DOI:
10.1039/C7RA08005K
(Paper)
RSC Adv., 2017,
7, 42685-42689
The study of perylene diimide–amino acid derivatives for the fluorescence detection of anions†
Received
20th July 2017
, Accepted 21st August 2017
First published on 4th September 2017
Abstract
Four types of perylene diimide–amino acid derivatives (H2PDIAla, H2PDIGlu, H2PDIPhe, H2PDITyr) were chosen to interact with anions. The UV-vis, fluorescence (in DMF solution) and NMR spectra in DMSO-d6 solution were studied. The results indicated that the four types of derivatives exhibit specific selectivity and high sensitivity for F− and OH− ions. The NMR titrations of the derivatives with F− and OH− ions confirmed the synergetic effect of hydrogen bonding and anion–π interaction between the derivatives and the anions (F−, OH−). In addition, we made a simple test-paper to develop its potential applications.
Introduction
Hydrogen bonding is the most important and widespread intermolecular interaction.1 The scientific branches involving the importance of hydrogen bonding are very diverse, including inorganic and organic chemistry, supramolecular chemistry, biochemistry, and medicine.2 It is operative in determining molecular conformation, molecular aggregation, and the function of a vast number of chemical systems ranging from inorganic to biological and is emerging as a powerful tool in the design of functional materials.3 In recent years, receptors using hydrogen bonding have been extensively developed, and a variety of functions have been investigated, including recognition, sensing, catalysis, and carrier-mediated transport across membranes.4
Perylene Diimide (PDI) is a valuable functional dye, which has numerous potential properties such as unique light-harvesting, redox properties, high chemical persistency, thermal durability, and outstanding optical and electronic properties. It has been applied as pigments, in fluorescent detectors, and semiconductors in organic electronics and photovoltaics.5
Anions are ubiquitous in environment and food samples and play an important role in numerous types of biological and medicinal processes.6 The design and development of synthetic receptors to recognize specific anions selectively had received more and more attention in recent years.7 Using PDI derivatives to detect anions became a developing trend of research. Sourav Saha and co-workers used aliphatic chain modified PDI to detect F− and OH−, which demonstrated anion–π electronic interactions from OH− and F− ions to PDI in aprotic solvents.8 On the other hand, a type of PDI derivative incorporated with functional groups of chloroacetamide (–NH–(C
O)–CH2–Cl) has been studied for fluoride ion detection.9 It possesses a specific selectivity and high sensitivity for fluoride ion (F−) among the halogen anions. The recognition was attributed to the intermolecular proton transfer between a hydrogen atom on the amide of the sensor and the fluoride anion. Based on this result, a new colorimetric and ratiometric fluorescent sensor for naked-eye detection of fluoride ion based on PDI–amide derivatives has been designed and synthesized.10 In consideration of the important effect of hydrogen bonding, the PDI derivatives equipped with a –COOH group, such as amino acid, should form a strong hydrogen bonding. However, there is no report of PDI–amino acid derivatives applied in detection of anions in literature. Amino acids are essential structural units and physiologically active substances of life and are environment friendly. The new material designed with amino acids has advantages of being green and safe, low price, easy to synthesize and so on.
Herein, we report the studies of PDI–amino acid derivatives (H2PDIAAs) as fluorescent sensors to detect anions, particularly halogen ions, in organic solvents. Four types of H2PDIAAs have been selected, which are perylene diimides functionalized with L-alanine (H2PDIAla), L-glutamic acid (H2PDIGlu), L-phenylalanine (H2PDIPhe) and L-tyrosine (H2PDITyr) (Scheme 1). A comparative study and discussion on their fluorescent properties have been provided explaining their interaction with halogen ions in DMF as H2PDIAAs have good solubility and strong fluorescence in DMF only. This work applies the PDI–amino acid derivatives on the fluorescent sensor to detect anions based on hydrogen bonding for the first time.
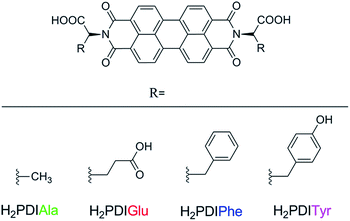 |
| Scheme 1 Structural representations of PDI functionalized with L-alanine (H2PDIAla), L-glutamic acid (H2PDIGlu), L-phenylalanine (H2PDIPhe) and L-tyrosine (H2PDITyr). | |
Experimental
Materials and measurements
All materials were reagent grade and obtained from commercial sources and used without further purification. IR spectra were recorded on a Nicolet-360 FT-IR spectrophotometer using KBr pellets. Absorbance spectra were obtained with a Persee TU-1950 spectrophotometer; the luminescent spectra were recorded at room temperature on Hitachi F-7000 FL spectrophotometer with a xenon arc lamp ashen light source spectra, with the pass width of Ex = 2.5 nm, Em = 2.5 nm. NMR spectra were recorded on a Bruker 400 MHz, and DMSO-d6 was used as the solvent, with TMS as the internal standard.
General procedure for H2PDIAAs
The four types of H2PDIAAs have been synthesized according to the method reported in literature.11 Furthermore, 3,4,9,10-perylene tetracarboxylic acid dianhydride (0.500 g, 1.28 mmol) in DMSO (50 mL) was heated at 100 °C with stirring. The amino acids, L-alanine (H2PDIAla) (0.341 g, 3.83 mmol), L-glutamic acid (H2PDIGlu) (0.563 g, 3.83 mmol), L-phenylalanine (H2PDIPhe) (0.632 g, 3.83 mmol) or L-tyrosine (H2PDITyr) (0.594 g, 3.83 mmol) was dissolved in 2 M KOH aq. (2.5 mL). The solution of potassium amino acid salt was added to the mixture. The mixture was stirred for 3 hours at 100 °C before cooling to the room temperature with the formation of a precipitate, which was recovered by filtration and recrystallized from hot water and acetone to yield the dipotassium salt K2PDIAAs. Moreover, 2 M HCl aq. (5 mL) was added, recovered by filtration, and washed extensively with water before being dried in vacuo (NMR, ESI-MS, IR for H2PDIAAs in Fig. S1–S16†). N,N′-Bis-(L-alanine)-3,4,9,10-perylene tetracarboxylic diimide (H2PDIAla). Yields: H2PDIAla (0.512 g, 75%). 1H NMR (400 MHz, DMSO-d6): δ 7.88 (d, J = 7.7 Hz, 4H), 7.75 (d, J = 8.1 Hz, 4H), 5.52 (dd, J = 13.9, 6.8 Hz, 2H), 1.67 (d, J = 7.0 Hz, 6H). 13C NMR (100 MHz, DMSO-d6): δ 172.2, 162.2, 133.2, 130.8, 127.9, 124.4, 123.5, 122.0, 49.5, 15.4. IR (film): ν = 1747, 1695, 1653, 1592, 1437, 1343, 1256, 974, 809, 747 cm−1. ESI-MS: m/z = 535 [M + H]+. N,N′-Bis-(L-glutamic acid)-3,4,9,10-perylene tetracarboxylic diimide (H2PDIGlu). Yields: H2PDIGlu (0.611 g, 73%). 1H NMR (400 MHz, DMSO-d6): δ 12.5 (br s, 4H), 8.43–7.94 (m, 8H), 5.66–5.53 (m, 2H), 2.61–2.47 (m, 4H), 2.46–2.25 (m, 4H). 13C NMR (100 MHz, DMSO-d6): δ 174.0, 170.9, 162.3, 133.1, 130.7, 128.0, 124.6, 123.1, 121.8, 52.7, 30.8, 23.9. IR (film): ν = 1751, 1697, 1642, 1591, 1363, 1255, 1168, 809, 747 cm−1. ESI-MS: m/z = 651 [M + H]+. N,N′-Bis-(L-phenylalanine)-3,4,9,10-perylene tetracarboxylic diimide (H2PDIPhe). Yields: H2PDIPhe (0.652 g, 74%). 1H NMR (400 MHz, DMSO-d6): δ 8.32–7.98 (m, 8H), 7.34–7.01 (m, 10H), 6.03–5.90 (m, 2H), 3.95–3.18 (m, 4H). 13C NMR (100 MHz, DMSO-d6): δ 170.6, 162.18, 137.9, 133.8, 131.1, 129.1, 128.2, 128.1, 126.4, 125.2, 123.6, 121.7, 54.0, 34.3. IR (film): ν = 1736, 1698, 1659, 1592, 1435, 1365, 1254, 1168, 810, 747 cm−1. ESI-MS: m/z = 687 [M + H]+. N,N′-Bis-(L-tyrosine)-3,4,9,10-perlene tetracarboxylic diimide (H2PDITyr). Yields: H2PDITyr (0.593 g, 70%). 1H NMR (400 MHz, DMSO-d6): δ 8.29–7.75 (m, 8H), 7.15–7.97 (m, 4H), 6.64–6.51 (m, 4H), 5.99–5.85 (m, 2H), 3.58–3.46 (m, 2H), 3.44–3.31 (m, 2H). 13C NMR (100 MHz, DMSO-d6): δ 170.8, 162.1, 155.9, 133.4, 130.9, 130.1, 127.9, 127.8, 124.9, 123.2, 121.6, 115.0, 54.1, 33.4. IR (film): ν = 1692, 1649, 1592, 14
434, 1402, 1367, 1253, 1009, 825, 745 cm−1. ESI-MS: m/z = 719 [M + H]+.
Preparation of solutions for absorbance and fluorescence detections
The stock solution of H2PDIAAs was prepared in DMF (1 × 10−5 M), and each inorganic salt (NaH2PO4, Na2HPO4, Na3PO4, Na4P2O7 (PPi), NaNO3, NaHCO3, Na2CO3, NaHSO4, Na2SO4, tetrabutylammonium fluoride (TBAF), tetrabutylammonium chloride (TBACl), tetrabutylammonium bromide (TBABr), tetrabutylammonium iodide (TBAI), tetrabutylammonium hydroxide (TBAOH)) was dissolved in DMF (1.5 × 10−2 M).
Test-paper measurement
The silica gel plate (2 × 1.5 cm2) was immersed in the DMF solution of H2PDIAla (1 × 10−5 M) by soaking half of it and then dried using the blower. The letters (BIT) were written both in the upper and lower panels with the TBAF solution and then put under the visible light and UV light (λ = 365 nm).
Fluorescence lifetime decay
Fluorescence decay lifetime was measured using a time-correlated single photon counting instrument. Only freshly prepared solutions were used for the spectroscopic study, and all experiments were carried out at room temperature (298 K). Fluorescence lifetime was measured using Fluorolog-Tau-3 picosecond level fluorescence spectroscopy.
Results and discussion
UV-vis spectra
The UV-vis spectra of H2PDIAAs show the typical features of perylene absorption with three peaks.12 The interactions of H2PDIAAs with different anions were studied by UV-vis absorption spectroscopy, in which there was found to be an obvious change in the presence of F− and OH− ions among Cl−, Br−, I−, H2PO4−, HPO42−, PO43−, PPi, NO3−, HCO3−, CO32−, HSO4−, and SO42− ions (Fig. S17†). Fig. 1 exhibits the results of UV-vis titration by adding TBAF into H2PDIAAs in DMF solution. Clearly, the typical absorption peaks red-shifted, and a new peak appeared around 700 nm. With the same condition, the UV-vis spectrometric titrations of H2PDIAAs with other anions did not show any significant change. Moreover, the inset pictures in Fig. 1 suggest that it has a good linearity between the absorbance at 700 nm with the concentrations of F− from 0.2 eq. to 6 eq., which indicates that H2PDIAla can detect F− quantitatively. The linear equation was found to be y = 0.0152x + 0.0008 (R = 0.9991), where “y” is the absorbance at 700 nm measured at a given F− concentration, and “x” represents the equivalent of F− added. When the concentration of F− was less than 1 equivalent of PDI, there was no large absorbance around 700 nm, and the absorbance appeared after the concentration of F− was more than 1 equivalent of PDI. The same trend in the detection was found using H2PDIPhe and H2PDITyr (Fig. 1c and d inset), but H2PDIGlu showed a different tendency in detection of F− because it contains four carboxyl groups and has a capacity to accept more F− ions (Fig. 1b inset, S18†). The OH− ion shows similar phenomenon with F− ion when it interacts with H2PDIAAs (Fig. S19†).
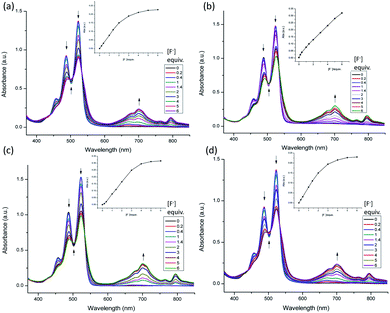 |
| Fig. 1 UV-vis titration of H2PDIAAs with TBAF in DMF (1 × 10−5 M), (a) H2PDIAla, (b) H2PDIGlu, (c) H2PDIPhe, (d) H2PDITyr. Inset: UV absorbance intensity at 700 nm vs. concentration of F− ion. | |
Fluorescent properties
The interactions between H2PDIAAs and various anions were studied by fluorescence spectra. There was an obvious change in fluorescence spectra of H2PDIAAs in the presence of F− and OH− ions as shown in Fig. S20.† Furthermore, fluorescence titration by adding standard solution of TBAF in DMF has been applied in investigation of H2PDIAAs interacting with F− and OH− ions (Fig. 2 and S21†). The four compounds have different fluorescence intensity from the strongest 7500 (a.u., H2PDIPhe) to the lowest 1500 (a.u., H2PDITyr), which may be related to the different substitutes on the branched chain of the four compounds. In order to understand the fluorescence behavior of H2PDIAAs in solution better, we measured the fluorescence lifetime of these four compounds in DMF and calculated the theoretical quantum yield, shown in Table 1, in which the quantum yield of H2PDIPhe in the four compounds is the largest one, and H2PDITyr is the lowest. As a general rule, the fluorescence intensity is proportional to the fluorescence quantum yield. Therefore, this result is consistent with the fluorescence intensity measured with the same conditions, as shown in Fig. 2. The fluorescence lifetime of H2PDITyr is longer than that of the other three H2PDIAAs.
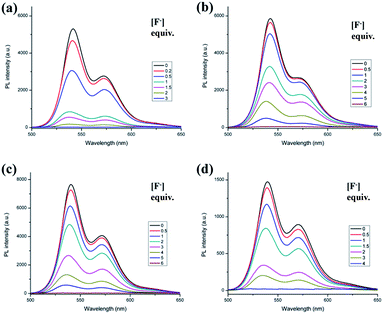 |
| Fig. 2 Fluorescence emission of H2PDIAAs with TBAF titration in DMF (1 × 10−5 M) (λex = 525 nm), (a) H2PDIAla, (b) H2PDIGlu, (c) H2PDIPhe and (d) H2PDITyr. | |
Table 1 The quantum yield (ΦF) and lifetime of H2PDIAAs in DMF solution (1 × 10−5 M)
|
H2PDIAla |
H2PDIGlu |
H2PDIPhe |
H2PDITyr |
The quantum yield (ΦF) was measured relative to the fluorescein solution in 0.1 M NaOH (ΦF = 0.79).13 Fluorescence lifetime values (λem = 541, 572 nm). |
Quantum yield (ΦF)a |
0.27 |
0.36 |
0.66 |
0.11 |
τ (ns)b |
4.51 |
4.65 |
4.54 |
5.06 |
As shown in Fig. 2, the H2PDIAla has been found to have sensitivity higher than that of the other three compounds. The OH− ion also has similar phenomenon with the interaction of H2PDIAAs (Fig. S21†), and fluorescence quenching can also be observed.
Fluorescent detectors for anions
Besides the UV-vis and fluorescent spectra, the selectivity of H2PDIAAs to F− and OH− ions can also be well identified by the naked eye with colorimetric change (Fig. 3). The interactions of H2PDIAla probe with various anions (H2PO4−, HPO42−, PO43−, PPi, NO3−, HCO3−, CO32−, HSO4−, SO42−, Cl−, Br−, I−) compared with F− were studied. Upon adding various anions to the blank solution of H2PDIAla, no appreciable color change was noticed under the visible light and UV light (Fig. 3a). Therefore, it was inferred that there were no interactions between H2PDIAla and various anions mentioned. Furthermore, with the addition of F− ion to the above solution, the color of both blank solution and that containing various anion's solution changed from yellow to brown as observed by the naked eye and fluorescence quenching under UV light (Fig. 3b). Clearly, the H2PDIAla can be a fluorescent probe for F− ion even with the existence of various other anions. Moreover, the detections by H2PDIGlu, H2PDIPhe and H2PDITyr were almost the same except for the difference of color changes with H2PDIGlu from yellow to purple, H2PDIPhe from yellow to brick red, and H2PDITyr from jacinth to brown (Fig. S22–S24†). The detection for OH− ion by the fluorescent probe H2PDIAAs has been processed, and a similar phenomenon was observed with fluorescence quenching and a color change could be observed by the naked eye (Fig. S25–S28†).
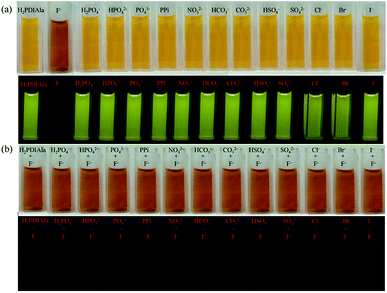 |
| Fig. 3 (a) The photographs of colorimetric identification by naked eye and fluorescence emission (λ = 365 nm) of H2PDIAla with various ions (6 eq., each). (b) The photographs of colorimetric identification by naked eye and fluorescence emission (λ = 365 nm) of H2PDIAla–F− ion with various ions (6 eq., each). | |
In order to improve the convenience and rapidity of F− ion detection out of the laboratory, we made a simple test-paper, which used the existing H2PDIAA solution with silica gel plate. By soaking half of the silica gel plate in the solution and drying, the letters “BIT” were written in both the upper and lower panels with the TBAF solution. There was a significant difference between the soaked part and the control part. The letters “BIT” could clearly be observed under the visible light and UV light, but there was nothing on the control part (Fig. 4). It suggests that this method can be used to detect F− ion conveniently and practically.
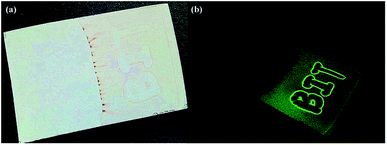 |
| Fig. 4 Photographs of test-paper of H2PDIAla solution in DMF (1 × 10−5 M) of TBAF. (a) Visible light of the test-paper, (b) UV light (λ = 365 nm) of the test-paper. | |
Mechanism of the fluorescent detectors
The NMR titration experiment is very useful in studying the mechanism of H2PDIAAs to detect anions in solutions (Fig. 5). According to our NMR titration experiment, when the addition of F− was equal or less than 1 equivalent of PDI, the signal of core structure protons always existed, whereas the signal of carboxylic acid moved to a low magnetic field and became broader. On the other hand, the signal of core structure protons gradually disappeared with the increasing of F− concentration after more than 1 equivalent of PDI. The results indicated that when the concentration of F− was equal or less than 1 equivalent of PDI, the association between H2PDIAAs and F− could be through the hydrogen bonding between the H+ of carboxylic acid (–COOH proton) and F−(COO–H⋯F−). The formation of hydrogen bonding followed by deprotonation of the –COOH fragment could reduce the electron density on the carboxylic moiety, which decreases the electron density of the core benzene ring of PDI, deshielding the corresponding protons and moving them to a low magnetic field.14 Clearly, when the concentration of F− is more than 1 equivalent of PDI, there is an interaction between core structure and F−. Moreover, UV-vis titration experiments indicated that the PDI anion radicals were generated at high concentration of F−. These results indicated that anion–π interaction may play the leading role in the association between H2PDIAAs and F− at high concentrations of F−. A similar phenomenon for OH− ion was observed (Fig. S32†). Based on the above observations and inferences, the synergetic effect of hydrogen bonding and anion–π interaction in the fluorescent detection of H2PDIAAs could be proposed and, the mechanism of fluorescent detection of H2PDIAAs towards anions can be inferred to be a synergetic effect (Scheme 2). To the best of our knowledge, this is the first report of PDI–amino acid derivatives as fluorescent probes to detect anions.
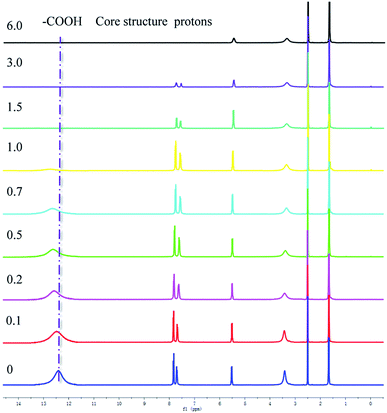 |
| Fig. 5 The 1H NMR titrations of H2PDIAla with TBAF in DMSO-d6. | |
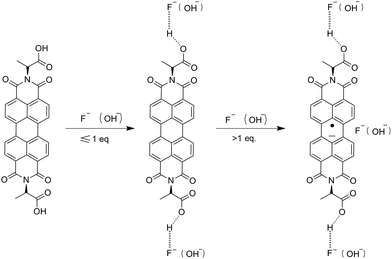 |
| Scheme 2 Proposed mechanism of H2PDIAA fluorescent probe to detect fluoride and hydroxide ions. | |
Conclusion
In summary, we have explored and discussed the fluorescent detection of anions using four types of PDI–amino acids derivatives. The result indicates that they are sensitive to F− and OH− ions in DMF solution with an obvious color change and fluorescence quenching in a short time. The detecting mechanism has been proposed according to the molecular structures and NMR titrations. In addition, we made a simple test-paper to develop its potential applications. These results constitute a promising platform for the exploitation and applications of H2PDIAAs as fluorescent probes, which are induced by the synergetic effect of hydrogen bonding and anion–π interaction, and laid a solid foundation for the PDI's development for fluorescent detectors.
Conflicts of interest
There are no conflicts to declare.
Acknowledgements
This work was financially supported by the National Natural Science Foundation of China (no. 21471017).
References
- S. Thomas, Angew. Chem., Int. Ed., 2002, 41, 48–76 CrossRef.
-
(a) E. E. Chufan, K. Kapoor and S. V. Ambudkar, Biochem. Pharmacol., 2016, 101, 40–53 CrossRef CAS PubMed;
(b) S. J. Yi, J. H. Kim, Y. J. Cho, J. Lee, T. S. Choi, D. W. Cho, C. Pac, W. S. Han, H. J. Son and S. O. Kang, Inorg. Chem., 2016, 55, 3324–3331 CrossRef CAS PubMed;
(c) J. W. Li, Y. F. Wang, J. F. Chen, Z. J. Liu, Ad. Bax and L. S. Yao, J. Am. Chem. Soc., 2016, 138, 1824–1827 CrossRef CAS PubMed.
-
(a) Z. Y. Ma, Z. J. Wang, X. Meng, Z. M. Ma, Z. J. Xu, Y. G. Ma and X. R. Jia, Angew. Chem., Int. Ed., 2016, 55, 519–522 CrossRef CAS PubMed;
(b) M. Maschke, K. Merz, O. V. Shishkin, R. I. Zubatyuk and N. M. Nolte, Struct. Chem., 2016, 27, 377–387 CrossRef CAS;
(c) R. Stefanovic, M. Ludwig, G. B. Webber, R. Atkin and A. J. Page, Phys. Chem. Chem. Phys., 2017, 19, 3297–3306 RSC.
-
(a) M. Boiocchi, L. D. Boca, D. E. Gómez, L. Fabbrizzi, M. Licchelli and E. Monzani, J. Am. Chem. Soc., 2004, 126, 16507–16514 CrossRef CAS PubMed;
(b) H. J. Kim, M. J. Ajitha, Y. Lee, J. Ryu, J. Kim, Y. Lee, Y. Jung and S. Chang, J. Am. Chem. Soc., 2014, 136, 1132–1140 CrossRef CAS PubMed;
(c) P. A. Gale and C. Caltagirone, Chem. Soc. Rev., 2015, 44, 4212–4227 RSC;
(d) D. D. Ford, D. Lehnherr, C. R. Kennedy and E. N. Jacobsen, ACS Catal., 2016, 6, 4616–4620 CrossRef CAS;
(e) A. Kaya, C. Onac, H. K. Alpoguz and A. Yilmaz, Chem. Eng. J., 2016, 283, 141–149 CrossRef CAS.
-
(a) E. Kozma, W. Mróz and F. V. Monteleone, RSC Adv., 2016, 6, 61175–61179 RSC;
(b) D. Görl, X. Zhang, V. Stepanenko and F. Würthner, Nat. Commun., 2015, 6, 7009 CrossRef PubMed;
(c) J. P. Sun, A. D. Hendsbee, A. J. Dobson, G. C. Welch and I. G. Hill, Org. Electron., 2016, 35, 151–157 CrossRef CAS.
-
(a) A. Dvivedi, S. Kumar and M. Ravikanth, Sens. Actuators, B, 2016, 24, 364–371 CrossRef;
(b) K. Rajaguru, A. Mariappan, A. J. Beneto, G. Sivaraman, S. Muthusubramanian, A. Siva and N. Bhuvanesh, ChemistrySelect, 2016, 1, 1729–1736 CrossRef CAS;
(c) K. M. Vengaian, C. D. Britto, K. Sekar, G. Sivaraman and S. Singaravadivel, Spectrochim. Acta, Part B, 2016, 235, 232–240 CAS.
-
(a) T. Anand, G. Sivaraman, M. Iniya, A. Siva and D. Chellappa, Anal. Chim. Acta, 2015, 876, 1–8 CrossRef CAS PubMed;
(b) C. A. Swamy and P. Thilagar, Inorg. Chem., 2014, 53, 2776–2786 CrossRef PubMed;
(c) B. Vidya, G. Sivaraman, R. V. Sumesh and D. Chellappa, ChemistrySelect, 2016, 1, 4024–4029 CrossRef CAS.
-
(a) Y. Zhou, J. F. Zhang and J. Yoon, Chem. Rev., 2014, 114, 5511–5571 CrossRef CAS PubMed;
(b) S. Guha and S. Saha, J. Am. Chem. Soc., 2010, 132, 17674–17677 CrossRef CAS PubMed;
(c) F. S. Goodson, D. K. Panda, S. Ray, A. Mitra, S. Guha and S. Saha, Org. Biomol. Chem., 2013, 11, 4797–4803 RSC.
- Y. S. Ma, Y. L. Zhao, F. X. Zhang, T. Y. Jiang, X. f. We, H. Shen, R. Wang and Z. Q. Shi, Spectrochim. Acta, Part B, 2017, 241, 735–743 CAS.
-
(a) Z. J. Chen, L. M. Wang, G. Zou, L. Zhang, G. J. Zhang, X. F. Cai and M. S. Teng, Dyes Pigm., 2012, 94, 410–415 CrossRef CAS;
(b) X. X. Chen, T. H. Leng, C. Y. Wang, Y. J. Shen and W. H. Zhu, Dyes Pigm., 2017, 14, 299–305 CrossRef.
- S. A. Boer, C. S. Hawes and D. R. Turner, Chem. Commun., 2014, 50, 1125–1127 RSC.
-
(a) M. J. Farooqi, M. A. Penick, J. Burch, G. R. Negrete and L. Brancaleon, Spectrochim. Acta, Part A, 2016, 153, 124–131 CrossRef CAS PubMed;
(b) E. Kozma, G. Grisci, W. Mróz, M. Catellani, A. Eckstein-Andicsovà, K. Pagano and F. Galeotti, Dyes Pigm., 2016, 125, 201–209 CrossRef CAS.
-
(a) J. John, L. Thomas, A. Kurian and S. D. George, J. Lumin., 2016, 172, 39–46 CrossRef CAS;
(b) J. Daniel, A. G. Godin, M. Palayret, B. Lounis, L. Cognet and M. Blanchard Desce, J. Phys. D: Appl. Phys., 2016, 49, 084002 CrossRef.
- J. H. Clark and J. M. Miller, Tetrahedron Lett., 1977, 18, 599–602 CrossRef.
Footnote |
† Electronic supplementary information (ESI) available: Spectra (IR, UV-vis, 1H NMR, 13C NMR, ESI-MS, fluorescence life time), or other electronic format. See DOI: 10.1039/c7ra08005k |
|
This journal is © The Royal Society of Chemistry 2017 |
Click here to see how this site uses Cookies. View our privacy policy here.