DOI:
10.1039/C7RA06466G
(Paper)
RSC Adv., 2017,
7, 40067-40073
Synthesis and evaluation of the antitumor activity of highly functionalised pyridin-2-ones and pyrimidin-4-ones†
Received
9th June 2017
, Accepted 9th August 2017
First published on 16th August 2017
Abstract
The methods for the synthesis of two novel types of compounds, including pyridin-2-ones 3 and pyrimidin-4-ones 4 were developed. Pyridin-2-ones 3 were synthesised via the regioselective reaction of N,N′-disubstituted 1,1-ene diamines 1a–1w with mercaptals 2a–2c in acetonitrile promoted by Cs2CO3 under refluxing conditions. Fortunately, pyrimidin-4-ones 4 were obtained when the N-monosubstituted 1,1-ene diamines 1x–1b′, used as substrate, by accident, reacted with mercaptals 2 under similar conditions. As a result, two kinds of novel heterocycles were synthesised by this protocol. The reactions have some advantages, such as excellent yield, inexpensive raw materials and convenient final treatment. The antitumor bioactivity screening showed that certain compounds had potent antitumor activity. Especially, compounds 3r, which showed the most potent activity with IC50 values lower than 12.3 μmol L−1 against four human tumor cell lines, making it more active than cisplatin (DDP). In addition, a preliminary assessment of the structure–selectivity relationship of the compounds was also performed.
Introduction
Pyridin-2-one derivatives are important N-containing heterocycles with a broad range of biological activities, including antitumor (Fig. 1, A),1 antibacterial,2 antianxiety (Fig. 1, JNJ40411813),3 anti-HIV,4–9 anti-inflammatory,10 anti-HBV,11 antituberculosis,12 antithrombus,13 non-steroidal steroid alpha reductase and phosphodiesterase inhibitory activities,14,15 etc. Additionally, they are commonly used as medicinal or pesticidal intermediates. Pyridin-2-ones are widely distributed in natural products, such as tenellin, funiculosin and ilicicolin H, which are a new type of natural alkaloid.16 To date, pyridin-2-ones have been studied by medical and chemical scientists. Various methods for the synthesis of this compound have been reported,17–19 including [1 + 2 + 3] cyclization, [3 + 3] cyclization, rearrangement process, etc. The synthesis of pyridin-2-ones have made important contributions to the development of pyridin-2-ones compounds and their application. However, some of the existing synthesis methods have certain limitations, such as the use of high temperature, strong acid, metal catalyst or multiple steps. To meet the demands of drug discovery and screening, a concise and efficient one-pot parallel synthesis is very desirable.
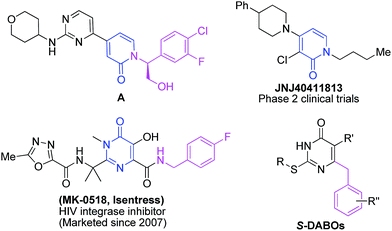 |
| Fig. 1 Biological activity pyridin-2-ones & pyrimidin-4-ones. | |
The pyrimidin-4-one also has various biological activities and is widely used as an inhibitor of the enzyme reverse transcriptase to develop anti-HIV drugs, such as MK0518 and dihydro-alkylthio-benzyl-oxopyrimidines (S-DABOs) (Fig. 1),20,21 tenofovir, dapivirine, MIV150, UC781, UAMC01398, and DABO.22–26 In addition, it is also used in the development of various other drugs, including anti-schizophrenia,27 and endothelial cell dysfunction inhibitors,28 phosphoinositide 3-kinase inhibitors,29 CXCR3 antagonists,30 etc.31–33 Accordingly, various pyrimidin-4-ones have been obtained by many groups.34
Our group has been applying the one-step strategy to construct drug-like N-containing heterocycles for many years.35–37 One-step strategies usually have some advantages over other methods, such as excellent yield, inexpensive raw materials and convenient final treatment, which reduce the production cost and avoid or reduce the environmental pollution.
1,1-Ene Diamines (EDAMs) serve as important and useful building blocks to construct various fused heterocyclic compounds including pyridines,38 1,4-dihydropyridine,39 pyridin-2-ones,40 indoles, isoquinolinone, etc.,41,42 have a broad range of biological activities.43 The novel properties of the chemical reaction of EDAMs, which serve as diversity building blocks, need to be explored in order to further widely use these blocks for the synthesis of heterocycles with potential biological activity to meet the demands of high activity screen.
In this paper, pyridin-2-ones 3 are synthesised by a one-step cascade reaction of N,N′-disubstituted 1,1-ene diamine (DEDAM) 1 with 2, which was promoted by Cs2CO3. Pyrimidin-4-ones 4 are also prepared based on the cascade reaction of the N-monosubstituted 1,1-ene diamine (MEDAM) 1 with 2 under similar conditions. As a result, the target compounds 3–4 are obtained with medium to good yields (83–98%). The reaction has good substrate adaptability (aromatic ring, aromatic heterocyclic, alkyl), and the target product has the characteristics of molecular diversity (R = Ar, Alk).
Results and discussion
First, N,N′-disubstituted 1,1-ene diamine (DEDAM) 1a is used as substrate and is reacted with ethyl 2-cyano-3,3-bis(methylthio)-acrylate 2a in 1,4-dioxane at reflux for 8 hours and we obtained the target compound 3a with very low yield (10%). Then, different solvents including 1,4-dioxane, ethanol, tetrahydrofuran (THF), N,N-dimethylformamide (DMF) and acetonitrile are assessed at reflux (Table 1, entries 1–5). The results showed that the best solvent is acetonitrile and we obtained the target compound 3a with 40% yield. Based on the optimal solvent, we further evaluated the alkali, such as Et3N, K2CO3, Cs2CO3, KOBu-t (Table 1, entries 6–9). The results demonstrated that Cs2CO3 can promote the reaction and largely increase the yield and we ultimately obtained the product with a good yield (89%). Finally, the reaction times were tested (Table 1, entries 8 vs. 10–11). The results revealed that the optimal reaction time is about 8 hours. Accordingly, we conclude that the optimal conditions are acetonitrile as solvent and Cs2CO3 as a base at reflux of 8 hours.
Table 1 Optimism conditions for synthesis of pyridin-2-one 3aa
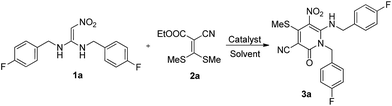
|
Entry |
Solvent |
Base |
t [°C] |
Time [h] |
Yieldb [%] |
Reagents and conditions: N,N′-disubstituted 1,1-ene diamine (DEDAM) 1a (1.0 mmol), mercaptal 2a (1.0 mmol), base (2.0 mmol) and solvent (15.0 mL). Isolated yield based on 1a. N.R. = no reaction. |
1 |
1,4-Dioxane |
— |
Reflux |
8 |
10 |
2 |
EtOH |
— |
Reflux |
8 |
N.R. |
3 |
THF |
— |
Reflux |
8 |
N.R. |
4 |
DMF |
— |
Reflux |
8 |
15 |
5 |
CH3CN |
— |
Reflux |
8 |
40 |
6 |
CH3CN |
Et3N |
Reflux |
8 |
65 |
7 |
CH3CN |
K2CO3 |
Reflux |
8 |
75 |
8 |
CH3CN |
Cs2CO3 |
Reflux |
8 |
89 |
9 |
CH3CN |
t-BuOK |
Reflux |
8 |
70 |
10 |
CH3CN |
Cs2CO3 |
Reflux |
4 |
68 |
11 |
CH3CN |
Cs2CO3 |
Reflux |
12 |
87 |
To expand the scope and application of this protocol, DEDAMs (n = 1, 2, 3, 4) bearing different aromatic groups, including p-CF3C6H4, p-FC6H4, p-ClC6H4, C6H5, p-MeC6H4, p-MeOC6H4, m-CF3C6H4, o-FC6H4, m-FC6H4, m-ClC6H4, p-BrC6H4, alkyl, etc., were used as substrate and reacted with mercaptals 2a–2c. Ultimately, a series of pyridin-2-one derivatives 3a–3y were prepared by this method (Table 2, entries 1–25). The yields of the products reveal that the group of DEDAMs have a slight influence on the yields (Table 2, entries 1–10). DEDAMs 1 with electron-withdrawing groups (F, Cl) often can obtain higher yields than those with electron-donating group of DEDAMs (MeO, Me) (Table 2, entries 1–3 & 7–9 vs. 5–6; 11–16 vs. 19). Longer chain DEDAMs (n = 2) produce the target compounds with higher yields (Table 2, 2 vs. 12; 3 vs. 15; 4–5 vs. 18–19) than those of the others. The longest chain DEDAMs (n = 3) gave the product with lowest yields compared with other DEDAMs (n = 1 or 2) (Table 2, 4 & 18 vs. 24).
Table 2 Preparation of pyridin-2-ones 3a–3ya
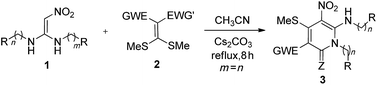
|
Entry |
1/R |
EWG |
EWG′ |
n |
Z |
Pr |
Yieldb [%] |
Reagents and conditions: N,N′-disubstituted 1,1-ene diamines (DEDAMs) 1 (1.0 mmol), mercaptals 2 (1.0 mmol), Cs2CO3 (2.0 mmol) and CH3CN (15.0 mL). Isolated yield based on DEDAMs 1. |
1 |
1a/p-CF3C6H4 |
CN |
COOEt |
1 |
O |
3a |
92 |
2 |
1b/p-FC6H4 |
CN |
COOEt |
1 |
O |
3b |
89 |
3 |
1c/p-ClC6H4 |
CN |
COOEt |
1 |
O |
3c |
87 |
4 |
1d/C6H5 |
CN |
COOEt |
1 |
O |
3d |
92 |
5 |
1e/p-MeC6H4 |
CN |
COOEt |
1 |
O |
3e |
85 |
6 |
1f/p-MeOC6H4 |
CN |
COOEt |
1 |
O |
3f |
84 |
7 |
1g/3,4-F2C6H3 |
CN |
COOEt |
1 |
O |
3g |
94 |
8 |
1h/2,4-F2C6H3 |
CN |
COOEt |
1 |
O |
3h |
96 |
9 |
1i/2,4-Cl2C6H3 |
CN |
COOEt |
1 |
O |
3i |
90 |
10 |
1d/C6H5 |
CN |
CN |
1 |
NH |
3j |
83 |
11 |
1j/m-CF3C6H4 |
CN |
COOEt |
2 |
O |
3k |
97 |
12 |
1k/p-FC6H4 |
CN |
COOEt |
2 |
O |
3l |
92 |
13 |
1l/m-FC6H4 |
CN |
COOEt |
2 |
O |
3m |
93 |
14 |
1m/o-FC6H4 |
CN |
COOEt |
2 |
O |
3n |
94 |
15 |
1n/p-ClC6H4 |
CN |
COOEt |
2 |
O |
3o |
91 |
16 |
1o/m-ClC6H4 |
CN |
COOEt |
2 |
O |
3p |
94 |
17 |
1p/p-BrC6H4 |
CN |
COOEt |
2 |
O |
3q |
86 |
18 |
1q/C6H5 |
CN |
COOEt |
2 |
O |
3r |
98 |
19 |
1r/p-MeC6H4 |
CN |
COOEt |
2 |
O |
3s |
88 |
20 |
1s/3,4-Cl2C6H3 |
CN |
COOEt |
2 |
O |
3t |
92 |
21 |
1t/2,4-Cl2C6H3 |
CN |
COOEt |
2 |
O |
3u |
93 |
22 |
1k/p-FC6H4 |
NO2 |
COOEt |
2 |
O |
3v |
86 |
23 |
1u/C6H5 |
CN |
COOEt |
3 |
O |
3w |
91 |
24 |
1v/C6H5 |
CN |
COOEt |
4 |
O |
3x |
88 |
25 |
1w/H |
CN |
COOEt |
4 |
O |
3y |
89 |
Surprisingly, we obtain excellent yield of the pyrimidin-4-one 4a when we use the N-monosubstituted 1,1-ene diamine (MEDAM) 1x as substrate in the reaction with ethyl 2-cyano-3,3-bis(methylthio)-acrylate 2a under similar conditions as in Table 2 (Table 3, entries 1–6). To expand the scope and application of this method, N-mono-substituted 1,1-ene diamines (MEDAMs) (n = 1, 2) bearing the different aromatic groups, including C6H4, p-MeC6H4 and p-FC6H4, were also used as substrate and reacted with mercaptals 2a & 2c. We obtained the pyrimidin-4-ones 4a–4f with excellent yields (92–98%). These results demonstrate that MEDAMs are all good substrates for the regioselective reaction for the synthesis of pyrimidin-4-ones. The reactions only need take 4 hours in acetonitrile at refluxing and promoted by Cs2CO3.
Table 3 Preparation of pyrimidin-4-ones 4a–4fa

|
Entry |
1/R |
EWG |
n |
Product |
Yieldb [%] |
Reagents and conditions: N-monosubstituted 1,1-ene diamines (MEDAMs) 1 (1.0 mmol), mercaptals 2 (1.0 mmol), Cs2CO3 (2.0 mmol) and CH3CN (15.0 mL). Isolated yield MEDAMs 1. |
1 |
1x/C6H5 |
CN |
1 |
4a |
93 |
2 |
1y/p-MeC6H4 |
CN |
1 |
4b |
92 |
3 |
1z/p-FC6H4 |
CN |
2 |
4c |
93 |
4 |
1a′/C6H5 |
CN |
2 |
4d |
96 |
5 |
1a′/C6H5 |
NO2 |
2 |
4e |
94 |
6 |
1b′/H |
CN |
4 |
4f |
98 |
All new compounds 3–4 were fully characterized by 1H-NMR, 13C-NMR spectroscopy, high resolution mass spectroscopy and IR spectroscopy (see ESI†). To further verify the structure of the pyridin-2-ones and pyrimidin-4-ones, the representative compound 3f & 4f were verified by the X-ray crystallographic analysis (Fig. 2, CCDC 1549520 (ref. 44) and Fig. 3, CCDC 1553238 (ref. 45)†).
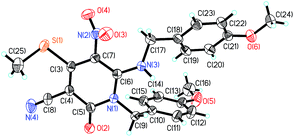 |
| Fig. 2 X-ray crystal structures of 3f. | |
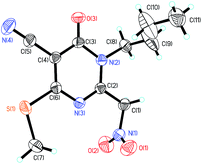 |
| Fig. 3 X-ray crystal structures of 4f. | |
To illustrate the proposed putative mechanism for the regioselective synthesis of pyridin-2-ones 3, the target compounds 3a was used as the example (Scheme 1). First, the compound 1a is reacted with 2a via the Michael addition reaction to form the intermediate 5. Then, the intermediate 5 loses a molecule of MeSH in a reaction promoted by Cs2CO3, to produce the intermediate 6. Next, the intermediate 6 forms the compound 7 via imine–enamine tautomerization. After that, the compound 7 produces intermediate 8 via an intramolecular cyclization reaction. Finally, the intermediate 8 loses one molecule of ethanol to form the target compound 3a.
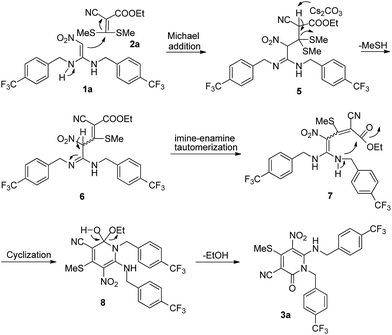 |
| Scheme 1 Proposed mechanism for synthesis of compound 3a. | |
The proposed putative mechanism for the synthesis of pyrimidin-4-ones 4 is shown in Scheme 2. First, compound 1 is reacted with 2 via the Michael addition reaction to produce the intermediate 9. Next, intermediate 9 loses one molecule of MeSH in a reaction promoted by the base Cs2CO3 and produces compound 10. Then, compound 10 forms compound 11 via intramolecular cyclization and loses one molecule of ethanol. Ultimately, compound 11 yields the products 4 via imine–enamine tautomerization.
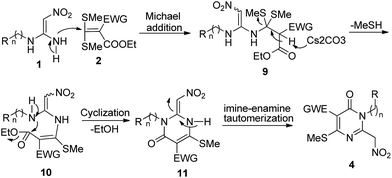 |
| Scheme 2 Proposed mechanism for synthesis of compounds 4. | |
We selected the novel pyridin-2-ones 3 and pyrimidin-4-ones 4 to evaluate their in vitro anticancer activity against human cancer cells according to procedures described in the literature.46 The tumor cell line panel consisted of gastric cancer (SGC-7901), ovarian carcinoma (Skov-3), lung adenocarcinoma (A549), and Henrietta Lacks strain of cervical cancer (Hela). Cisplatin (DDP) was used as the reference drug. The results of the cytotoxicity data are summarized in Table 4 (IC50 value, defined as the concentration corresponding to 50% growth inhibition). As shown in Table 4, some of the compounds exhibited excellent antitumor activity against the cancer cells. Actually, 3e, 3h, 3k–3o, 3q–3s and 3u are more active than cisplatin against SGC-7901 cells (Table 4, entries 4, 7, 9–13, 15–17 and 19). In particular, 3k is almost seven times more active against SGC-7901 cells than cisplatin (Table 4, entry 9). The data indicates that N,N′-diphenethylethene-1,1-diamines (n = 2) are usually the most active against the SGC-7901 cells, the N,N′-dibenzylethene-1,1-diamines (n = 1) are usually more active against SGC-7901 cells than N,N′-bis(3-phenylpropyl)ethene-1,1-diamines (n = 3) (Table 4, entries 1–8 vs. 9–19 vs. 20–22). Only three compounds 3m, 3r and 3t are more active than cisplatin against Skov-3 cells (Table 4, entries 11, 16, 18). Seven compounds (3m, 3n, 3q, 3r 3t, 3u, and 3x) are more active than cisplatin against A549 cells (Table 4, entries 11, 12, 15, 16, 18, 19, 21). The results demonstrated that N,N′-diphenethylethene-1,1-diamines (n = 2) are usually the most active against the A549 cells, while the N,N′-dibenzylethene-1,1-diamines (n = 1) are usually less active against SGC-7901 cells than N,N′-bis(3-phenylpropyl)ethene-1,1-diamines (n = 3) (Table 4, entries 9–19 vs. 20–22 vs. 1–8). Compound 3t is almost five times more active against A549 cells than cisplatin (Table 4, entry 18). Additionally, 3o and 3r are more potent against the tumor cell lines Hela (Table 4, entries 13 & 16). Overall, N,N′-diphenethylethene-1,1-diamines (n = 2) usually are the most active compounds against the SGC-7901, Skov-3, A549 and Hela cells. Among them, compound 3r was more potent against the tumor cell lines SGC-7901, Skov-3, A549 and Hela than cisplatin (DDP) in all four cell lines (Table 4, entry 16). These results suggest that N,N′-diphenethylethene-1,1-diamines (n = 2) play a key role in the modulation of the cytotoxic activities in these cancer cells (Scheme 3 & Table 4). Additionally, the substituted group also has an influence on the cytotoxic activities. Generally, the contribution order of the groups of EDAMs to cytotoxic activities is Ph > p-FPh > m-FPh > p-ClPh > 2,4-Cl2C6H3 ≈ 3,4-Cl2C6H3 > 2,4-F2C6H3 ≈ 3,4-Cl2C6H3 > p-MePh > p-MeOPh.
Table 4 Cytotoxic activities of 3–4 in vitroa (IC50, μmol mL−1)b
No. |
Compound |
SGC-7901 |
Skov-3 |
A549 |
Hela |
Cytotoxicity as IC50 for each cell line, is the concentration of compound which reduced the optical density of treated cells by 50% with respect to untreated cells using the MTT assay. Data are represented as the mean values of three independent determinations. |
1 |
3a |
11.13 |
>100 |
62.56 |
29.08 |
2 |
3c |
41.73 |
52.71 |
34.84 |
27.96 |
3 |
3d |
36.56 |
>100 |
80.72 |
68.25 |
4 |
3e |
4.73 |
61.13 |
42.45 |
23.00 |
5 |
3f |
31.17 |
50.05 |
29.88 |
>100 |
6 |
3g |
12.95 |
>100 |
>100 |
37.15 |
7 |
3h |
5.71 |
>100 |
94.52 |
>40 |
8 |
3i |
15.85 |
28.95 |
22.83 |
58.90 |
9 |
3k |
1.51 |
22.91 |
48.65 |
74.86 |
10 |
3l |
9.81 |
>100 |
47.74 |
15.45 |
11 |
3m |
2.26 |
12.38 |
4.15 |
12.30 |
12 |
3n |
4.89 |
21.06 |
5.58 |
15.94 |
13 |
3o |
6.28 |
61.70 |
16.19 |
4.14 |
14 |
3p |
>100 |
20.00 |
15.79 |
12.63 |
15 |
3q |
9.42 |
23.48 |
8.32 |
37.15 |
16 |
3r |
6.79 |
12.28 |
4.22 |
3.26 |
17 |
3s |
3.02 |
28.95 |
25.69 |
>40 |
18 |
3t |
>100 |
6.98 |
3.00 |
96.14, 14 |
19 |
3u |
2.55 |
21.94 |
8.07 |
23.00 |
20 |
3w |
23.65 |
17.95 |
25.57 |
23.00 |
21 |
3x |
>100 |
23.64 |
13.12 |
>100 |
22 |
3y |
>100 |
>100 |
27.18 |
>100 |
23 |
4a |
>100 |
>100 |
>100 |
>100 |
24 |
4b |
>100 |
>100 |
>100 |
>100 |
25 |
4c |
>100 |
>100 |
>100 |
>100 |
26 |
4d |
>100 |
>100 |
>100 |
>100 |
27 |
4e |
>100 |
>100 |
>100 |
>100 |
28 |
4f |
>100 |
>100 |
>100 |
>100 |
29 |
Cisplatin (DDP) |
11.00 |
12.78 |
15.32 |
9.94 |
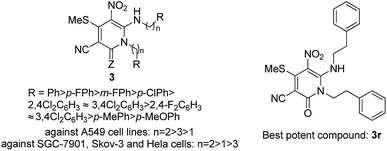 |
| Scheme 3 Structure activity relationship of pyridin-2-ones 3. | |
However, pyrimidin-4-ones 4 does not have any antitumor activity. This finding clearly indicates that the two kinds of heterocycles have different antitumor activity.
Conclusions
In conclusion, a concise and efficient method for the regioselective synthesis of two novel types of compounds including pyridin-2-ones 3 and pyrimidin-4-ones 4 had been developed. Pyridin-2-ones 3 was synthesised via the regioselective addition reaction of N,N′-disubstituted 1,1-ene diamines 1a–1w with mercaptals 2a–2c in acetonitrile promoted by Cs2CO3 at refluxing. Remarkably, pyrimidin-4-ones 4 are obtained when N-monosubstituted 1,1-ene diamines 1x–1b′ are used as substrate in the reaction with mercaptals 2 under the same conditions. The reactions have some advantages, such as excellent yield, inexpensive raw materials and convenient final treatment. The screening of the antitumor bioactivity showed that some compounds exhibited potent antitumor activity. Especially, 3k which is almost seven times more active against SGC-7901 cells than cisplatin. Compound 3t is almost five times more active against A549 cells than cisplatin. On the whole, compounds 3r proved to be the most potent derivative with IC50 values lower than 12.3 μmol L−1 of against all four human tumor cell lines, which makes it more active than cisplatin (DDP).
Experimental section
All compounds were fully characterized by spectroscopic data. The NMR spectra were recorded on a Bruker DRX500 (1H: 500 MHz, 13C: 125 MHz) or DRX600 (1H: 600 MHz, 13C: 150 MHz), chemical shifts (δ) are expressed in ppm, and J values are given in Hz, deuterated DMSO-d6 or CDCl3 was used as solvent. IR spectra were recorded on a FT-IR Thermo Nicolet Avatar 360 using KBr pellet. The reactions were monitored by thin layer chromatography (TLC) using silica gel GF254. The melting points were determined on XT-4A melting point apparatus and are uncorrected. HRMs were performed on an Agilent LC/Msd TOF instrument. All chemicals and solvents were used as received without further purification unless otherwise stated. Compounds 1 were obtained according to the literature.47 The synthetic method of compound 2 according the literature.48 Fetal bovine serum (FBS) was purchased from Hyclone Laboratories (Logan, UT, USA). The tumor cell line panel consisted of gastric cancer (SGC-7901), ovarian carcinoma (Skov-3), lung adenocarcinoma (A549), and Henrietta Lacks strain of cervical cancer (Hela) were obtained from American Type Culture Collection.
General procedure to prepare pyridin-2-ones 3
N,N′-Disubstituted 1,1-ene diamines (DEDAMs) 1 (1.0 mmol), mercaptals 2 (1.0 mmol), Cs2CO3 (2.0 mmol), acetonitrile (15.0 mL) were added into a 25 mL round-bottom flask, the mixture at reflux for about 8 h and monitored by thin layer chromatography (TLC) until the DEDAMs 1 substrate was completely consumed. After the completion of the reaction, the reaction system was cooled to room temperature. The reaction mixture was poured into 25 mL of water and ethyl acetate for extraction and separation. Then the crude product was collected by filtering and enrichment, which was purified by column chromatography (petroleum ether/EtOAc = 10
:
1) or recrystallization and obtained a series of pyridin-2-one compounds 3 with 83–98% yield.
4-(Methylthio)-5-nitro-2-oxo-1-(4-(trifluoromethyl)benzyl)-6-((4-(trifluoromethyl)-benzyl)amino)-1,2-dihydropyridine-3-carb-onitrile (3a)
Yellow solid, mp 159.1–160.2 °C; IR (KBr): 3413, 2316, 1638, 1618, 1328, 1165, 1124, 1069 cm−1; 1H NMR (600 MHz, DMSO-d6): δ = 2.74 (s, 3H, CH3), 4.17 (m, 2H, CH2), 5.47 (m, 2H, CH2), 7.14–7.16 (m, 2H, ArH), 7.35–7.37 (m, 2H, ArH), 7.44–7.45 (m, 2H, ArH), 7.66–7.68 (m, 2H, ArH), 8.37 (br, 1H, NH); 13C NMR (150 MHz, DMSO-d6): δ = 19.4, 45.3, 49.2, 89.2, 116.8, 122.0, 122.7, 123.6, 123.8, 125.2, 125.3, 125.7, 125.9, 127.2, 127.4, 128.7, 128.7, 129.2, 139.5, 140.9, 149.9, 156.2, 159.2; HRMS (ESI-TOF): m/z calcd for C23H15F6N4O3S [M − H]−, 541.0775; found, 541.0773.
1-(4-Fluorobenzyl)-6-((4-fluorobenzyl)amino)-4-(methylthio)-5-nitro-2-oxo-1,2-dihydropyridine-3-carbonitrile (3b)
Yellow solid, mp 177.8–178.0 °C; IR (KBr): 3334, 1639, 1554, 1512, 1494, 1466, 1328, 1235 cm−1; 1H NMR (600 MHz, DMSO-d6): δ = 2.72 (s, 3H, CH3), 4.10 (m, 2H, CH2), 5.36 (m, 2H, CH2), 6.97–7.02 (m, 4H, ArH), 7.14–7.21 (m, 4H, ArH), 8.31 (br, 1H, NH); 13C NMR (150 MHz, DMSO-d6): δ = 19.4, 44.9, 49.0, 89.0, 115.3, 115.5, 115.8, 115.9, 116.9, 122.6, 128.9, 129.0, 130.6, 130.7, 132.3, 132.3, 149.8, 156.0, 159.2, 162.1, 162.1; HRMS (ESI-TOF): m/z calcd for C21H15F2N4O3S [M − H]−, 441.0838; found, 441.0836.
General procedure for prepared pyrimidin-4-ones 4
N-Monosubstituted 1,1-ene diamines (MEDAMs) 1 (1.0 mmol), mercaptals 2 (1.0 mmol), Cs2CO3 (2.0 mmol) and acetonitrile (15.0 mL) were added into a 25 mL round-bottom flask, the mixture at reflux for about 4 h and monitored by TLC until the MEDAMs 1 substrate was completely consumed. After the completion of the reaction, the reaction system was cooled to room temperature. The reaction mixture was poured into 25 mL of water and 25 mL ethyl acetate for extraction and separation. Then the crude product was collected by filtering and enrichment, which was purified by column chromatography (petroleum ether/EtOAc = 3
:
1) and obtained a series of pyrimidin-4-ones 4 with 92–98% yield.
1-Benzyl-4-(methylthio)-2-(nitromethyl)-6-oxo-1,6-dihydropy-rimidine-5-carbonitrile (4a)
Orange solid, mp 115.0–116.2 °C; IR (KBr): 3291, 2926, 2206, 1506, 1439, 1291, 1215, 832 cm−1; 1H NMR (500 MHz, DMSO-d6): δ = 2.54 (s, 3H, CH3), 5.25 (m, 2H, CH2), 6.11 (s, 2H, CH2), 7.27–7.32 (m, 2H, ArH), 7.32–7.39 (m, 3H, ArH); 13C NMR (125 MHz, DMSO-d6): δ = 13.4, 47.2, 78.1, 94.1, 114.1, 127.3, 127.3, 128.4, 129.3, 129.3, 134.4, 154.8, 158.2, 174.3; HRMS (ESI-TOF): m/z calcd for C14H11N4O3S [M − H]−, 315.0557; found, 315.0546.
1-(4-Methylbenzyl)-4-(methylthio)-2-(nitromethyl)-6-oxo-1,6-dihydropyrimidine-5-carbonitrile (4b)
White solid, mp 158.0–158.5 °C; IR (KBr): 3441, 2930, 2222, 1684, 1572, 1506, 1379, 974 cm−1; 1H NMR (500 MHz, DMSO-d6): δ = 2.29 (s, 3H, CH3), 2.53 (s, 3H, CH3), 5.21 (m, 2H, CH2), 6.10 (s, 2H, CH2), 7.18 (m, 4H, ArH); 13C NMR (125 MHz, DMSO-d6): δ = 13.4, 21.1, 46.9, 78.1, 94.0, 114.1, 126.9, 127.1, 129.9, 129.9, 131.4, 137.8, 154.8, 158.2, 174.2; HRMS (ESI-TOF): m/z calcd for C15H13N4O3S [M − H]−, 329.0714; found 329.0703.
Conflicts of interest
There are no conflicts to declare.
Acknowledgements
This work was supported by the National Natural Science Foundation of China (No. 21362042, 21662042, U1202221, 21262042), the Natural Science Foundation of Yunnan Province (No. 2017FA003), the Reserve Talent Foundation of Yunnan Province for Middle-aged and Young Academic and Technical Leaders (No. 2012HB001), Donglu Schloars of Yunnan University, Excellent Young Talents, Yunnan University, and High-Level Talents Introduction Plan of Yunnan Province.
Notes and references
-
(a) L. Ren, J. Grina, D. Moreno, J. F. Blake, J. J. Gaudino, R. Garrey, A. T. Metcalf, M. Burkard, M. Martinson, K. Rasor, H. Chen, B. Dean, S. E. Gould, P. Pacheco, S. Shahidi-Latham, J. Yin, K. West, W. Wang, J. G. Mo and J. B. Schwarz, J. Med. Chem., 2015, 58, 1976 CrossRef CAS PubMed;
(b) L. Wang, J. K. Pratt, T. Soltwedel, G. S. Sheppard, S. D. Fidanze, D. Liu, L. A. Hasvold, R. A. Mantei, J. H. Holms, W. J. McClellan, M. D. Wendt, C. Wada, R. Frey, T. M. Hansen, R. Hubbard, C. H. Park, L. Li, T. J. Magoc, D. H. Albert, X. Lin, S. E. Warder, P. Kovar, X. Huang, D. Wilcox, R. Wang, G. Rajaraman, A. M. Petros, C. W. Hutchins, S. C. Panchal, C. Sun, S. W. Elmore, Y. Shen, W. M. Kati and K. F. McDaniel, J. Med. Chem., 2017, 60, 3828 CrossRef CAS PubMed.
- J. A. D. Good, J. Silver, C. Núñez-Otero, W. Bahnan, K. S. Krishnan, O. Salin, P. Engström, R. Svensson, P. Artursson, Å. Gylfe, S. Bergström and F. Almqvist, J. Med. Chem., 2016, 59, 2094 CrossRef CAS PubMed.
- J. M. Cid, G. Tresadern, G. Duvey, R. Lütjens, T. Finn, J.-P. Rocher, S. Poli, J. A. Vega, A. de Lucas, E. Matesanz, M. L. Linares, J. I. Andrés, J. Alcazar, J. M. Alonso, G. J. Macdonald, D. Oehlrich, H. Lavre, A. Ahnaous, W. Drinkenburg, C. Mackie, S. Pype, D. Gallacher and A. A. Trabanco, J. Med. Chem., 2014, 57, 6495 CrossRef CAS PubMed.
- K. L. Van, C. Cauvin, S. de Walque, B. Georges, S. Boland, V. Martinelli, D. Demonte, F. Durant, L. Hevesi and C. V. Lint, J. Med. Chem., 2009, 52, 3636 CrossRef PubMed.
- J. M. Hoffman, J. S. Wai, C. M. Thomas, R. B. Levin, J. A. O'Brien and M. E. Goldman, J. Med. Chem., 1992, 35, 3784 CrossRef CAS PubMed.
- J. M. Hoffman, A. M. Smith, C. S. Rooney, T. E. Fisher, J. S. Wai, C. M. Thomas, D. L. Bamberger, J. L. Barnes and T. M. Williams, J. Med. Chem., 1993, 36, 953 CrossRef CAS PubMed.
- W. S. Saari, J. S. Wai, T. E. Fisher, C. M. Thomas, J. M. Hoffman, C. S. Rooney, A. M. Smith, J. H. Jones and D. L. Bamberger, J. Med. Chem., 1992, 35, 3792 CrossRef CAS PubMed.
- J. S. Wai, T. M. Williams, D. L. Bamberger, T. E. Fisher, J. M. Hoffman, R. J. Hudcosky, S. C. MacTough, C. S. Rooney and W. S. Saari, J. Med. Chem., 1993, 36, 249 CrossRef CAS PubMed.
- W. S. Saari, J. M. Hoffman, J. S. Wai, T. E. Fisher, C. S. Rooney, A. M. Smith, C. M. Thomas, M. E. Goldman and J. A. O'Brien, J. Med. Chem., 1991, 34, 2922 CrossRef CAS PubMed.
- N. A. Hamdy and A. M. Gamal-Eldeen, Eur. J. Med. Chem., 2009, 44, 4547 CrossRef CAS PubMed.
- Z. Lv, C. Q. Sheng, T. T. Wang, Y. K. Zhang, J. Liu, J. L. Feng, H. L. Sun, H. Y. Zhong, C. J. Niu and K. Li, J. Med. Chem., 2010, 53, 660 CrossRef CAS PubMed.
- G. C. Moraski, L. D. Markley, P. A. Hipskind, H. Boshoff, S. Cho, S. G. Franzblau and M. J. Miller, ACS Med. Chem. Lett., 2011, 2, 466 CrossRef CAS PubMed.
- J. J. Parlow, R. G. Kurumbail, R. A. Stegeman, A. M. Stevens, W. C. Stallings and M. S. South, J. Med. Chem., 2003, 46, 4696 CrossRef CAS PubMed.
- R. W. Hartmann and M. Reichert, Arch. Pharm., 2000, 333, 145 CrossRef CAS PubMed.
- V. S. Prasadarao Lingam, D. H. Dahale, V. E. Rathi, Y. B. Shingote, R. R. Thakur, A. S. Mindhe, S. Kummari, N. Khairatkar-Joshi, M. Bajpai, D. M. Shah, R. S. Sapalya, S. Gullapalli, P. K. Gupta, G. S. Gudi, S. B. Jadhav, R. Pattem and A. Thomas, J. Med. Chem., 2015, 58, 8292 CrossRef PubMed.
- H. J. Jessen and K. Gademann, Nat. Prod. Rep., 2010, 27, 1168 RSC.
- M. Ando, T. Wada and N. Sato, Org. Lett., 2006, 8, 3805 CrossRef CAS PubMed.
- H. Schirok, C. Alonso-Alija, J. Benet-Buchholz, A. H. Göller, R. Grosser, M. Michels and H. Paulsen, J. Org. Chem., 2005, 70, 9463 CrossRef CAS PubMed.
- M. P. Balu, G. Singh, H. lla and H. Junjappa, Tetrahedron Lett., 1986, 27, 117 CrossRef CAS.
- J. Marinello, C. Marchand, B. T. Mott, A. Bain, C. J. Thomas and Y. Pommier, Biochemistry, 2008, 47, 9345 CrossRef CAS PubMed.
- F. Manetti, J. A. Esté, I. Clotet-Codina, M. Armand-Ugón, G. Maga, E. Crespan, R. Cancio, C. Mugnaini, C. Bernardini, A. Togninelli, C. Carmi, M. Alongi, E. Petricci, S. Massa, F. Corelli and M. Botta, J. Med. Chem., 2005, 48, 8000 CrossRef CAS PubMed.
- D. Rotili, D. Tarantino, M. B. Nawrozkij, A. S. Babushkin, G. Botta, B. Marrocco, R. Cirilli, S. Menta, R. Badia, E. Crespan, F. Ballante, R. Ragno, J. A. Esté, G. Maga and A. Mai, J. Med. Chem., 2014, 57, 5212 CrossRef CAS PubMed.
- K. K. Ariën, M. V. Johan Michiels, J. Joosens, K. Vereecken, P. V. D. Veken, S. Abdellati, V. Cuylaerts, T. Crucitti, L. Heyndrickx, J. Heeres, K. Augustyns, P. J. Lewi and G. Vanham, J. Antimicrob. Chemother., 2013, 68, 2038 CrossRef PubMed.
- M. M. Hossain and M. A. Parniak, J. Virol., 2006, 80, 4440 CrossRef CAS PubMed.
- O. J. D'Cruz and F. M. Uckun, J. Antimicrob. Chemother., 2006, 57, 411 CrossRef PubMed.
- Q. Abdool Karim, S. S. Abdool Karim, J. A. Frohlich, A. C. Grobler, C. Baxter, L. E. Mansoor, A. B. M. Kharsany, S. Sibeko, K. P. Mlisana, Z. Omar, T. N. Gengiah, S. Maarschalk, N. Arulappan, M. Mlotshwa, L. Morris and D. Taylor, Science, 2010, 329, 1168 CrossRef CAS PubMed.
- J. Younkin, S. A. Gaitonde, A. Ellaithy, R. Vekariya, L. Baki, J. L. Moreno, S. Shah, P. Drossopoulos, K. S. Hideshima, J. M. Eltit, J. González-Maeso, D. E. Logothetis, M. Dukat and R. Glennon, ACS Chem. Neurosci., 2016, 7, 1292 CrossRef CAS PubMed.
- S. D. Turco, S. Sartini, C. Sentieri, C. Saponaro, T. Navarra, B. Dario, F. D. Settimo, C. L. Motta and G. Basta, Eur. J. Med. Chem., 2014, 72, 102 CrossRef PubMed.
- Y.-L. Li, B. W. Metcalf and A. P. Combs, EP2448938, 2015.
- R. A. Nugent and S. T. Schlachter, WO9511235A1, 1995.
- J. Bagli, T. Bogri, B. Palameta, S. Rakhit, S. Peseckis, J. McQuillan and D. K. H. Lee, J. Med. Chem., 1988, 31, 814 CrossRef CAS PubMed.
- K. M. Belyk, H. G. Morrison, P. Jones and V. Summa, WO2006060712A2, 2006.
- C. Hoornaert, and A. Wick, EP607077A1, 1994.
- Y. S. Chun, J. H. Kim, S. Y. Choi, Y. O. Ko and S. Lee, Org. Lett., 2012, 14, 6358 CrossRef CAS PubMed.
-
(a) B. Zhou, Z.-C. Liu, W.-W. Qu, R. Yang, X.-R. Lin, S.-J. Yan and J. Lin, Green Chem., 2014, 16, 4359 RSC;
(b) F.-C. Yu, Z.-Q. Chen, X.-P. Hao, S.-J. Yan, R. Huang and J. Lin, RSC Adv., 2014, 4, 6110 RSC;
(c) L. Chen, R. Huang, X.-X. Du, S.-J. Yan and J. Lin, ACS Sustainable Chem. Eng., 2017, 5, 1899 CrossRef CAS.
-
(a) X. B. Chen, Z.-C. Liu, L.-F. Yang, S.-J. Yan and J. Lin, ACS Sustainable Chem. Eng., 2014, 2, 1155 CrossRef CAS;
(b) X.-B. Chen, Z.-C. Liu, X.-R. Lin, R. Huang, S.-J. Yan and J. Lin, ACS Sustainable Chem. Eng., 2014, 2, 2391 CrossRef CAS.
-
(a) F.-C. Yu, X.-R. Lin, Z.-C. Liu, J.-H. Zhang, F.-F. Liu, W. Wu, Y.-L. Ma, W.-W. Qu, S.-J. Yan and J. Lin, ACS Omega, 2017, 2, 873 CrossRef CAS;
(b) K.-M. Wang, Y.-L. Ma, X.-R. Lin, S.-J. Yan and J. Lin, RSC Adv., 2015, 5, 36472 RSC.
-
(a) M. Papmeyer, C. A. Vuilleumier, G. M. Pavan, K. O. Zhurov and K. Severin, Angew. Chem., Int. Ed., 2016, 55, 1685 CrossRef CAS PubMed;
(b) N. Poomathi, P. T. Peumal and S. Ramakrishna, Green Chem., 2017, 19, 2524 RSC.
- Sunesis pharmaceuticals, INC. WO2006/65703 A1, 2006.
- H. Schirok, C. Alonso-Alija, J. Benet-Buchholz, A. H. Goeller, R. Grosser, M. Michels and H. Paulsen, J. Org. Chem., 2005, 70, 9463 CrossRef CAS PubMed.
-
(a) A. M. Kelly-Rowley, V. M. Lynch and E. V. Anslyn, J. Am. Chem. Soc., 1995, 117, 3438 CrossRef CAS;
(b) A. M. Kelly-Rowley, L. A. Cabell and E. V. Anslyn, J. Am. Chem. Soc., 1991, 113, 9687 CrossRef CAS;
(c) A. Alizadeh, A. Zarei and A. Rezvanian, Synthesis, 2011, 3, 497 CrossRef.
-
(a) S. Lu, X. Shao, Z. Li, Z. Xu, S. Zhao, Y. Wu and X. Xu, J. Agric. Food Chem., 2012, 60, 322 CrossRef CAS PubMed;
(b) N. Chen, X. Meng, F. Zhu, J. Cheng, X. Shao and Z. Li, J. Agric. Food Chem., 2015, 63, 1360 CrossRef CAS PubMed;
(c) H. Bao, X. Shao, Y. Zhang, Y. Deng, X. Xu, Z. Liu and Z. Li, J. Agric. Food Chem., 2016, 64, 5148 CrossRef CAS PubMed;
(d) L.-R. Wen, Z.-R. Li, M. Li and H. Cao, Green Chem., 2012, 14, 707 RSC.
-
(a) A. Maryamabadi, A. Hasaninejad, N. Nowrouzi, G. Mohbbi and B. Asghari, Bioorg. Med. Chem., 2016, 24, 1408 CrossRef CAS PubMed;
(b) A. Maryamabadi, A. Hasaninejad, N. Nowrouzi and G. Mohebbi, Bioorg. Med. Chem., 2017, 25, 2507 CrossRef PubMed.
- CCDC 1549520 contain the supplementary crystallographic data for compound 3f.†.
- CCDC 1553238 contain the supplementary crystallographic data for compound 4f.†.
- S.-J. Yan, C. Huang, X.-H. Zeng, R. Huang and J. Lin, Bioorg. Med. Chem. Lett., 2010, 20, 48 CrossRef CAS PubMed.
- R. C. da Silva, G. P. da Silva, D. P. Sangi, J. G. de M. Pontes, A. G. Ferreira, A. G. Corrêa and M. W. Paixão, Tetrahedron, 2013, 69, 9007 CrossRef CAS.
-
(a) W. M. Al-Adiwish, M. I. M. Tahir and W. A. Yaacob, Synth. Commun., 2013, 43, 3203 CrossRef CAS;
(b) Y.-C. Wu, H.-J. Li and H.-Z. Yang, Org. Biomol. Chem., 2010, 8, 3394 RSC.
Footnotes |
† Electronic supplementary information (ESI) available: CCDC 1549520 (3f), 1553238 (4f). For ESI and crystallographic data in CIF or other electronic format see DOI: 10.1039/c7ra06466g |
‡ The two authors contributed equally to this paper. |
|
This journal is © The Royal Society of Chemistry 2017 |
Click here to see how this site uses Cookies. View our privacy policy here.