DOI:
10.1039/C7RA09214H
(Paper)
RSC Adv., 2017,
7, 44132-44135
Synthesis of pyrrolo[1,2-a]quinoxalines via copper or iron-catalyzed aerobic oxidative carboamination of sp3C–H bonds†
Received
21st August 2017
, Accepted 6th September 2017
First published on 12th September 2017
Abstract
An aerobic oxidative carboamination of sp3C–H bonds with 2-(1H-pyrrol-1-yl)anilines has been developed. The oxidative carboamination processes utilized simple and readily available starting materials to produce pyrrolo[1,2-a]quinoxalines in good to moderate yields. The transformations also featured inexpensive metal catalysts (copper or iron) and a green oxidant (O2).
The pyrrolo[1,2-a]quinoxalines are an important class of heterocyclic compounds which are present in various biologically active agents. For example, some substituted pyrrolo[1,2-a]quinoxaline derivatives promised utilization for novel and highly potent 5-HT3 receptor agonists.1 And 4-substituted pyrrolo[1,2-a]quinoxalines could specifically inhibit the D-loop activity of RAD51.2a Recently, Mai, Steegborn et al. presented a good work about using pyrrolo[1,2-a]quinoxaline derivatives as Sirt6 activators.2b Furthermore, many pyrrolo[1,2-a]quinoxaline derivatives have been proven to possess other biological activities, including antimalarial activity,3 antitumor activity,4 HIV-1 reverse transcriptase inhibitors,5 human protein kinase CK2 inhibitors,6 PARP-1 inhibitors,7 non-peptide glucagon receptor antagonists,8 and so on. They are also used as fluorescent probes for amyloid fibril.9 In 1965, Cheesman and Tuck groups firstly presented the method for the synthesis of pyrrolo[1,2-a]quinoxaline compounds via the reactions between 2-(1H-pyrrol-1-yl)anilines and formic acid under reflux conditions (Scheme 1a).10 Afterward, many protocols for the preparation of pyrrolo[1,2-a]quinoxalines have been developed one after another.11 Among them, the cyclization reactions involving 2-(1H-pyrrol-1-yl)anilines have always been the most active part. And it was most reported to utilize 2-(1H-pyrrol-1-yl)anilines and aldehydes as the starting materials (Scheme 1b).11e–g However, these methods suffered from some drawbacks such as tedious procedure, strong oxidant, poor substrate applicability, and so on. In 2017, Jiang and co-workers reported a green aerobic oxidative synthesis of pyrrolo[1,2-a]quinoxalines from simple alcohols (Scheme 1c).11h However, the transformation was just suitable for alkyl alcohols, and required alcohols as solvents and high reaction temperature. Subsequently, a method which utilized DMSO not only as solvent but also as the substrate for the synthesis of pyrrolo[1,2-a]quinoxalines was reported by Ma (Scheme 1d).11i This was a good transformation but only C4 no-substituted products were obtained. Therefore, it is highly desirable to develop new method for the construction of this important skeleton.
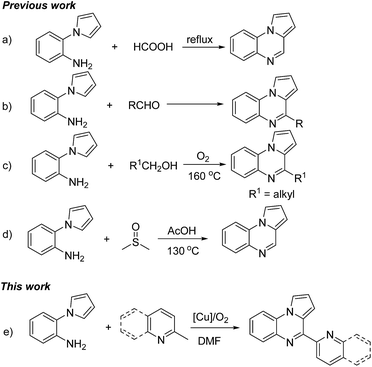 |
| Scheme 1 Synthesis of pyrrolo[1,2-a]quinoxalines from 1-(2-aminophenyl)pyrrole. | |
On the other hand, direct transformations of inert chemical bonds especially sp3C–H bonds play an important role in sustainable chemistry.12 A copper/O2 system could be viewed as an ideal reaction system for carbon–carbon and carbon–heteroatom bonds formation because copper catalysts are inexpensive and low-toxic and molecular oxygen as an oxidant is easily available and environment friendly.13 Recently, the aerobic copper-catalyzed transformations of methylhetarenes have attracted increasing attention, such as esterification,14a amidation,14b,c oxygenation,14d,e oxidative cross-coupling.14f–h However, it remains scarce that applying these reactions to construct N-heterocycles.14i,j Herein, we disclose a new aerobic copper-catalyzed cyclization reaction of 2-methylpyridine/quinoline with 2-(1H-pyrrol-1-yl)anilines for synthesis of pyrrolo[1,2-a]quinoxalines (Scheme 1e).
Initially, the reaction of 2-(1H-pyrrol-1-yl)aniline 1a (0.3 mmol) with 2-methylpyridine 2a (0.6 mmol) in 3 mL solvent for 12 h under a O2 atmosphere was chosen as the model reaction to examine various reaction parameters and the results were summarized in Table 1. Our experiment began by reacting 1a and 2a in DMF at 120 °C in the presence of Cu(OTf)2 (0.06 mmol) and TFA (0.3 mmol). To our delight, the target product 4-(pyridin-2-yl)pyrrolo[1,2-a]quinoxaline (3a) was obtained in 48% yield (entry 1). Several other copper salts were then screened, including Cu(OAc)2, CuCl2, CuBr2, Cu(NO3)2, CuCl, CuI, CuBr and CuCN. Our experimental results showed that all copper catalysts could catalyze the transformation to some extent and Cu(OAc)2 was most efficient (entries 2–9). Among the solvents tested, DMF was found to be the most effective in comparison to DMSO, PhCl, and toluene (entries 10–12). Different acids, such as AcOH, Ph2PO2H and TfOH were investigated, and the results indicated that they were no better than TFA (entries 13–15). When the reaction was carried out in the absence of a copper catalyst, no product was formed and if the reaction proceeded without the acid, the yield of the desired heterocyclic product was very low (entries 16–17). The increase or decrease of reaction temperature led to a diminished yield (entries 18–19). Lower yield was obtained when the reaction was conducted in the oper air (entry 20). Furthermore, no product was obtained under N2 atmosphere (entry 21). Thus, the optimized reaction system for this aerobic oxidative carboamination reaction was: 1a (0.3 mmol), 2a (0.6 mmol), Cu(OAc)2 (0.06 mmol), TFA (0.3 mmol), and DMF (3 mL) under O2 atmosphere at 120 °C for 12 h.
Table 1 Optimization of the reaction conditionsa
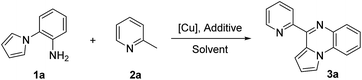
|
Entry |
[Cu] |
Additive |
Solvent |
Yield (%) |
Reaction were performed with 1a (0.3 mmol), 2a (0.6 mmol), [Cu] (0.06 mmol), acid (0.3 mmol) in solvent (3.0 mL) under O2 atmosphere for 12 h. Isolated yield. n.d. = not determined. The reaction temperature was 110 °C. The reaction temperature was 130 °C. The reaction was under air. The reaction was under N2 atmosphere. |
1 |
Cu(OTf)2 |
TFA |
DMF |
48 |
2 |
Cu(OAc)2 |
TFA |
DMF |
78 |
3 |
CuCl2 |
TFA |
DMF |
64 |
4 |
CuBr2 |
TFA |
DMF |
67 |
5 |
Cu(NO3)2 |
TFA |
DMF |
70 |
6 |
CuCl |
TFA |
DMF |
65 |
7 |
CuI |
TFA |
DMF |
45 |
8 |
CuBr |
TFA |
DMF |
54 |
9 |
CuCN |
TFA |
DMF |
52 |
10 |
Cu(OAc)2 |
TFA |
DMSO |
63 |
11 |
Cu(OAc)2 |
TFA |
PhCl |
n.d. |
12 |
Cu(OAc)2 |
TFA |
Toluene |
n.d. |
13 |
Cu(OAc)2 |
AcOH |
DMF |
37 |
14 |
Cu(OAc)2 |
Ph2PO2H |
DMF |
41 |
15 |
Cu(OAc)2 |
TfOH |
DMF |
56 |
16 |
— |
TFA |
DMF |
n.d. |
17 |
Cu(OAc)2 |
— |
DMF |
15 |
18b |
Cu(OAc)2 |
TFA |
DMF |
68 |
19c |
Cu(OAc)2 |
TFA |
DMF |
59 |
20d |
Cu(OAc)2 |
TFA |
DMF |
64 |
21e |
Cu(OAc)2 |
TFA |
DMF |
n.d. |
With the optimal conditions established (Table 1, entry 2), we started to examine the reaction scope of the present transformation and the results were summarized in Table 2. Various 2-methylpyridine reacted with 2-(1H-pyrrol-1-yl)aniline were firstly examined and all of the reactions afforded the corresponding pyrrolo[1,2-a]quinoxalines in moderate yields (Table 2, 3a–3e). When 2-methylquinoline was used as substrate, the yield of product was up to 85% (Table 2, 3f). Similarly, 2,6-dimethylquinoline, 6-fluoro-2-methylquinoline and 7-fluoro-2-methylquinoline also gave the expected cyclization products 3g, 3h and 3i in 72%, 58% and 54% yields, respectively. Finally, the reaction of 5-methyl-2-(1H-pyrrol-1-yl)aniline with 2-methylquinoline could produce 7-methyl-4-(quinolin-2-yl)pyrrolo[1,2-a]quinoxaline in 67% yield (Table 2, 3j).
Table 2 Substrate scope of pyrrolo[1,2-a]quinoxalines via copper-catalyzed cyclization reactiona
Reactions were performed with 1 (0.3 mmol), 2 (0.6 mmol), Cu(OAc)2 (20 mol%), TFA (0.3 mmol) and DMF (3 mL) at 120 °C under O2 atmosphere for 12 h. Yields referred to isolated yields. |
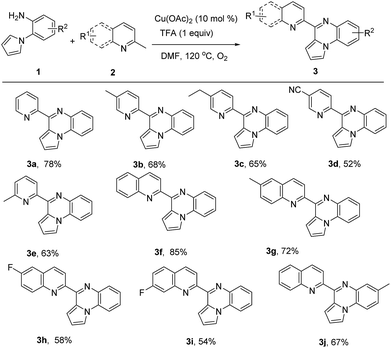 |
It was amazing that the product pyrrolo[1,2-a]quinoxaline 4a was formed when we treated 1a with Fe catalyst in DMSO, which revealed that DMSO also served as C1 source in the oxidative cyclization reaction. Compared with previous work,11i our reaction proceeded with lower reaction temperature. Where after, we optimized the reaction conditions based on Fe catalyst and the best reaction condition were as follows: 1a (0.3 mmol), Fe(0) (20 mol%), AcOH (0.3 mmol) in DMSO (3 mL) at 100 °C for 12 h (see the ESI† for details). Then, various 2-(1H-pyrrol-1-yl)aniline 1 were examined in the cyclization reaction and the results were summarized in Table 3. Different substituted 2-(1H-pyrrol-1-yl)aniline could be converted into the corresponding pyrrolo[1,2-a]quinoxaline in moderate to good yields, and electron-withdrawing groups did not show a positive effect on our reaction (Table 3, 4a–4e).
Table 3 Substrate scope of pyrrolo[1,2-a]quinoxalines via iron-catalyzed cyclization reactiona
Reaction conditions: 1 (0.3 mmol), Fe(0) (20 mol%), AcOH (0.3 mmol), and DMSO (3 mL) at 100 °C under air atmosphere for 12 h. Yields referred to isolated yields. |
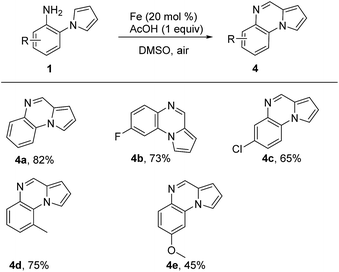 |
To gain more insight into the mechanism, some control experiments shown in Scheme 2 were carried out. Firstly, the reaction of 1a and 2a under standard conditions was interrupted after 1.0 h to analyze the intermediates (Scheme 2, eqn (1)). Except for the generation of the product 3a (32% yield), 2-pyridinecarboxaldehyde 5a was obtained in 10% yield. When 2-pyridinecarboxaldehyde 5a was allowed to react with 1a, the pyrrolo[1,2-a]quinoxalins 3a was formed in 81% yield (Scheme 2, eqn (2)). These results indicated that 5a perhaps served as the key intermediate in the cyclization reaction. When we added a radical-trapping reagent (TEMPO) or a radical inhibitor (BHT) to the reaction system, no product was detected. These observations demonstrated that there should be a radical pathway in this reaction.
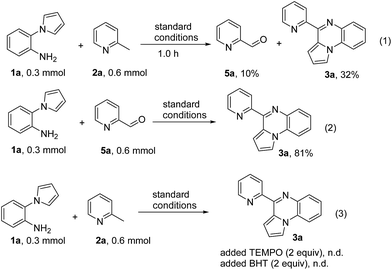 |
| Scheme 2 Control experiments. | |
According to previous studies11,14 and our experimental results above, we proposed the mechanism shown in Scheme 3. Initially, 1a isomerizes to a nonaromatic enamine intermediate A in the presence of acid.14a,b,i,j Where after, intermediate A reacts with the copper species to afford intermediate B and further combines with the oxygen to generates peroxycopper intermediate C.14b,j Intermediate C transforms to intermediate D and subsequent elimination of CunOH produces aldehyde 5a.14b,j DMSO could decompose and produce CH2O in the presence of Fe catalyst with O2.11i,14k,14l The imine intermediate E or G is formed by dehydration condensation of 5a or CH2O with 1a, which cyclize to generate intermediate F or H through intramolecular Mannich reaction.11g Finally, oxidation of intermediate F or H provides the final product 3a or 4a.11e–g
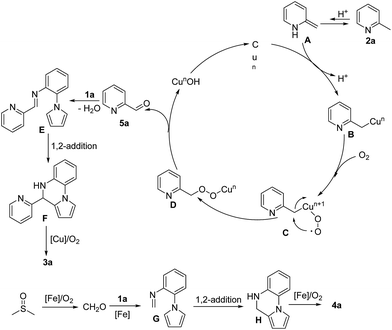 |
| Scheme 3 Possible reaction mechanism. | |
In conclusion, we have developed a copper or iron-catalyzed direct aerobic oxidative carboamination of sp3C–H bonds with 2-(1H-pyrrol-1-yl)aniline. These methods afforded a novel approach for the construction of biologically important pyrrolo[1,2-a]quinoxaline skeleton from readily available materials using inexpensive metal catalysts and green oxidant (O2). Further research for the mechanism and the synthetic applications are ongoing in our laboratory.
Conflicts of interest
There are no conflicts to declare.
Acknowledgements
The authors thank the National Natural Science Foundation of China (21702096), the High-level Talent Introduction Foundation of Southern Medical University (C1033520), and the Science and Technology Program of Guangdong Province (2015A010105015) for financial support.
Notes and references
-
(a) G. Campiani, A. Cappelli, V. Nacci, M. Anzini, S. Vomero, M. Hamon, A. Cagnotto, C. Fracasso, C. Uboldi, S. Caccia, S. Consolo and T. Mennini, J. Med. Chem., 1997, 40, 3670 CrossRef CAS PubMed;
(b) H. Prunier, S. Rault, J.-C. Lancelot, M. Robba, P. Renard, P. Delagrange, B. Pfeiffer, D.-H. Caignard, R. Misslin, B. Guardiola-Lemaitre and M. Hamon, J. Med. Chem., 1997, 40, 1808 CrossRef CAS PubMed;
(c) E. Morelli, S. Gemma, R. Budriesi, G. Campiani, E. Novellino, C. Fattorusso, B. Catalanotti, S. S. Coccone, S. Ros, G. Borrelli, V. Kumar, M. Persico, I. Fiorini, V. Nacci, P. Ioan, A. Chiarini, M. Hamon, A. Cagnotto, T. Mennini, C. Fracasso, M. Colovic, S. Caccia and S. Butini, J. Med. Chem., 2009, 52, 3548 CrossRef CAS PubMed.
-
(a) W. Lv, B. Budke, M. Pawlowski, P. P. Connell and A. P. Kozikowski, J. Med. Chem., 2016, 59, 4511 CrossRef CAS PubMed;
(b) W. You, D. Rotili, T.-M. Li, C. Kambach, M. Meleshin, M. Schutkowski, K. F. Chua, A. Mai and C. Steegborn, Angew. Chem., Int. Ed., 2017, 56, 1007 CrossRef CAS PubMed.
-
(a) J. Guillon, P. Grellier, M. Labaied, P. Sonnet, J.-M. Léger, R. Déprez-Poulain, I. Forfar-Bares, P. Dallemagne, N. LemaÎtre, F. Péhourcq, J. Rochette, C. Sergheraert and C. Jarry, J. Med. Chem., 2004, 47, 1997 CrossRef CAS PubMed;
(b) J. Guillon, E. Mouray, S. Moreau, C. Mullié, I. Forfar, V. Desplat, S. Belisle-Fabre, N. Pinaud, F. Ravanello, A. Le-Naour, J.-M. Léger, G. Gosmann, C. Jarry, G. Déléris, P. Sonnet and P. Grellier, Eur. J. Med. Chem., 2011, 46, 2310 CrossRef CAS PubMed;
(c) J. Guillon, S. Moreau, E. Mouray, V. Sinou, I. Forfar, S. B. Fabre, V. Desplat, P. Millet, D. Parzy, C. Jarry and P. Grellier, Bioorg. Med. Chem., 2008, 16, 9133 CrossRef CAS PubMed.
-
(a) F. Grande, F. Aiello, O. D. Grazia, A. Brizzi, A. Garofalo and N. Neamati, Bioorg. Med. Chem., 2007, 15, 288 CrossRef CAS PubMed;
(b) P. V. Babu, S. Mukherjee, G. S. Deora, K. S. Chennubhotla, R. Medisetti, S. Yellanki, P. Kulkarni, S. Sripelly, K. V. L. Parsa, K. Chatti, K. Mukkanti and M. Pal, Org. Biomol. Chem., 2013, 11, 6680 RSC.
- G. Campiani, F. Aiello, M. Fabbrini, E. Morelli, A. Ramunno, S. Armaroli, V. Nacci, A. Garofalo, G. Greco, E. Novellino, G. Maga, S. Spadari, A. Bergamini, L. Ventura, B. Bongiovanni, M. Capozzi, F. Bolacchi, S. Marini, M. Coletta, G. Guiso and S. Caccia, J. Med. Chem., 2001, 44, 305 CrossRef CAS PubMed.
- J. Guillon, M. L. Borgne, C. Rimbault, S. Moreau, S. Savrimoutou, N. Pinaud, S. Baratin, M. Marchivie, S. Roche, A. Bollacke, A. Pecci, L. Alvarez, V. Desplat and J. Jose, Eur. J. Med. Chem., 2013, 65, 205 CrossRef CAS PubMed.
- J. Miyashiro, K. W. Woods, C. H. Park, X. Liu, Y. Shi, E. F. Johnson, J. J. Bouska, A. M. Olson, Y. Luo, E. H. Fry, V. L. Giranda and T. D. Penning, Bioorg. Med. Chem. Lett., 2009, 19, 4050 CrossRef CAS PubMed.
- J. Guillom, P. Dallemagne, B. Pfeiffer, P. Renard, D. Manechez, A. Kervran and S. Rault, Eur. J. Med. Chem., 1998, 33, 293 CrossRef.
- S. Gemma, L. Colombo, G. Forloni, L. Savini, C. Fracasso, S. Caccia, M. Salmona, M. Brindisi, B. P. Joshi, P. Tripaldi, G. Giorgi, O. Taglialatela-Scafati, E. Novellino, I. Fiorini, G. Campiani and S. Butini, Org. Biomol. Chem., 2011, 9, 5137 CAS.
- G. W. H. Cheeseman and B. Tuck, Chem. Ind., 1965, 1382 CAS.
-
(a) Z. Zhang, J. Li, G. Zhang, N. Ma, Q. Liu and T. Liu, J. Org. Chem., 2015, 80, 6875 CrossRef CAS PubMed;
(b) H. Liu, T. Duan, Z. Zhang, C. Xie and C. Ma, Org. Lett., 2015, 17, 2932 CrossRef CAS PubMed;
(c) J. T. Reeves, D. R. Fandrick, Z. Tan, J. J. Song, H. Lee, N. K. Yee and C. H. Senanayake, J. Org. Chem., 2010, 75, 992 CrossRef CAS PubMed;
(d) M. F. Pereira and V. Thiéry, Org. Lett., 2012, 14, 4754 CrossRef CAS PubMed;
(e) A. Preetam and M. Nath, RSC Adv., 2015, 5, 21843 RSC;
(f) A. K. Verma, R. R. Jha, V. K. Sankar, T. Aggarwal, R. P. Singh and R. Chandra, Eur. J. Org. Chem., 2011, 6998 CrossRef CAS;
(g) C. Wang, Y. Li, J. Zhao, B. Cheng, H. Wang and H. Zhai, Tetrahedron Lett., 2016, 57, 3908 CrossRef CAS;
(h) J. Li, J. Zhang, H. Yang, Z. Gao and G. Jiang, J. Org. Chem., 2017, 82, 765 CrossRef CAS PubMed;
(i) C. Xie, Z. Zhang, D. Li, J. Gong, X. Han, X. Liu and C. Ma, J. Org. Chem., 2017, 82, 3491 CrossRef CAS PubMed;
(j) Z. He, M. Bae, J. Wu and T. F. Jamison, Angew. Chem., Int. Ed., 2014, 53, 14451 CrossRef CAS PubMed;
(k) A. A. Kalinin and V. A. Mamedov, Chem. Heterocycl. Compd., 2011, 46, 1423 CrossRef CAS;
(l) M. Wang, C. Liu and Y. Gu, Tetrahedron, 2016, 72, 6854 CrossRef CAS;
(m) M. Piltan, Chin. Chem. Lett., 2014, 25, 1507 CrossRef CAS;
(n) Y. Harrak, S. Weber, A. B. Gómez, G. Rosell and M. D. Pujol, ARKIVOC, 2007, 251 CAS.
- For selected reviews:
(a) S. A. Girard, T. Knauber and C.-J. Li, Angew. Chem., Int. Ed., 2014, 53, 74 CrossRef CAS PubMed;
(b) R. A. Baillie and P. Legzdins, Acc. Chem. Res., 2014, 47, 330 CrossRef CAS PubMed;
(c) P. B. Arockiam, C. Bruneau and P. H. Dixneuf, Chem. Rev., 2012, 112, 5879 CrossRef CAS PubMed.
- For selected reviews:
(a) A. E. Wendlandt, A. M. Suess and S. S. Stahl, Angew. Chem., Int. Ed., 2011, 50, 11062 CrossRef CAS PubMed;
(b) S. D. McCann and S. S. Stahl, Acc. Chem. Res., 2015, 48, 1756 CrossRef CAS PubMed;
(c) S. E. Allen, R. R. Walvoord, R. Padilla-Salinas and M. C. Kozlowski, Chem. Rev., 2013, 113, 6234 CrossRef CAS PubMed;
(d) X.-X. Guo, D.-W. Gu, Z. Wu and W. Zhang, Chem. Rev., 2015, 115, 1622 CrossRef CAS PubMed.
-
(a) M. Liu, T. Chen and S.-F. Yin, Catal. Sci. Technol., 2016, 6, 690 RSC;
(b) H. Xie, Y. Liao, S. Chen, Y. Chen and G.-J. Deng, Org. Biomol. Chem., 2015, 13, 6944 RSC;
(c) Y. Huang, T. Chen, Q. Li, Y. Zhou and S.-F. Yin, Org. Biomol. Chem., 2015, 13, 7289 RSC;
(d) G. Zheng, H. Liu and M. Wang, Chin. J. Chem., 2016, 34, 519 CrossRef CAS;
(e) J. Liu, X. Zhang, H. Yi, C. Liu, R. Liu, H. Zhang, K. Zhuo and A. Lei, Angew. Chem., Int. Ed., 2015, 54, 1261 CrossRef CAS PubMed;
(f) Z.-Q. Zhu, P. Bai and Z.-Z. Huang, Org. Lett., 2014, 16, 4881 CrossRef CAS PubMed;
(g) S. Lei, Y. Mai, C. Yan, J. Mao and H. Cao, Org. Lett., 2016, 18, 3582 CrossRef CAS PubMed;
(h) F.-F. Wang, C.-P. Luo, G. Deng and L. Yang, Green Chem., 2014, 16, 2428 RSC;
(i) Z. Tan, H. Zhao, C. Zhou, H. Jiang and M. Zhang, J. Org. Chem., 2016, 81, 9939 CrossRef CAS PubMed;
(j) Q. Li, Y. Huang, T. Chen, Y. Zhou, Q. Xu, S.-F. Yin and L.-B. Han, Org. Lett., 2014, 16, 3672 CrossRef CAS PubMed;
(k) Z. Zhang, Q. Tian, J. Qian, Q. Liu, T. Liu, L. Shi and G. Zhang, J. Org. Chem., 2014, 79, 8182 CrossRef CAS PubMed;
(l) S. Xiang, H. Chen and Q. Liu, Tetrahedron Lett., 2016, 57, 3870 CrossRef CAS.
Footnote |
† Electronic supplementary information (ESI) available: Experimental section, characterization of all compounds, Fig. S1, copies of 1H and 13C NMR spectra for all target compounds. See DOI: 10.1039/c7ra09214h |
|
This journal is © The Royal Society of Chemistry 2017 |
Click here to see how this site uses Cookies. View our privacy policy here.