DOI:
10.1039/C7RA06300H
(Paper)
RSC Adv., 2017,
7, 35814-35818
Highly selective detection of nitrotoluene based on novel lanthanide-containing ionic liquids†
Received
6th June 2017
, Accepted 10th July 2017
First published on 19th July 2017
Abstract
Two novel fluorescent ionic liquids based on europium and dysprosium, [MOEMIm][Ln(NO3)4] (Ln = Eu, Dy), were synthesized. They exhibited good fluorescence properties under UV-light irradiation, and the phosphor presented bright red (Eu3+) and light blue (Dy3+) luminescence. This is the first time that the application of lanthanide-based room temperature ionic liquids has been investigated in the field of fluorescence analysis for aromatic compounds. As a fluorescence-quenching sensor for o-(m-, p-)nitrotoluene, trace amounts of the quenchers caused the fluorescence intensity to decrease and produced significant fluorescence quenching. Additionally, p-nitrotoluene gave the most significant fluorescence quenching effect among the three isomeric nitrotoluenes; also, the quenching coefficient KSV was calculated using the Stern–Volmer equation. What's more, the two fluorescent ionic liquids demonstrated high selectivity toward nitrotoluene even in the presence of methylbenzene, phenol, chlorobenzene, and aminobenzene. Hence, the selective recognition of nitrotoluene from other aromatic compounds may be used for the analytical detection of nitrotoluene.
Introduction
Ionic liquids (ILs) have attracted much attention from both the industrial and academic communities due to the nature of these liquid soft materials.1 Recently, IL-containing systems have been widely studied and different nanostructures in IL-containing systems have been obtained, such as micelles,2 emulsions,3 microemulsions,4 gels,5,6 liquid crystals and vesicles.7–9 In recent years, ILs have also been discovered that exhibit fluorescence, and can therefore be applied in the determination of mercury ions,10 picric acid,11 and others,12–14 which indicates that they are promising for fluorescence detection.
Nitrotoluene compounds are very important organic chemical materials and intermediates, which are widely applied in the pharmaceutical, pesticide and dye industries.15 However, pollution due to nitrotoluene causes adverse effects in industrial wastewaters and consequently on human health.16 As multiple aromatic compounds exist in industrial wastewaters, among them, nitrotoluene, as a kind of toxic and non-degradable material, is hard to detect in industry, so methods for its detection have received growing interest. Current detection methods for nitrotoluene typically include gas chromatography,17 gas chromatography coupled with mass spectrometry,18 reversed-phase high-performance liquid chromatography,19 surface-enhanced Raman spectroscopy,20 thermal neutron analysis,21 ion mobility spectroscopy22 and fluorescence sensing.23–26 Among these technologies, the fluorescence quenching method has received considerable attention due to its simplicity, high sensitivity, high selectivity and fast response times.16,27,28
As a continuation of our previous work,29 we report, for the first time, the successful synthesis of two novel fluorescent ILs containing Eu(III) and Dy(III) with a 1-(2-methoxyethyl)-3-methylimidazolium cation, which exhibit enhanced fluorescence. Interestingly, the fluorescence intensity of the two ILs can be influenced strongly by trace amounts of nitrotoluene, which produces significant fluorescence quenching. This phenomenon is not affected by the presence of possible interferents such as other aromatic compounds. Thus, the reported ionic liquids may have great potential for application in the analytical detection of nitrotoluene.
Materials and methods
Materials
N-Methylimidazolium, (2-methoxy)bromoethane, nitrogen, silver nitrate (AgNO3), acetonitrile (ACN), dimethyl sulfoxide (DMSO), methanol (MeOH) and ethanol (EtOH) were acquired at analytical reagent grade and used without further purification. They were purchased from Shanghai Chemical Reagent Co. Ltd. Dy(NO3)3·6H2O and Eu(NO3)3·6H2O were purchased from Beijing HWRK Chem Co. Ltd and used without any further purification.
Synthesis of [MOEMIm][Ln(NO3)4] (Ln = Eu, Dy)
A mixture of N-methylimidazolium (0.18 mol) and ether (50 mL) was stirred at room temperature under a nitrogen atmosphere; then, (2-methoxy)bromoethane (0.18 mol) was added via a syringe, and the reaction mixture was stirred for 18 h. The product, 1-(2-methoxyethyl)-3-methylimidazolium bromide [MOEMIm]Br (white solid), was washed with diethyl ether three times and dried in vacuo for 2 days at 353 K. Then 1-(2-methoxyethyl)-3-methylimidazolium nitrate ([MOEMIm]NO3) was synthesized from [MOEMIm]Br and AgNO3 in methanol ([MOEMIm]Br
:
AgNO3 = 1
:
1), and the reaction mixture was stirred for 1 h at room temperature in the absence of light. The product, [MOEMIm]NO3, was prepared through filtration and evaporation in turn. Subsequently, [MOEMIm]NO3 (9 mmol) was added to a mixture of Dy(NO3)3·6H2O or Eu(NO3)3·6H2O (9 mmol) and acetonitrile (80 mL) at room temperature, and the mixture was stirred vigorously for 2 h at 353.15 K. After completion of the reaction, the reaction mixture was cooled to room temperature, and the water and acetonitrile were vaporized under a reduced pressure of 1.0 ± 0.1 kPa at a temperature of 323–333 K. The products, [MOEMIm][Ln(NO3)4] (Ln = Eu, Dy), were dried in vacuo for 2 days at 338 K. The reaction scheme is shown in Fig. 1 and the structures of the products were characterized. For [MOEMIm][Eu(NO3)4], 1H NMR (600 MHz, DMSO, δ): 9.15 (s, 1H, N–CH
N), 7.71 (s, 1H, CH
CH), 7.75 (s, 1H, CH
CH), 4.36 (t, 2H, N–CH2), 3.88 (s, 3H, N–CH3), 3.68 (t, 2H, O–CH2), 3.27 (s, 3H, O–CH3); elemental analysis (%) calcd: C, 15.53; H, 2.42; N, 15.53, found: C, 15.31; H, 2.70; N, 15.51; ESI-MS: m/z [M+]: 141.10, [M−]: 401.23. For [MOEMIm][Dy(NO3)4], 1H NMR (600 MHz, DMSO, δ): 9.18 (s, 1H, N–CH
N), 7.80 (s, 1H, CH
CH), 7.76 (s, 1H, CH
CH), 4.41 (t, 2H, N–CH2), 3.92 (s, 3H, N–CH3), 3.74 (t, 2H, O–CH2), 3.30 (s, 3H, O–CH3); elemental analysis (%) calcd: C, 15.24; H, 2.38; N, 15.23, found: C, 15.16; H, 2.41; N, 15.19; ESI-MS: m/z [M+]: 141.08, [M−]: 410.92, no impurity peaks were found (Fig. S1–S7 ESI†).
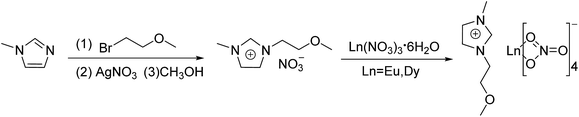 |
| Fig. 1 The reaction scheme of [MOEMIm][Ln(NO3)4] (Ln = Eu, Dy). | |
Fluorescence measurements
To examine the potential of the samples for sensing aromatic compounds, their absorption was measured using a Cary Eclipse Fluorescence Spectrophotometer (Varian) in a quartz cuvette of 10 mm optical path length. For the measurements, the suspension containing Eu(III) was excited at λex = 394 nm, and the suspension containing Dy(III) was excited at λex = 351 nm. The corresponding emission wavelengths were narrow peaks and were monitored from 500 to 650 nm and 450 to 770 nm, respectively. All of the corresponding fluorescence emission spectra were recorded at room temperature.
Results and discussion
Optical properties
The rare earth ILs [MOEMIm][Ln(NO3)4] (Ln = Eu, Dy) displayed excellent fluorescence behaviors under UV light irradiation. We first examined the fluorescence properties of the two ILs dispersed in dimethyl sulfoxide (DMSO) and other common solvents (acetonitrile (ACN), methanol (MeOH), ethanol (EtOH) and water) at the same concentration (1 M) at room temperature. The fluorescence intensity had nothing to do with the polarity of solvents, and it was the weakest in aqueous solution among the five solvents (Fig. S8 and S9 ESI†). Eventually, due to it combining a strong emission spectrum with the ability to dissolve aromatic compounds, DMSO was selected to be utilized as the final solvent. For Eu(III), Fig. 2a shows typical intense transitions in the emission spectrum at 538, 560, 593, and 617 nm with excitation at 394 nm for [MOEMIm][Eu(NO3)4] (0.5 M), with the representative derived peaks corresponding to 5D0–7F1, 5D0–7F2, 5D0–7F3, and 5D0–7F4 transitions. Upon UV-light irradiation, the phosphor presents bright red luminescence. For Dy(III) (0.1 M), the emission spectrum (λex = 351 nm) showed peaks at 480, 574, 655, and 751 nm in Fig. 2b, and it presents blue luminescence upon UV-light irradiation, corresponding to the intense transitions of 4F9/2–6H15/2, 4F9/2–6H13/2, 4F9/2–6H11/2, and 4F9/2–6H9/2.
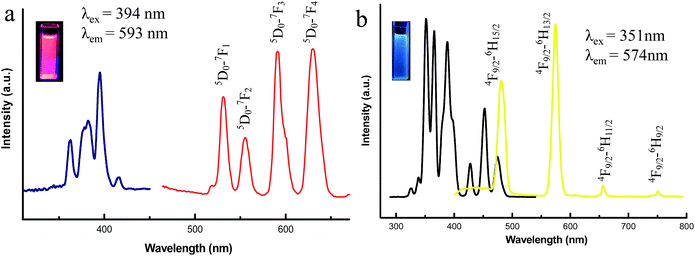 |
| Fig. 2 Excitation and emission spectra of the ILs: (a) [MOEMIm][Eu(NO3)4] and (b) [MOEMIm][Dy(NO3)4]. | |
Fluorescence properties of nitrotoluene
Different solutions of nitrotoluene were separately added to the prepared mixtures of ILs [MOEMIm][Ln(NO3)4] (Ln = Eu, Dy) and DMSO. The fluorescence measurement results showed that the emission spectrum of [MOEMIm][Ln(NO3)4] (Ln = Eu, Dy) was unchanged in shape with increasing concentration of the quencher, and even a low concentration of nitrotoluene can easily cause fluorescence quenching. This may be caused by the effective interactions with the electron-withdrawing group of nitrotoluene (–NO2), which lead to electron transfer between the ILs and the nitrotoluene, consequently leading to significant fluorescence quenching.23 The response behavior towards nitrotoluene is shown in Fig. 3 and 4. Both figures show a process of fluorescence quenching and the fluorescence intensity decreases with an increase in the nitrotoluene concentration. Moreover, p-nitrotoluene gave a more significant fluorescence quenching effect than o-(m-)nitrotoluene, with the order of the quenching performance being as follows: p-nitrotoluene > o-nitrotoluene > m-nitrotoluene. This is the same result as that reported by Wang et al.23 When [MOEMIm][Ln(NO3)4] (Ln = Eu, Dy) was exposed to various concentrations of nitrotoluene as a quencher, the dependence of the fluorescence signal in response to nitrotoluene can be described by the Stern–Volmer equation:
where I0 is the initial fluorescence intensity, I is the fluorescence intensity measured in the presence of the analyte, [Q] is the concentration of the quencher and KSV is the Stern–Volmer constant. A linear Stern–Volmer behavior was obtained (Fig. S10 and S11 ESI†) for the lower concentration of nitrotoluene, where the quenching is dominated by a dynamic process. The KSV values upon the addition of o-(m-, p-)nitrotoluene were calculated and are shown in Fig. 3 and 4, respectively. The detection limit (DL) of p-nitrotoluene was calculated by using the equation 3σ/K, where σ denotes the standard deviation of the quenching efficiency, and K represents the slope of the equation. The values of DL were 0.14 μM for [MOEMIm][Eu(NO3)4] and 0.15 μM for [MOEMIm][Dy(NO3)4] (Fig. S12 and S13 ESI†). In addition, for [MOEMIm][Eu(NO3)4], when the concentration of p-nitrotoluene was 6.5 μM, the quenching efficiency was above 92%, which was calculated by using the formula [(F0 − F)/F0] × 100% (F0 is the initial fluorescence intensity). Similarly, other quenching efficiencies at certain concentrations were also obtained. The changes in fluorescence intensity are shown in Fig. 3 and 4, and the corresponding histograms and color change processes are shown in Fig. S14 and S15.†
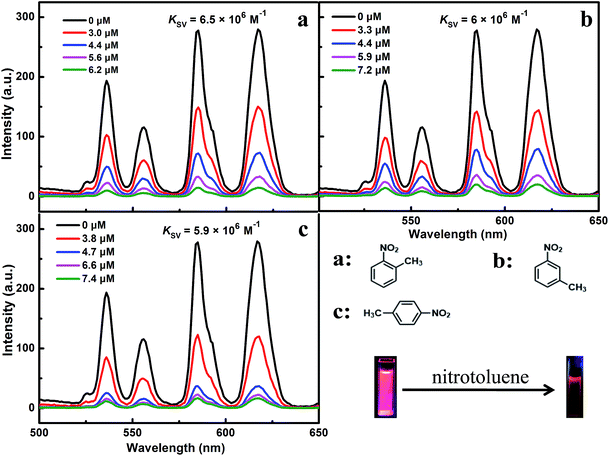 |
| Fig. 3 The photoluminescence response of [MOEMIm][Eu(NO3)4] upon the addition of o-(m-, p-)nitrotoluene. | |
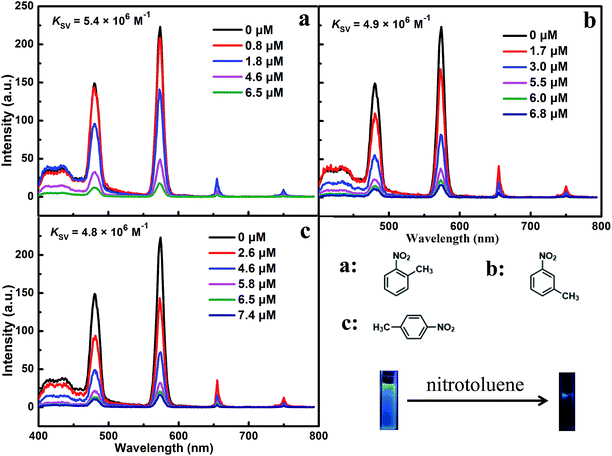 |
| Fig. 4 The photoluminescence response of [MOEMIm][Dy(NO3)4] upon the addition of o-(m-, p-)nitrotoluene. | |
As can be seen in Fig. 3 and 4, the color of the luminescence images in the process of fluorescence quenching varies from bright red or blue to black under UV light (λ = 365 nm), which demonstrates that the fluorescence quenching behavior can also be detected by visual observation.
Fluorescence detection of aromatic compounds
To investigate the sensing selectivity toward nitrotoluene, the possible interference from some other relevant substances (methylbenzene, phenol, chlorobenzene and aminobenzene) was investigated. The two samples were first dissolved in DMSO (1 M) containing methylbenzene, phenol, aminobenzene, chlorobenzene and p-nitrotoluene, respectively. As can be seen in Fig. 5 and 6, the fluorescence intensity remained almost unchanged with the addition of methylbenzene, phenol, chlorobenzene and aminobenzene, but significantly decreased in the presence of nitrotoluene. This indicates that the fluorescence intensity is not influenced by the former four compounds, but is obviously quenched by p-nitrotoluene. To further investigate the high selectivity for p-nitrotoluene over other aromatic compounds, a mixture containing methylbenzene, phenol, aminobenzene, chlorobenzene and p-nitrotoluene was prepared and added to the Dy(III) or Eu(III)-based ionic liquid in DMSO. Under the irradiation of UV light at 365 nm, the test sample showed a difference in color compared with the original one that was visible to the naked eye. The measurement of the emission spectrum indicated that the selectivity for nitrotoluene was not affected by the existence of other common aromatic compounds.
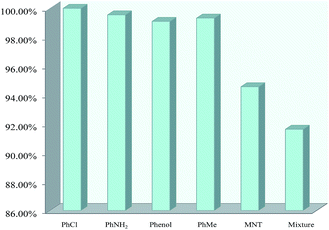 |
| Fig. 5 The fluorescence changes of [MOEMIm][Eu(NO3)4] with different aromatic compounds. | |
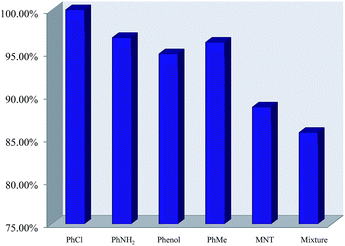 |
| Fig. 6 The fluorescence changes of [MOEMIm][Dy(NO3)4] with different aromatic compounds. | |
Conclusions
Two novel fluorescent ionic liquids based on europium and dysprosium, [MOEMIm][Ln(NO3)4] (Ln = Eu, Dy), were synthesized, and exhibit good fluorescence properties under UV-light irradiation, with the phosphor presenting bright red (Eu3+) and light blue (Dy3+) luminescence. Furthermore, we investigated the application of the lanthanide-based room temperature ionic liquids in the field of fluorescence analysis. When trace amounts of o-(m-, p-)nitrotoluene were added, the fluorescence intensity decreased and significant fluorescence quenching was observed. Among the three isomeric nitrotoluenes, p-nitrotoluene gave the most significant fluorescence quenching effect compared to that of o-(m-)nitrotoluene. Moreover, the two fluorescent ionic liquids demonstrated high selectivity toward nitrotoluene, even in the presence of aromatic compounds. Therefore, the selective recognition of nitrotoluene over other aromatic compounds may be used for explosives detection.
Acknowledgements
The project was supported by the National Natural Science Foundation of China (21673107) and LNET (LR2015025).
Notes and references
- Z. Y. Yan and B. Yan, New J. Chem., 2014, 38, 2604–2610 RSC.
- M. Pal, K. Behera and S. Pandey, Colloids Surf., A, 2016, 507, 227–235 CrossRef CAS.
- G. S. Medina and M. Reta, J. Sep. Sci., 2016, 39, 4209–4218 CrossRef CAS PubMed.
- K. Damarla, P. Bharmoria, K. S. Rao, P. S. Gehlot and A. Kumar, Chem. Commun., 2016, 52, 6320–6323 RSC.
- M. Baloch, A. Vizintin, R. K. Chellappan, J. Moskon, D. Shanmukaraj, R. Dedryvee're, T. Rojo and R. Dominko, J. Electrochem. Soc., 2016, 163, 2390–2398 CrossRef.
- C. C. Hall, C. Zhou, S. P. O. Danielsen and T. P. Lodge, Macromolecules, 2016, 49, 2298–2306 CrossRef CAS.
- G. Saielli and Y. Wang, J. Phys. Chem. B, 2016, 120, 9152–9160 CrossRef CAS PubMed.
- C. Banerjee, A. Roy, N. Kundu, D. Banik and N. Sarkar, Phys. Chem. Chem. Phys., 2016, 18, 14520–14530 RSC.
- S. Wang, X. L. Yin, Y. Yan, Z. Y. Xiang, P. Liu, Y. Chen, X. Xin and Y. Z. Yang, Ind. Eng. Chem. Res., 2016, 55, 8207–8214 CrossRef CAS.
- X. D. Wen, Y. Gao, P. Wu, Z. Q. Tan, C. B. Zheng and X. D. Hou, J. Anal. At. Spectrom., 2016, 31, 415–422 RSC.
- X. Tian, X. J. Qi, X. Y. Liu and Q. H. Zhang, Sens. Actuators, B, 2016, 229, 520–527 CrossRef CAS.
- H. T. Temiz, U. Tamer, A. Berkkan and I. H. Boyaci, Talanta, 2017, 167, 557–562 CrossRef CAS PubMed.
- J. Shen, C. Sun and X. Wu, Talanta, 2017, 165, 369–376 CrossRef CAS PubMed.
- X. Deng and D. Wu, RSC Adv., 2014, 4, 42066–42070 RSC.
- M. He, Y. Sun, X. Li and Z. Yang, Chemosphere, 2006, 65, 365–374 CrossRef CAS PubMed.
- K. Volkan Özdokur, L. Pelit, H. Ertaş, S. Timur and F. N. Ertaş, Talanta, 2012, 98, 34–39 CrossRef PubMed.
- B. S. Crimmins and J. E. Baker, Atmos. Environ., 2006, 40, 6764–6779 CrossRef CAS.
- K. Schoene, H. J. Bruckert, J. Steinhanses and A. Koenig, Fresenius. J. Anal. Chem., 1994, 348, 364–370 CrossRef CAS.
- K. Kalikova, R. Geryk, J. Vozka and E. Tesarova, J. Sep. Sci., 2015, 38, 711–719 CrossRef CAS PubMed.
- M. M. Liu and W. Chen, Biosens. Bioelectron., 2013, 46, 68–73 CrossRef CAS PubMed.
- G. Vourvopoulos and P. C. Womble, Talanta, 2001, 54, 459–468 CrossRef CAS PubMed.
- J. M. Perr, K. G. Furton and J. R. Almirall, J. Sep. Sci., 2005, 28, 177–183 CrossRef CAS PubMed.
- F. Q. Wang, Z. C. Yu, C. M. Wang, K. H. Xu, J. G. Yu, J. X. Zhang, Y. Y. Fu, X. Y. Li and Y. N. Zhao, Sens. Actuators, B, 2017, 239, 688–695 CrossRef CAS.
- B. Xing, H. Y. Li, Y. Zhu, Z. Zhao, Z. G. Sun, D. Yang and J. Li, RSC Adv., 2016, 6, 110255–110265 RSC.
- D. Wang, L. Sun, C. Hao, Y. Yan and Z. Liang, RSC Adv., 2016, 6, 57828–57834 RSC.
- S. N. Zhao, X. Z. Song, M. Zhu, X. Meng, L. L. Wu, S. Y. Song, C. Wang and H. Zhang, RSC Adv., 2015, 5, 93–98 RSC.
- T. M. Geng, S. N. Ye, W. Yu, H. Zhu, X. Wang and X. Liu, Talanta, 2017, 165, 282–288 CrossRef CAS PubMed.
- N. Ferretto, M. Tedetti, C. Guigue, S. Mounier, R. Redon and M. Goutx, Chemosphere, 2014, 107, 344–353 CrossRef CAS PubMed.
- B. H. Fan, J. Wei, X. X. Ma, X. X. Bu, N. N. Xing, Y. Pan, L. Zheng and W. Guan, Ind. Eng. Chem. Res., 2016, 55, 2267–2271 CrossRef CAS.
Footnote |
† Electronic supplementary information (ESI) available. See DOI: 10.1039/c7ra06300h |
|
This journal is © The Royal Society of Chemistry 2017 |
Click here to see how this site uses Cookies. View our privacy policy here.