DOI:
10.1039/C7RA08136G
(Paper)
RSC Adv., 2017,
7, 44470-44473
Bicyclo[6.1.0]nonyne and tetrazine amino acids for Diels–Alder reactions†
Received
24th July 2017
, Accepted 10th September 2017
First published on 15th September 2017
Abstract
Here we report a general method for the de novo synthesis of a bicyclo[6.1.0]nonyne group containing an amino acid, and used Marfey's reagent for chiral analysis. This unnatural amino acid offered exceptional reactivity in the inverse electron demand Diels–Alder cycloaddition with tetrazine containing amino acids. The subsequent selective labeling of living cells at low dye concentrations demonstrated the usefulness of the new amino acid for future imaging studies. This work also laid the foundation for introducing this unnatural amino acid into peptides based on the solid-phase synthesis method.
Introduction
The selective and efficient labeling of biomolecules under physiological conditions is still hard to achieve with traditional biochemical or molecular biology tools. Since bioorthogonal chemical reactions can be performed without any interference to the biological system, there is considerable interest in their utilization to label and track small molecules on live cells.1 Commonly used bioorthogonal reactions that meet these criteria are Staudinger ligations,2 copper(I)-catalyzed azide–alkyne cycloaddition,3 and strain-promoted azide–alkyne cycloaddition.4 However, the derivatives of these reactions have poor water solubility or difficulty in synthesizing large quantities. Indeed, improving the reaction kinetics and the biocompatibility of the current reactions needs to be further studied, and the development of new bioorthogonal reactions with high reactivity is urgent. Currently, some reports demonstrated that tetrazines can react rapidly and specifically with strained alkenes to form stable adducts in inverse electron demand Diels–Alder (IED-DA) cycloaddition reactions.5–7 This chemistry is orders of magnitude faster than the classical cycloadditions and has been used in live cell labeling.8,9
Very recently, with the development of IED-DA cycloaddition reaction in bioorthogonal field, the method for specific labeling of proteins in complex biological systems is the currently attractive area. The most popular technique in the modification of proteins is based on the introduction of genetical unnatural amino acids by using bioorthogonal tRNA/tRNA-synthetase pairs.10,11 Inspired by recent advances in IED-DA cycloaddition reactions, the repertoire of genetically encoded chemical reactive amino acids grew considerably.12 And a set of new dienophilic amino acids were synthesized and incorporated into proteins in E. coli and mammalian cells through suppressing the amber stop codon.13–15
Many of the synthetic unnatural amino acids were connected by a ligand or a linker between these functional tags and natural amino acid groups.16–18 This method provides a easy way to synthesis a wide range of unnatural amino acids with diverse side chains, but this kind amino acids also have many deficiencies, such as unstability, difficult to handle, and complex structure may affecting the function. While the de novo synthesis of unnatural amino acids provides another way to overcome this defects, and many method had developed.19–21 But the de novo synthesis of unnatural amino acids for IED-DA reactions was almost no literature reports. The toolbox of unnatural amino acids was further expanded by our recent report, in which tetrazine group was connected directly to the benzene ring of phenylalanine and a tetrazine-containing amino acid was synthesized.22 The tetrazine amino acid has shown to be stable enough to be used for peptide modification and live cell labeling.
In this study, based on the known diversity of hydrophilic dienes, we sought to de novo synthesize an unnatural amino acid containing a hydrophilic diene group. As a reaction partner for the tetrazines, bicyclo[6.1.0]nonyne (BCN) group was selected as a model dienophile substrate for the unnatural amino acid synthesis, which possessed a higher reactivity than many hydrophilic dienes because of its enhanced cyclopropane fusion reactivity.23 Moreover, unlike other strained alkenes, BCN reacted with tetrazine to give a single product of defined stereochemistry. Besides, the compound was easily obtained in a highly straightforward process through cyclopropanation of 1,5-cyclooctadiene.
Results and discussion
Synthesis and characterizations of L-BCN-containing amino acid
Just as the literature reported,23–25 compound 6 can be synthesized in four steps started by the dropwise addition of ethyl diazoacetate to excess 1,5-cyclooctadiene in the presence of rhodium acetate, to give a mixture of diastereomeric compounds exo-3 and endo-3. Next, the individual stereoisomer exo-3 was selected because of its higher yield, and was converted into the corresponding hydroxyalkyne product by the reduction of the ester group, bromination, and elimination, to give the compound BCN group.
Compound 11 can be synthesized in five steps started by the oxidation of alcohol of BCN to the aldehyde under Swern conditions,26 followed by the Strecker reaction to nitrile.27 One key step in the reaction was the hydrolysis of the intermediate nitrile 8 with 2 M NaOH to afford BCN amino acid. While by contrast, in experimenting we found that the hydrolysis of the intermediate nitrile 8 with 6 M HCl and 10% aqueous H2SO4 led to an entirely conversion of 8 into by-product ketone amino acid. Attempts of enantioselective hydrolysis of 8 with recombinant nitrilase from Arabidopsis thaliana (EC 3.5.5.1) failed. At last, the amino acid 9 was acetylated with Ac2O and the resulting Ac-BCN was enantiomerically resolved with kidney acylase I to produce L-BCN amino acid (Scheme 1).28
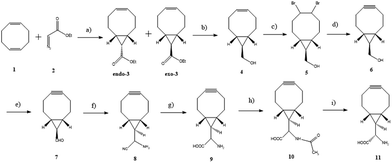 |
| Scheme 1 Synthetic route of L-BCN amino acid 11. (a) Rh2(OAc)4 (0.5% eq.), rt, 25 h. (b) LiAlH4 (0.8 eq.), 0 °C, 30 min, 93.6%. (c) Br2 (1.1 eq.), 0 °C, 20 min, 92.0%. (d) KOtBu (3 eq.), 80 °C, 2h, 65.0%. (e) (COCl)2 (1.3 eq.), DMSO (2.5 eq.), Et3N (6 eq.), −78 °C, 5 h, 95%. (f) (LiHMDS) (1.0 M in THF, 1.2 eq.), −40 °C, acetone cyanohydrin (2 eq.), rt, 12 h, 78%. (g) 2 M NaOH, 80 °C, 2h, 38.1%. (h) Ac2O (2.5 eq.), 1 M NaOH, rt, 2 h, 54%. (i) kidney acylase I, 37 °C, 2 days, 25%. | |
To test whether the configuration of the product was single, the Nα-(2,4-dinitro-5-fluorophenyl)-L-alanine amide (FDAA) reagent was selected for chiral analysis,29 and the results confirmed the enantiomeric purity of the compound. Compound 11 was sufficiently stable for prolonged storage at 20 °C and did not undergo any structural changes upon stirring in the presence of PBS solution.
Synthesis and characterizations of tetrazine-containing amino acid
Next, we aimed to synthesize tetrazine-containing amino acids (Scheme 2). We all knew that 1,2,4,5-tetrazines were pretty reactive toward water, which made them unsuitable for bioconjugation. While, the stability of tetrazines can be dramatically improved by the substitution with aromatic groups. Our previously synthesized tetrazine amino acid 13 showed excellent stability in PBS and biological media, with little or no decomposition after prolonged exposure at room temperature, but was unstable in 20% piperidine/DMF.22 In general, tetrazines, which was substituted with electron donating groups like alkyl, tended to be more stable with slower cycloaddition kinetics.30 According to the above summarized theory, we added a methyl in tetrazine of compound 13 to give another tetrazine amino acid (S)-2-amino-3-(4-(6-methyl-1,2,4,5-tetrazin-3-yl) phenyl) propanoic acid 14, which demonstrated a good balance of solution stability and fast reaction kinetics.
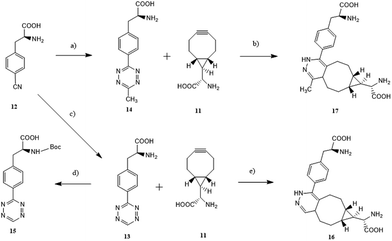 |
| Scheme 2 Synthetic route of tetrazine amino acid 13 and 14, and Boc-protected tetrazine amino acid 15, and the IED-DA reaction of 13 and 14 with L-BCN amino acid 11 (a) (i) acetamidine hydrochloride (4 eq), N2H4 (40 eq.), S (1 eq.), rt, 22 h; (ii) CH3COOH, NaNO2 (5 eq.), 0 °C, 30 min, 64.9%. (b) Phosphate buffered solution (PBS), rt, 30 min. (c) (i) Formamidine acetate (4 eq.), N2H4 (40 eq.), S (1 eq.), rt, 22 h; (ii) CH3COOH, NaNO2 (5 eq.), 0 °C, 30 min, 64.9%. (d) Boc2O (2 eq.), 1 : 1 dioxane/H2O (V/V), NaHCO3 (2.5 eq.), 0 °C, 8 h, 72.3%. (e) Phosphate buffered solution (PBS), rt, 30 min. | |
The tetrazine amino acid 14 was synthesized in a way similar to compound 13. Compound 14 was prepared starting from the commercially available 4-cyano-L-phenylalanine with acetamidine hydrochloride and anhydrous hydrazine in the presence of elemental sulfur. The initial product dihydrotetrazine derivative was oxidized to the tetrazine by treating with sodium nitrite in acetic acid. Moreover, compound 14 showed excellent stability in 20% piperidine/DMF with little or no decomposition observed after prolonged exposure at room temperature (see Fig. S2†), a prerequisite for peptide synthesis via an Fmoc synthetic strategy.
Kinetic experiments between BCN-containing amino acid and tetrazine-containing amino acids
We have synthesized a BCN-containing amino acid and tetrazine-containing amino acids. Alternatively, we sought to directly select the reaction of the BCN amino acid and tetrazine amino acids as bioorthogonal chemical “handles” for amino acid coupling via the IED-DA reactions. The rate constants of the reactions between BCN amino acid and tetrazines, a prerequisite in vivo applications, was determined by manual mixing under the pseudo-first-order conditions. By following the exponential decay of the tetrazine absorbance at 523 nm upon reaction with a 10–100 fold excess of BCN, we determined the rate constants of the reactions between BCN amino acid and tetrazine 13 as k2 = (437 ± 13) M−1 s−1 (see Fig. S3†). Under the same conditions, the rate constants of the reactions between BCN amino acid and tetrazine 14 was determined with UV/vis spectroscopy following the decay in the absorption of tetrazine derivative at 527 nm. The results showed that k2 = (1.45 ± 0.05) M−1 s−1 (see Fig. S4†). Obviously, the reactions between two tetrazine amino acids and BCN amino acid were so reactive and suitable for rapid biological labeling.
L-BCN-containing amino acid in the epidermoid carcinoma cells labeling
Specific labeling of living cell was one of the most valuable applications in bioorthogonal chemistry.31,32 To prove the possible biological application of the L-BCN amino acid, we chose to label epidermal growth factor receptors (EGFR), which played an important role in cancer-cell signaling and was considered as the key target for therapeutic inhibition, with the anti-EGFR monoclonal antibody cetuximab. Commercially available cetuximab was marked with tetrazine amino acid derivative and fluorescein dye rhodamine group. A431 epidermoid carcinoma cells can overexpress EGFR, which bound antibody cetuximab specifically and efficiently.33
Based on the commercial availability of numerous amine-reactive fluorophores, the BCN-fluorophore probes were utilized. And the free amine of BCN amino acid 11 was conjugated to a commercially available near-infrared (NIR) fluorophore Cy5.5 to label cells pretargeted with tetrazine-bearing antibodies. Furthermore, we chose the more reactive Boc-protected tetrazine 13 for the cell-labeling studies, which was serum-stable and reacted rapidly with strained dienophiles BCN.
A431 cancer cells were first incubated with modified cetuximab for 45 min in serum. Then, the cells were washed and incubated with BCN-Cy5.5 for 10 min in 100% fetal bovine serum (FBS). After being rewashed, the cells were imaged immediately by confocal microscopy (see Fig. 1). We can observe cellular changes in rhodamine channel and NIR channel, respectively. A431 cells were incubated with rhodamine modified cetuximab and BCN-Cy5.5 as control experiment 1. A431 cells were incubated with tetrazine modified cetuximab and BCN-Cy5.5 as control experiment 2. The results showed that the antibody could be visualized clearly in rhodamine channel and the covalently bound tetrazine-VT680 could be monitored apparently in the NIR channel. Cells incubated with the control antibody, which contained rhodamine but not tetrazine, showed no NIR labeling after exposure to 11. While control experiment 2, the antibody containing tetrazine instead of rhodamine, resulted in no rhodamine fluorescence (see Fig. 2), suggesting that BCN-Cy5.5 conjugate did not react with other cellular components.
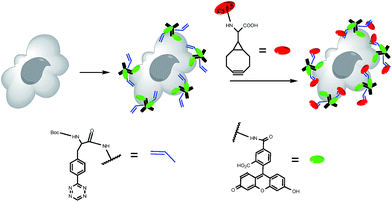 |
| Fig. 1 Compound 11 reacted with Boc-protected tetrazine amino acid to label cancer cells. Cancer cells A431, which overexpressed EGFR, were exposed to the Cetuximab antibodies modified with tetrazine and 5-carboxyfluorescein (green). In the next step, the pretargeted cells were labeled with a BCN bearing a fluorophore such as Cy5.5 (red). | |
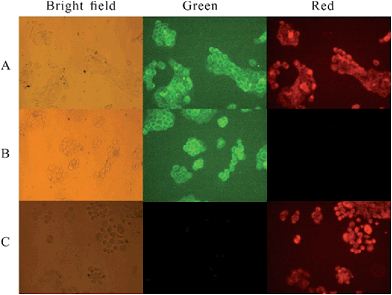 |
| Fig. 2 (A) Fluorescent microscope images of A431 epidermoid carcinoma cells after pretargeting Cetuximab antibodies modified with 5-carboxyfluorescein and tetrazine and subsequent labeling with BCN-Cy5.5. From left to right, bright field and fluorescence from Rhodamine channel and Near-IR channel, respectively. Images of control experiments were also taken. (B) Control experiment with 5-carboxyfluorescein modified cetuximab and BCN-Cy5.5. (C) Control experiment with tetrazine modified cetuximab and BCN-Cy5.5. | |
These experiments demonstrated the specificity and sensitivity of the reaction between BCN-containing amino acid and tetrazine-modified antibody in the complex biological living cells. Consequently, we concluded that this BCN-containing amino acid of using IED-DA reaction was suitable for in vitro labeling experiments, and might also provide an effective way to label cells in the complex intracellular environment.
Experimental
All the experimental procedures and supporting figures are reported in the ESI.†
Conclusions
In conclusion, we reported a general method for de novo synthesis of a BCN group containing amino acid, and used Marfey's reagent for chiral analysis, which laid the foundation for being introduced into peptides based on the solid-phase synthesis method. Moreover, as a reaction partner for the BCN amino acid, we also de novo synthesized another tetrazine containing amino acid 14 in a way similar to compound 13, which was synthesized in our previous work. We demonstrated that the reactions of BCN amino acid and tetrazine amino acids can be used as a bioorthogonal chemical “handle” for amino acid coupling via the IED-DA reaction. Finally, the high reaction rate of BCN amino acid and tetrazine amino acids was suitable for cancer cell labeling under physiological conditions. Our future efforts will focus on the incorporation of this BCN amino acid site-directly in peptide modification.
Conflicts of interest
There are no conflicts to declare.
Acknowledgements
The authors want to thank Prof. Lingyun Mu for the Fluorescent microscope experiments.
References
- E. M. Sletten and C. R. Bertozzi, Angew. Chem., Int. Ed., 2009, 48, 6974–6998 CrossRef CAS PubMed.
- B. L. Nilsson, L. L. Kiessling and R. T. Raines, Org. Lett., 2000, 2, 1939–1941 CrossRef CAS PubMed.
- V. V. Rostovtsev, L. G. Green, V. V. Fokin and K. B. Sharpless, Angew. Chem., 2002, 114, 2708–2711 CrossRef.
- J. C. Jewett, E. M. Sletten and C. R. Bertozzi, J. Am. Chem. Soc., 2010, 132, 3688–3690 CrossRef CAS PubMed.
- M. L. Blackman, M. Royzen and J. M. Fox, J. Am. Chem. Soc., 2008, 130, 13518–13519 CrossRef CAS PubMed.
- L. Törk, G. Jiménez-Osés, C. Doubleday, F. Liu and K. Houk, J. Am. Chem. Soc., 2015, 137, 4749–4758 CrossRef PubMed.
- S. Kotha, A. S. Chavan and D. Goyal, ACS Comb. Sci., 2015, 17, 253–302 CrossRef CAS PubMed.
- N. K. Devaraj, R. Weissleder and S. A. Hilderbrand, Bioconjugate Chem., 2008, 19, 2297–2299 CrossRef CAS PubMed.
- H. Wu, J. Yang, J. Šečkutė and N. K. Devaraj, Angew. Chem., Int. Ed., 2014, 53, 5805–5809 CrossRef CAS PubMed.
- C. H. Kim, J. Y. Axup and P. G. Schultz, Curr. Opin. Chem. Biol., 2013, 17, 412–419 CrossRef CAS PubMed.
- W. H. Zhang, G. Otting and C. J. Jackson, Curr. Opin. Struct. Biol., 2013, 23, 581–587 CrossRef CAS PubMed.
- T. Plass, S. Milles, C. Koehler, J. Szymański, R. Mueller, M. Wießler, C. Schultz and E. A. Lemke, Angew. Chem., Int. Ed., 2012, 51, 4166–4170 CrossRef CAS PubMed.
- E. Kaya, M. Vrabel, C. Deiml, S. Prill, V. S. Fluxa and T. Carell, Angew. Chem., Int. Ed., 2012, 51, 4466–4469 CrossRef CAS PubMed.
- J. L. Seitchik, J. C. Peeler, M. T. Taylor, M. L. Blackman, T. W. Rhoads, R. B. Cooley, C. Refakis, J. M. Fox and R. A. Mehl, J. Am. Chem. Soc., 2012, 134, 2898–2901 CrossRef CAS PubMed.
- Z. Yu, Y. Pan, Z. Wang, J. Wang and Q. Lin, Angew. Chem., Int. Ed., 2012, 51, 10600–10604 CrossRef CAS PubMed.
- K. Lang, L. Davis, J. Torres-Kolbus, C. Chou, A. Deiters and J. W. Chin, Nat. Chem., 2012, 4, 298–304 CrossRef CAS PubMed.
- S. Schneider, M. J. Gattner, M. Vrabel, V. Flügel, V. López-Carrillo, S. Prill and T. Carell, ChemBioChem, 2013, 14, 2114–2118 CrossRef CAS PubMed.
- K. Lang, L. Davis, S. Wallace, M. Mahesh, D. J. Cox, M. L. Blackman, J. M. Fox and J. W. Chin, J. Am. Chem. Soc., 2012, 134, 10317–10320 CrossRef CAS PubMed.
- S. Kotha, Acc. Chem. Res., 2003, 34, 342–351 CrossRef PubMed.
- M. A. T. Blaskovich, J. Med. Chem., 2016, 59, 10807–10836 CrossRef CAS PubMed.
- K. Undheim, Amino Acids, 2008, 34, 357–402 CrossRef CAS PubMed.
- Z. Ni, L. Zhou, X. Li, J. Zhang and S. Dong, PLoS One, 2015, 10, e0141918 Search PubMed.
- J. Dommerholt, S. Schmidt, R. Temming, L. J. Hendriks, F. P. Rutjes, J. van Hest, D. J. Lefeber, P. Friedl and F. L. Van Delft, Angew. Chem., Int. Ed., 2010, 49, 9422–9425 CrossRef CAS PubMed.
- M. T. Taylor, M. L. Blackman, O. Dmitrenko and J. M. Fox, J. Am. Chem. Soc., 2011, 133, 9646–9649 CrossRef CAS PubMed.
- A. Borrmann, S. Milles, T. Plass, J. Dommerholt, J. M. Verkade, M. Wießler, C. Schultz, J. van Hest, F. L. van Delft and E. A. Lemke, ChemBioChem, 2012, 13, 2094–2099 CrossRef CAS PubMed.
- A. J. Mancuso, S.-L. Huang and D. Swern, J. Org. Chem., 1978, 43, 2480–2482 CrossRef CAS.
- G. H. Chu, M. Gu, B. Gerard and R. E. Dolle, Synth. Commun., 2004, 34, 4583–4590 CrossRef CAS.
- S. Dong, L. Merkel, L. Moroder and N. Budisa, J. Pept. Sci., 2008, 14, 1148–1150 CrossRef CAS PubMed.
- R. Bhushan and H. Brückner, Amino Acids, 2004, 27, 231–247 CrossRef CAS PubMed.
- M. R. Karver, R. Weissleder and S. A. Hilderbrand, Bioconjugate Chem., 2011, 22, 2263–2270 CrossRef CAS PubMed.
- N. K. Devaraj and R. Weissleder, Acc. Chem. Res., 2011, 44, 816–827 CrossRef CAS PubMed.
- K. Lang and J. W. Chin, Chem. Rev., 2014, 114, 4764–4806 CrossRef CAS PubMed.
- H.-S. Han, N. K. Devaraj, J. Lee, S. A. Hilderbrand, R. Weissleder and M. G. Bawendi, J. Am. Chem. Soc., 2010, 132, 7838–7839 CrossRef CAS PubMed.
Footnote |
† Electronic supplementary information (ESI) available. See DOI: 10.1039/c7ra08136g |
|
This journal is © The Royal Society of Chemistry 2017 |
Click here to see how this site uses Cookies. View our privacy policy here.