DOI:
10.1039/C7RA06223K
(Paper)
RSC Adv., 2017,
7, 40952-40956
Acid-assisted hydrothermal synthesis of red fluorescent carbon dots for sensitive detection of Fe(III)†
Received
4th June 2017
, Accepted 7th August 2017
First published on 22nd August 2017
Abstract
Red-emitting carbon dots (C-dots) were synthesized from p-phenylenediamine (p-PD) aqueous solution with nitric acid (HNO3) assistance by hydrothermal reaction at 200 °C for 2 h. p-PD aqueous solution can be transferred to C-dots (or poly(p-PD)) with (or without) the addition of acid. Different acid systems, such as HNO3, H3PO4 and HF, can directly synthesize red-emitting C-dots, and the fluorescence can be enhanced by increasing the strength of acids. N-CDs, 3.46 nm-average-sized C-dots, prepared in dilute HNO3, have a quantum yield of 15.8% with unique, excitation-wavelength independent emission in the red region (600 and 680 nm) and a broad visible excitation band. Carboxyl, ester and hydroxyl groups on the C-dots surface directly lead to red emission. N-CDs have a certain selective specificity for Fe3+ detection and the linear range is 10–300 nmol L−1 with a limit of determination of 1.9 nmol L−1.
1. Introduction
Emerging as a new class of zero-dimensional nano-materials, carbon dots (C-dots) have been widely studied during the past decade. Thanks to their advantages including excellent water solubility, optical stability, unique fluorescence properties, low toxicity, low cost, etc.,1 C-dots can be applied in various fields, such as electrochemical immune-sensors,2 bio-imaging,3,4 sensors,5 photo-catalysis6 and optoelectronics.7
Various synthesis methods have been developed since carbon dots were discovered,8 and they can be mainly categorized into “top-down” and “bottom-up” routes.9 This classification is mainly based on carbon sources. The “top-down” route physically decomposes macro carbon materials into smaller and smaller nano materials, while the “bottom-up” route chemically creates C-dots by molecular self-assembly reactions from various small molecular reagents. Most of the “bottom-up” routes are efficient to prepare C-dots on a large scale10 and the carbon sources are widely available from various natural products (fruit juice,11–14 dried grass,15etc.) and small organic molecules.16 Because of the complex composition of natural products, the photoluminescence (PL) mechanism for C-dots need further study, and thus small molecular carbon sources have become hot research in recent years.
Isomers of phenylenediamine (PD),o-, m-, and p-PD, had been studied as a new carbon source for preparing o-CDs,5,17,18 m-CDs,16 and p-CDs,16,19 respectively, among which o-PD and m-PD are relatively easy to form C-dots, while there is challenge in employing p-PD. p-PD has been studied and proposed as a carbon source since 2015,17 and the as-prepared p-CDs emit red fluorescence. After that, Ding et al.19 prepared full-colour C-dots from p-PD aqueous solution and urea, and utilized a complex separation process of silica gel column chromatography. However, the process of silica gel column chromatograph is laborious and solvent-, time-, and cost-consuming. The disadvantages of low efficiency, low yield, and high cost of the preparation and separation limit the further applications of p-CDs. Metal ions, especially for transition metal ions, are toxic and hard to recycle in wastewater, surface water and ground water.20 Therefore, it is necessary to develop an efficient metal ions sensor.
Herein, we report a one-pot synthesis for red emitting C-dots from p-PD in the assistance of acids. Among the different inorganic acids, such as H3PO4, HNO3, and HF etc., HNO3 were found to be efficient to prepare red C-dots (labelled as N-CDs). These C-dots possess unique optical properties. The as-prepared N-CDs can be directly used as a sensor to detect metal ions, and they are sensitive for Fe3+ sensing at a range of 10–300 nmol L−1 with the limit of determination (LOD) of 1.9 nmol L−1.
2. Experimental section
2.1 Experimental materials
p-PD, hexane, and ethyl alcohol were purchased from Aladdin Co., China. The concentrated acids of HNO3, HF, H3PO4 were purchased from Sinopharm Chemical Reagent Co., China. KCl, CaCl2, Mg(NO3)2, Al(NO3)3, Zn(NO3)2, FeCl3, SnCl2, Pb(NO3)2, CuCl2, AgNO3, CoCl2, NiCl2, MnCl2, InCl3, Cd(NO3)2 and Cr(NO3)2, were purchased from Aladdin Ltd., China. All the reagents were used without purification. Deionized (DI) water was used in the whole experimental procedures.
2.2 Synthesis of CDs
Several parameters, such as different acids, molar ratio of c(acid)
:
c(p-PD), reaction temperature (T), reaction time (t), were concerned to optimize the experimental conditions. Firstly, mixture aqueous solutions of 0.1 mol L−1 p-PD and acid solution (HNO3, H3PO4 or HF) with controlled concentration of x mol L−1 were sealed into Teflon-lined autoclave, heated up to 200 °C inside a constant temperature oven keeping for 2 h, and then naturally cooled to room temperature. The c(acid) for the brightest and reddest C-dots under 365 nm UV light (PL assessment, see Fig. S1†) was optimized. Secondly, solutions with y mol L−1 p-PD and c(acid) acid were treated as the first step and then the optimal mole ratio was obtained by PL assessment. Thirdly, keeping the optimal mole ratio and other conditions constant and changing c(p-PD) from 0.02 to 0.20 mol L−1, then the optimal ratio was refined and the range of c(p-PD) for the preparation of red C-dots was obtained. At last, fixing the refined ratio and other conditions, the reaction temperature T was varied from 140 °C to 220 °C. Similarly, the reaction time t was also optimized ranging from 2 h to 8 h. The optimized experimental parameters, such as the molar ratio, T and t, were finally confirmed (see Fig. S2†). As a contrast, p-PD aqueous solution (0.1 mol L−1) without acid adding was hydrothermally reacted at 200 °C for 2 h. Samples prepared by p-PD aqueous solutions with HNO3, H3PO4, HF, and without acid adding, were labelled as N-CDs, P-CDs, F-CDs, and Pp-PD, respectively. For the purification (see Table S1†), the crude products of N-CDs were successively washed (by hexane to remove the unreacted p-PD), centrifuged (at 14
000 rpm for 30 min to remove polymer precipitation), and filtered (through a 0.22 μm filter membrane). The final concentration of the obtained N-CDs was determined by weighting the mass the dried powder of the corresponding C-dots solution sample.
2.3 Quantum yield measurements
Quantum yields (QYs) of the C-dots were determined by a relative measurement method.17,21 Specially, Rhodamine B (QYs = 56% in ethanol) was selected as the reference dye for the emission range of 580–610 nm excited by 365 nm UV light. The QYs was calculated by
where ϕ and ϕ′ are the QYs of sample and reference dye, I and I′ are the integrated emission intensity of the sample and dye, n and n′ are the refractive indexes (1.33 for H2O and 1.36 for ethanol) of sample and dye. A series of C-dots solution samples and the reference dye were prepared with proper concentrations to ensure the light absorbance A < 0.1 at 365 nm. The QYs values were then obtained by comparing the integrated PL intensities vs. absorbance curves.
2.4 Characterization
High-resolution TEM (HR-TEM) images were recorded on transmission microscope (JEM-2100HR, JEOL) operating at 200 kV. FT-IR spectra of C-dots solutions were collected using FT-IR spectrometer (IRPrestige-21, SHIMADZU) by use of KRS-5 window slices (mixture of TlBr and TlI). Typically, liquid phases were dropped on one slice and dried. The slice was covered by the other slice and fixed on the testing stand, then the infrared spectra recorded.
2.5 Optical measurements
The fluorescence spectra of C-dots were measured using a fluorescence spectrophotometer (F-2500, HITACHI). UV-Vis absorption spectra were recorded on using UV/VIS/NIR Spectrometer (Lambda 950, Perkin Elmer).
2.6 Detection of metal ions
The fluorescence intensity of the N-CDs sample is concentration dependent and reaches its highest value at 0.1 mg mL−1. Therefore, the concentration of C-dots for metal ions sensing is set up at 0.1 mg mL−1. The as-prepared N-CDs solution (9.6 mg mL−1, pH = 3) was diluted to 0.1 mg mL−1 by 0.1 mol L−1 HNO3 solution, and all the metal ion solutions were prepared and diluted step-wise with 0.1 mol L−1 HNO3 solution to the concentrations of interest. Herein, 0.1 mol L−1 HNO3 solution was used for two reasons. The one is intended to build a method of metal ions sensing with purification-free C-dots, and the other is to avoid the hydrolysis of some unstable ions, such as Sn2+, Mn2+, etc.
Selective sensing experiments for metal ions were carried out by fluorescence intensity quenching method. Typically, 200 μL of different metal ion solutions (1 μmol L−1) or blank sample (0.1 mol L−1 HNO3 solution) were added into 1800 μL N-CDs solutions (1 mg mL−1) to prepare a serious testing solutions (Mn+ = 100 nmol L−1, blank = 0 nmol L−1). After incubated at 30 °C for 30 min, fluorescence of all incubated solutions were measured at the same excitation wavelength of 510 nm.
For Fe3+ sensing experiments, 1800 μL N-CDs (0.1 mg mL−1) and 200 μL Fe3+ (0–10 μmol L−1) were incubated at 30 °C for 30 min prior to fluorescence measurements (excited at 510 nm).
3. Results and discussion
3.1 Red C-dots can be prepared from p-PD in acidic solutions
Massive experiments have been performed to optimize the experimental parameters, and the results show that the reproducibility of the proposed synthesis is very high. The best conditions for the preparation of red emitting C-dots are concluded and shown in Table 1. The molar ratio of c(acid)
:
c(p-PD) for HNO3-, H3PO4-, and HF-assisted synthesis are 2.5, 7, and 62.5, respectively. The best temperature (180–200 °C) and the reaction time (2 h) are similar. The QYs of N-CDs is the highest (15.8%) (see Table 1, Fig. S3†). In the HNO3-assisted synthesis, N-CDs emit blue light at the higher mole ratio range of 3.75 to 5 and red light at a lower range of 3 to 1.5 (see Fig. S2a†). When the ratio was fixed to 2.5, all the N-CDs emit red light at the full range (0.02–0.20 mol L−1) of c(p-PD) (see Fig. 1). It is worth noting that N-CDs emit turquoises and blue light when the reaction time was increased to 6 h and above (see Fig. S2a†). In the H3PO3-assisted synthesis, all as-prepared P-CDs emit weak red light and show no colour change (see Fig. S1b†). P-CDs are easy to be prepared at a lower temperature (140 °C). However, in general the PL intensities of P-CDs are all weak. In the HF-assisted synthesis, the preparation of F-CDs is acid-consuming and the PL is rich in colour. At the high ratio of 220–500 (see Fig. S2c†), the as-prepared F-CDs emit blue light. At the lower ratio range of 50–100, the F-CDs emit red light. All the as-prepared C-dots crude products are dark burgundy under day light and emit red under 365 nm UV light (see Fig. S2d†).
Table 1 The optimized reaction parameters of p-PD aqueous solution with different acid systems and the quantum yields of the C-dots aqueous solutions (10× diluted from as-prepared products)
C-dots |
Acid |
c(acid) (M) |
c(p-PD) (M) |
T (°C) |
t (h) |
QYs (%) |
F-CDs |
HF |
10 |
0.16 |
200 |
2 |
11.5 |
P-CDs |
H3PO4 |
0.7 |
0.10 |
180 |
2 |
11.7 |
N-CDs |
HNO3 |
0.35 |
0.14 |
200 |
2 |
15.8 |
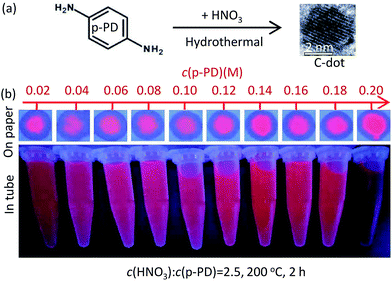 |
| Fig. 1 (a) The schematic diagram of N-CDs synthesis. (b) The photographs of PL spots of as-prepared N-CDs aqueous solutions dropped on filter paper (top row) and the N-CDs solutions (10× diluted from as-prepared products) in tubes (bottom row) under 365 nm UV light. | |
3.2 TEM characterization and FT-IR analysis
TEM images of Pp-PD and N-CDs were shown in Fig. 2. There were no C-dots but a large amount of polymer nanosheets formed in the p-PD aqueous solution without acid added (see Fig. 2a), while the red-emitting C-dots formed in the assistance of acids. N-CDs, which were prepared from p-PD with the assistance of HNO3, are uniform and monodisperse nanodots (see Fig. 2b) with an average diameter of 3.46 nm (see Fig. 2c). The C-dots exhibit identical well-resolved lattice fringes with a space of ∼0.21 nm, corresponding to the (100) in-plane lattice spacing of graphene.22,23 It indicates that the addition of acid can inhibit the polymerization process and promoted the formation of nanodots.
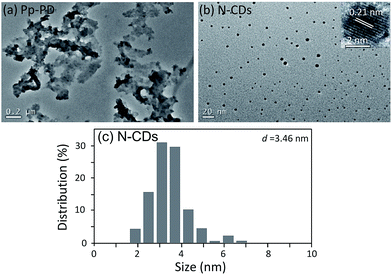 |
| Fig. 2 Reprehensive TEM image of the as-prepared Pp-PD (a) and N-CDs (b), the size distribution of N-CDs (c). The distribution was calculated from the TEM image in (b). N-CDs and Pp-PD were prepared by hydrothermal reaction from p-PD aqueous solution with and without HNO3 assistance. | |
Chemical groups on the surface of C-dots may impact the optical properties. From the FT-IR spectra of N-CDs (see Fig. 3), N-CDs contain many groups derived from the precursor, such as v(Ar–H) (2700–3200 cm−1, belonging to aromatic C–H stretching vibration24), δ(Ar–H) (800–850 cm−1, belonging to aromatic C–H out-of-plane deformation vibration of substituted benzene ring25), and δ(N–H) (1520 cm−1, belonging to the deformation vibration of –NH2 or –NH– groups). Other groups, such as v(O–H) (3500 cm−1, belonging to the deformation vibration of –OH groups in aliphatic alcohols), v(C
O) (1600 cm−1, belonging to the stretching vibration of carbonyl in carboxyl groups), and v(C–O–C) (1200 cm−1, belonging to the stretching vibration of ester groups), were formed by ring opening and oxidation reactions.
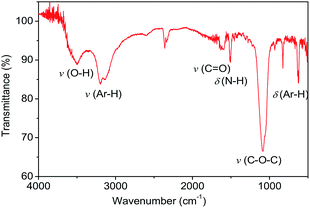 |
| Fig. 3 FT-IR spectra of N-CDs prepared from p-PD and HNO3 solution. | |
3.3 Optical properties
Although being prepared assisted with different acids, F-CDs, P-CDs and N-CDs showed similar optical properties (see Fig. 4). For UV-Vis spectra, the absorption peaks are located at around 290 and 510 nm, and the absorbance intensities are relatively strong at the range from UV to visible light zone (350–600 nm, see Fig. 4a). The maximum wavelengths of the absorption peaks at around 510 nm occurred red-shift in the order of F-CDs, P-CDs, and N-CDs, this is due to the gradually increasing of acidity and oxidation of acids that added in the precursors. For excitation, there are 4 obvious peaks (A: 260 nm, B: 430 nm, C: 510 nm and D: 580 nm) in N-CDs while several peaks are less prominent in F-CDs and P-CDs samples, and all CDs can be excited at a maximum wavelength of 580 nm (see Fig. 4b). It indicates that N-CDs have a broad and efficient excitation band in the visible region. For emission, being excited at the same wavelength (260 nm), F-CDs and P-CDs emit at 355 and 600 nm, while N-CDs emission slightly blue-shift (349 and 594 nm) and another significant peak is found at around 680 nm (see Fig. 4c). This 680 nm-peak can increase the intensity of red light emission for N-CDs. N-CDs is more chemically stable than P-CDs and F-CDs. It is worth noting that the emission is excitation-wavelength-independent (see Fig. 4d).26 This indicates that N-CDs are homogeneous C-dots and emit at a single dot level.27–29
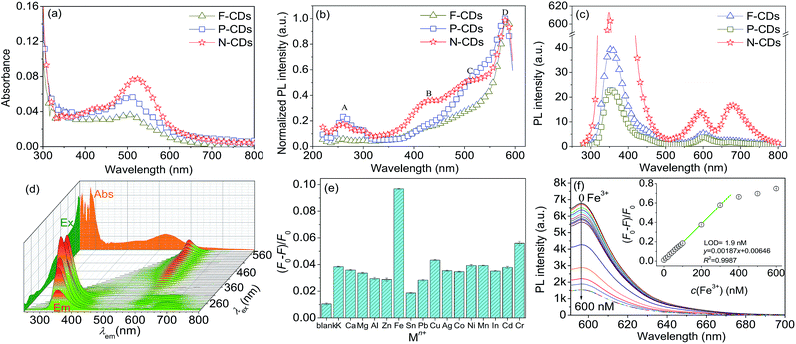 |
| Fig. 4 UV-Vis absorption (a), excitation (λem = 600 nm) (b) and emission (λex = 260 nm) spectra (c) of different C-dots aqueous solutions (10× diluted from as-prepared products), the emission spectra of N-CDs aqueous solution (10× diluted from the as-prepared product) with the λex = 260–580 nm (d), the selectivity (e) and the sensitivity (f) of N-CDs (0.1 mg mL−1) for the Fe3+ ions sensing in 0.1 mol L−1 HNO3 solutions. The concentrations of metal ions are all at 100 nmol L−1 (e), and the Fe3+ concentrations varied at 0, 10, 20, 30, 40, 50, 60, 70, 80, 90, 100, 200, 300, 400, 500, and 600 nmol L−1 (f). Fluorescence curves of all the samples were measured at the excitation wavelength of 510 nm. The inset in (f) is the linearity of PL intensity at 580 nm against the measured Fe3+ concentrations. | |
3.4 Selective Fe(III) sensing
The selectivity results of N-CDs for 16 kinds of different metal ions are shown in Fig. 4e. Among the tested metal ions, Fe3+ is relatively efficient to quench the PL of N-CDs. Fe3+ sensing experiments by using N-CDs probe were carried out as Fig. 4f. The quenching of N-CDs is linear at the range of 10–300 nmol L−1 of Fe3+, and the LOD is 1.9 nmol L−1.
C-dots were environmental friendly and potential alternative of fluorescent dyes in metal ions detection. The most prominent advantage is that the red-emitting C-dots can be excited at visible region (400–580 nm) and avoid the use of UV light.
4. Conclusions
p-Phenylenediamine (p-PD) aqueous solution can be transformed to C-dots (or poly(p-PD)) with (or without) acid assistance. Many common inorganic acid, such as HNO3, H3PO4 and HF, can efficiently help to synthesize red-emitting C-dots. The red-emission of p-PD-based C-dots can be enhanced by strong acid (such as HNO3). N-CDs, prepared with the assistance of HNO3, have a wide linear range with a lower LOD for Fe3+ sensing. The selectivity could be further improved for future applications. QYs and the selectivity are two key challenges for N-CDs applied in metal ions detection. Introducing the third reactants, which contain amino- and carboxylic-groups, will be our further research work. Red-emitting C-dots would be also very powerful in the biomedical optical imaging and sensing.
Conflicts of interest
There are no conflicts to declare.
Acknowledgements
This work was supported by the National Natural Science Foundation of China (61675071, 61405062, 61471186 and 91233208), the Pearl River Nova Program of Guangzhou (201710010010), the Guangdong Innovative Research Team Program (201001D00104799318, 2011D039), the Guangdong Natural Science Foundation of Guangdong Province (2014A030313445), the China Postdoctoral Science Foundation (2016M600659, XJ2015018 and 2014T70818), and Joint International Research Laboratory of Optical Information and the Key Laboratory of Optoelectronic Devices and Systems of ministry of Education.
References
- N. Durán, M. B. Simões, A. C. M. de Moraes, W. J. Fávaro and A. B. Seabra, J. Biomed. Nanotechnol., 2016, 12, 1323 CrossRef
. - T. S. Xu, X. Y. Li, Z. H. Xie, X. G. Li and H. Y. Zhang, Microchim. Acta, 2015, 182, 2541 CrossRef CAS
. - J. C. Ge, Q. Y. Jia, W. M. Liu, L. Guo, Q. Y. Liu, M. H. Lan, H. Y. Zhang, X. M. Meng and P. F. Wang, Adv. Mater., 2015, 27, 4169 CrossRef CAS PubMed
. - L. Bao, C. Liu, Z. L. Zhang and D. W. Pang, Adv. Mater., 2015, 27, 1663 CrossRef CAS PubMed
. - M. Vedamalai, A. P. Periasamy, C. W. Wang, Y. T. Tseng, L. C. Ho, C. C. Shih and H. T. Chang, Nanoscale, 2014, 6, 13119 RSC
. - S. L. Hu, Z. J. Wei, Q. Chang, A. Trinchi and J. L. Yang, Appl. Surf. Sci., 2016, 378, 402 CrossRef CAS
. - P. Mirtchev, E. J. Henderson, N. Soheilnia, C. M. Yip and G. A. Ozin, J. Mater. Chem., 2011, 22, 1265 RSC
. - X. Y. Xu, R. Ray, Y. L. Gu, H. J. Ploehn, L. Gearheart, K. Raker and W. A. Scrivens, J. Am. Chem. Soc., 2004, 126, 12736 CrossRef CAS PubMed
. - S. N. Baker and G. A. Baker, Angew. Chem., Int. Ed., 2010, 49, 6726 CrossRef CAS PubMed
. - S. J. Zhu, Y. B. Song, X. H. Zhao, J. R. Shao, J. H. Zhang and B. Yang, Nano Res., 2015, 8, 355 CrossRef CAS
. - J. Yu, N. Song, Y. K. Zhang, S. X. Zhong, A. J. Wang and J. R. Chen, Sens. Actuators, B, 2015, 214, 29 CrossRef CAS
. - Y. Liu, Y. N. Liu, M. Park, S. J. Park, Y. F. Zhang, M. R. Akanda, B. Y. Park and H. Y. Kim, Carbon Lett., 2017, 21, 61 CrossRef
. - B. S. B. Kasibabu, S. L. D'souza, S. Jha and S. K. Kailasa, J. Fluoresc., 2015, 25, 803 CrossRef CAS PubMed
. - V. N. Mehta, S. Jha and S. K. Kailasa, Mater. Sci. Eng., C, 2014, 38, 20 CrossRef CAS PubMed
. - H. Zhang, Y. Wang, D. Wang, Y. B. Li, P. Liu, H. G. Yang, T. C. An, Z. Y. Tang and H. J. Zhao, Small, 2014, 10, 3371 CrossRef CAS PubMed
. - Y. W. Zheng, D. Yang, X. Wu, H. R. Yan, Y. C. Zhao, B. Feng, K. Duan, J. Weng and J. X. Wang, RSC Adv., 2015, 5, 90245 RSC
. - K. Jiang, S. Sun, L. Zhang, Y. Lu, A. G. Wu, C. Z. Cai and H. W. Lin, Angew. Chem., Int. Ed., 2015, 54, 5360 CrossRef CAS PubMed
. - L. Song, Y. Y. Cui, C. F. Zhang, Z. B. Hu and X. F. Liu, RSC Adv., 2016, 6, 17704 RSC
. - H. Ding, S. B. Yu, J. S. Wei and H. M. Xiong, ACS Nano, 2016, 10, 484 CrossRef CAS PubMed
. - P. Wang, P. Y. Xiao, S. X. Zhong, J. R. Chen, H. J. Lin and X. L. Wu, J. Mater. Chem. A, 2016, 4, 15450 CAS
. - M. Grabolle, M. Spieles, V. Lesnyak, N. Gaponik, A. Eychmüller and U. Reschgenger, Anal. Chem., 2009, 81, 6285 CrossRef CAS
. - S. Qu, D. Zhou, D. Li, W. Ji, P. Jing, D. Han, L. Liu, H. Zeng and D. Shen, Adv. Mater., 2016, 28, 3516 CrossRef CAS PubMed
. - L. Tang, R. Ji, X. Li, G. Bai, C. P. Liu, J. Hao, J. Lin, H. Jiang, K. S. Teng and Z. Yang, ACS Nano, 2014, 8, 6312 CrossRef CAS PubMed
. - Z. Gao, Z. Lin, X. Chen, H. Zhong and Z. Huang, Anal. Methods, 2016, 8, 2297 RSC
. - A. M. Craciun, A. Diac, M. Focsan, C. Socaci, K. Magyari, D. Maniu, I. Mihalache, L. M. Veca, S. Astilean and A. Terec, RSC Adv., 2016, 6, 56944 RSC
. - Z. H. Wen and X. B. Yin, RSC Adv., 2016, 6, 27829 RSC
. - S. Ghosh, A. M. Chizhik, N. Karedla, M. O. Dekaliuk, I. Gregor, H. Schuhmann, M. Seibt, K. Bodensiek, I. A. T. Schaap, O. Schulz, A. P. Demchenko, J. Enderlein and A. I. Chizhik, Nano Lett., 2014, 14, 5656 CrossRef CAS PubMed
. - V. Strauss, J. T. Margraf, C. Dolle, B. Butz, T. J. Nacken, J. Walter, W. Bauer, W. Peukert, E. Spiecker, T. Clark and D. M. Guldi, J. Am. Chem. Soc., 2014, 136, 17308 CrossRef CAS PubMed
. - S. K. Das, Y. Liu, S. Yeom, D. Y. Kim and C. I. Richards, Nano Lett., 2014, 14, 620 CrossRef CAS PubMed
.
Footnote |
† Electronic supplementary information (ESI) available. See DOI: 10.1039/c7ra06223k |
|
This journal is © The Royal Society of Chemistry 2017 |
Click here to see how this site uses Cookies. View our privacy policy here.