summary, the upper part of valence band and the bottom of conduct band are principally determined by the P (As) and S elements, which indicated that the optical absorption is mostly determined by (PnS4)3− (Pn = P, As) groups.
To further investigate the relationship between the structures and properties of the compounds, the contours of electronic density difference on the PS4 and AsS4 groups were drawn for the title compounds in Fig. 7, which illustrate charge redistribution due to the formation of chemical bonds. It is obvious that more charges are located on the P–S bonds than on the As–S bonds, indicating the much stronger covalent characteristic of the former chemical bonds.
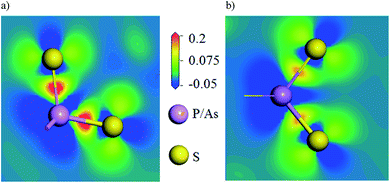 |
| Fig. 7 (a) The electronic charge density difference plotting on the PS4 groups, and (b) AsS4 groups. | |
Conclusions
To obtain compounds with wide band gaps, in this work, introduction of both the alkali and alkaline earth cations was carried out. Two new compounds KSrPS4 and CsBaAsS4 were successfully synthesized. The compounds crystallize in space group Pnma (62) of the orthorhombic system and adopt the TlEuPS4 structure type. The band gaps of compounds were determined by four methods. The results revealed that the band gaps of KSrPS4 and CsBaAsS4 are larger than 3.62 eV and 2.86 eV, respectively, which imply that the band gaps of compounds were successfully broadened compared with the commercial materials AgGaS2 and AgGaSe2, respectively. The band gaps are primarily determined by the PnS4 (Pn = P, As) tetrahedra. Such compounds may arouse further interest in exploring new IR NLO materials with wide band gaps, through introducing the alkali or alkaline earth cations.
Acknowledgements
This research was supported by the National Natural Science Foundation of China (No. 51502169), China “863” project (No. 2015AA034203), Opening Project of Key Laboratory of Green Catalysis of Sichuan Institutes of High Education (No. LZJ1503), Shanghai University of Engineering Science “Innovation Training Project of Undergraduate” (No. cs1504002) and Shanghai University of Engineering Science “Scientific Research Training Project of Undergraduate” (No. HZJY20160029).
References
- D. Chemla, P. Kupecek, D. Robertson and R. Smith, Opt. Commun., 1971, 3, 29 CrossRef CAS.
- R. K. Route, R. S. Feigelson and R. J. Raymakers, J. Cryst. Growth, 1974, 24, 390 CrossRef.
- K. Ramanathan, M. A. Contreras, C. L. Perkins, S. Asher, F. S. Hasoon, J. Keane, D. Young, M. Romero, W. Metzger, R. Noufi, J. Ward and A. Duda, Prog. Photovoltaics, 2003, 11, 225 CAS.
- K. Biswas, J. He, I. D. Blum, C.-I. Wu, T. P. Hogan, D. N. Seidman, V. P. Dravid and M. G. Kanatzidis, Nature, 2012, 489, 414 CrossRef CAS PubMed.
- Y. Pei, X. Shi, A. LaLonde, H. Wang, L. Chen and G. J. Snyder, Nature, 2011, 473, 66 CrossRef CAS PubMed.
- S. N. Guin, A. Chatterjee, D. S. Negi, R. Datta and K. Biswas, Energy Environ. Sci., 2013, 6, 2603 CAS.
- S. Roychowdhury, U. S. Shenoy, U. V. Waghmare and K. Biswas, Angew. Chem., Int. Ed., 2015, 54, 15241 CrossRef CAS PubMed.
- S. N. Guin, J. Pan, A. Bhowmik, D. Sanyal, U. V. Waghmare and K. Biswas, J. Am. Chem. Soc., 2014, 136, 12712 CrossRef CAS PubMed.
- W. Yin, K. Feng, R. He, D. Mei, Z. Lin, J. Yao and Y. Wu, Dalton Trans., 2012, 41, 5653 RSC.
- Z.-Z. Luo, C.-S. Lin, W.-L. Zhang, H. Zhang, Z.-Z. He and W.-D. Cheng, Chem. Mater., 2013, 26, 1093 CrossRef.
- G. Zhang, Y. Li, K. Jiang, H. Zeng, T. Liu, X. Chen, J. Qin, Z. Lin, P. Fu, Y. Wu and C. Chen, J. Am. Chem. Soc., 2012, 134, 14818 CrossRef CAS PubMed.
- A. S. Haynes, F. O. Saouma, C. O. Otieno, D. J. Clark, D. P. Shoemaker, J. I. Jang and M. G. Kanatzidis, Chem. Mater., 2015, 27, 1837 CrossRef CAS.
- D. Mei, W. Yin, L. Bai, Z. Lin, J. Yao, P. Fu and Y. Wu, Dalton Trans., 2011, 40, 3610 RSC.
- D. Mei, W. Yin, K. Feng, Z. Lin, L. Bai, J. Yao and Y. Wu, Inorg. Chem., 2012, 51, 1035 CrossRef CAS PubMed.
- H. Lin, L. Chen, L. J. Zhou and L. M. Wu, J. Am. Chem. Soc., 2013, 135, 12914 CrossRef CAS PubMed.
- Y. Kim, I. S. Seo, S. W. Martin, J. Baek, P. S. Halasyamani, N. Arumugam and H. Steinfink, Chem. Mater., 2008, 20, 6048 CrossRef CAS.
- Z. H. Kang, J. Guo, Z. S. Feng, J. Y. Gao, J. J. Xie, L. M. Zhang, V. Atuchin, Y. Andreev, G. Lanskii and A. Shaiduko, Appl. Phys. B, 2012, 108, 545 CrossRef CAS.
- V. P. Sachanyuk, G. P. Gorgut, V. V. Atuchin, I. D. Olekseyuk and O. V. Parasyuk, J. Alloys Compd., 2008, 452, 348 CrossRef CAS.
- I. Chung, J. H. Song, J. I. Jang, A. J. Freeman, J. B. Ketterson and M. G. Kanatzidis, J. Am. Chem. Soc., 2009, 131, 2647 CrossRef CAS PubMed.
- M.-J. Zhang, X.-M. Jiang, L.-J. Zhou and G.-C. Guo, J. Mater. Chem. C, 2013, 1, 4754 RSC.
- K. Wu, Z. Yang and S. Pan, Inorg. Chem., 2015, 54, 10108 CrossRef CAS PubMed.
- J. A. Brant, D. J. Clark, Y. S. Kim, J. I. Jang, J.-H. Zhang and J. A. Aitken, Chem. Mater., 2014, 26, 3045 CrossRef CAS.
- M. V. Shevchuk, V. V. Atuchin, A. V. Kityk, A. O. Fedorchuk, Y. E. Romanyuk, S. CaŁus, O. M. Yurchenko and O. V. Parasyuk, J. Cryst. Growth, 2011, 318, 708 CrossRef CAS.
- S. Zhang, D. Mei, X. Du, Z. Lin, J. Zhong, Y. Wu and J. Xu, J. Solid State Chem., 2016, 238, 21 CrossRef CAS.
- D. Mei, P. Gong, Z. Lin, K. Feng, J. Yao, F. Huang and Y. Wu, CrystEngComm, 2014, 16, 6836 RSC.
- X. Lin, G. Zhang and N. Ye, Cryst. Growth Des., 2009, 9, 1186 CAS.
- L. Isaenko, A. Yelisseyev, S. Lobanov, A. Titov, V. Petrov, J. J. Zondy, P. Krinitsin, A. Merkulov, V. Vedenyapin and J. Smirnova, Cryst. Res. Technol., 2003, 38, 379 CrossRef CAS.
- J. Yao, D. Mei, L. Bai, Z. Lin, W. Yin, P. Fu and Y. Wu, Inorg. Chem., 2010, 49, 9212 CrossRef CAS PubMed.
- K. Wu, Z. Yang and S. Pan, Angew. Chem., Int. Ed., 2016, 55, 6713 CrossRef CAS PubMed.
- L. Kang, M. Zhou, J. Yao, Z. Lin, Y. Wu and C. Chen, J. Am. Chem. Soc., 2015, 137, 13049 CrossRef CAS PubMed.
- S. K. Kurtz and T. T. Perry, J. Appl. Phys., 1968, 39, 3798 CrossRef CAS.
- G. Li, K. Wu, Q. Liu, Z. Yang and S. Pan, J. Am. Chem. Soc., 2016, 138, 7422 CrossRef CAS PubMed.
- G. M. Sheldrick, Acta Crystallogr., Sect. A: Found. Crystallogr., 2008, 64, 112 CrossRef CAS PubMed.
- A. L. Spek, J. Appl. Crystallogr., 2003, 36, 7 CrossRef CAS.
- P. Hohenberg and W. Kohn, Phys. Rev., 1964, 136, B864 CrossRef.
- W. Kohn and L. J. Sham, Phys. Rev., 1965, 140, A1133 CrossRef.
- H. J. Monkhorst and J. D. Pack, Phys. Rev. B: Condens. Matter Mater. Phys., 1976, 13, 5188 CrossRef.
- W. Carrillo-Cabrera, K. Peters, H. G. von Schnering, F. Menzel and W. Brockner, Z. Anorg. Allg. Chem., 1995, 621, 557 CrossRef CAS.
- D. Mei, Z. Lin, L. Bai, J. Yao, P. Fu and Y. Wu, J. Solid State Chem., 2010, 183, 1640 CrossRef CAS.
- V. Petrykin, M. Okube, H. Yamane, S. Sasaki and M. Kakihana, Chem. Mater., 2010, 22, 5800 CrossRef CAS.
- S. Jörgens, D. Johrendt and A. Mewis, Z. Anorg. Allg. Chem., 2002, 628, 1765 CrossRef.
- T. K. Bera, R. G. Iyer, C. D. Malliakas and M. G. Kanatzidis, Inorg. Chem., 2013, 52, 11370 CrossRef CAS PubMed.
- M.-C. Chen, L.-M. Wu, H. Lin, L.-J. Zhou and L. Chen, J. Am. Chem. Soc., 2012, 134, 6058 CrossRef CAS PubMed.
- T. K. Bera, J. I. Jang, J. B. Ketterson and M. G. Kanatzidis, J. Am. Chem. Soc., 2009, 131, 75 CrossRef CAS PubMed.
- D. Mei, W. Yin, Z. Lin, R. He, J. Yao, P. Fu and Y. Wu, J. Alloys Compd., 2011, 509, 2981 CrossRef CAS.
- O. Schevciw and W. B. White, Mater. Res. Bull., 1983, 18, 1059 CrossRef CAS.
- J. Tauc, R. Grigorovici and A. Vancu, Phys. Status Solidi, 1966, 15, 627 CrossRef CAS.
Footnote |
† Electronic supplementary information (ESI) available: Crystallographic data in CIF format for KSrPS4 and CsBaAsS4. CCDC 1502143 and 1503947. For ESI and crystallographic data in CIF or other electronic format see DOI: 10.1039/c7ra01142c |
|
This journal is © The Royal Society of Chemistry 2017 |
Click here to see how this site uses Cookies. View our privacy policy here.