DOI:
10.1039/C7RA09268G
(Paper)
RSC Adv., 2017,
7, 52762-52771
Epinephrine inhibits vascular hyperpermeability during platelet-activating factor- or ovalbumin-induced anaphylaxis†
Received
22nd August 2017
, Accepted 8th November 2017
First published on 14th November 2017
Abstract
Platelet-activating factor (PAF) has been shown to play a critical role in mediating vascular hyperpermeability during anaphylaxis. Although epinephrine is the first-line treatment for anaphylaxis and can inhibit increased vascular permeability during anaphylaxis, the effect of epinephrine on PAF-induced vascular hyperpermeability is still unclear. In this study, we investigated whether epinephrine can reduce vascular permeability and alleviate anaphylactic symptoms induced by PAF. We tested vascular permeability in vivo by measuring the Evans blue dye leakage and in vitro by transendothelial electrical resistance measurement. Blood pressure was measured with a noninvasive monitoring system. Body temperature was recorded using an electronic thermometer. Confocal microscopy was used to characterize gap areas of human umbilical vein endothelial cells (HUVECs). Phosphorylation of VE-cadherin was assessed using western blot. (1) We observed that epinephrine substantially inhibited PAF-induced vascular permeability in vivo and in vitro. In vivo, administration of epinephrine significantly decreased PAF-induced Evans blue leakage and ear swelling, elevated hematocrit levels and maintained sufficient blood supply to the brain. In vitro, administration of epinephrine alleviated PAF-induced gap numbers and areas in HUVECs. (2) We found that epinephrine can inhibit PAF or ovalbumin (OVA)-induced anaphylactic symptoms. Epinephrine administration effectively inhibited hypothermia and hypotension induced by PAF or OVA. Timely epinephrine administration dramatically decreased the PAF-induced mortality rate. (3) We found that epinephrine inhibited the release of PAF during OVA-induced anaphylaxis. In conclusion, epinephrine administration can reduce PAF-induced vascular hyperpermeability and anaphylactic symptoms, including hypothermia and hypotension. Early addition of epinephrine was critical for the control of PAF-induced anaphylaxis.
Introduction
Anaphylaxis is a serious, potentially fatal, systemic hypersensitivity reaction, which is characterized by cutaneous manifestations and acute respiratory and cardiovascular compromise (laryngeal edema, hypotension and vascular leakage).1 The presence of respiratory or cardiovascular symptoms is a sign of life-threatening anaphylaxis.2 Within the first 10 minutes of anaphylaxis, vascular hyperpermeability can rapidly transfer up to 35% of the intravascular fluid into the extravascular space, which leads to hemodynamic collapse and laryngeal obstruction and exacerbates anaphylactic symptoms.3–5
During anaphylaxis, several pro-inflammatory mediators are released into the bloodstream, among which platelet-activating factor (PAF), histamine, and TNF-alpha have been shown to increase vascular permeability.6–8 PAF, also known as 1-O-alkyl-2-acetyl-sn-glycero-3-phosphocholine, acetyl-glyceryl-ether-phosphorylcholine (AGEPC), or PAF-acether, has been proposed to be the most powerful phospholipid-derived mediator (Fig. 1A).9 It has been shown to be effective at concentrations as low as 10−12 mol L−1, a thousand times lower than that of histamine.9 PAF receptor antagonists that inhibit PAF binding to its receptor can substantially reduce the increased vascular leakage in peanut-sensitized animals.10 In ovalbumin (OVA)-sensitized mice, PAF, rather than histamine, is involved in mouse anaphylactic hypotension.11 PAFR-knockout mice showed less severe hypotension and higher survival rates than wild-type mice.12 Clinical research showed that serum PAF levels were directly correlated with the severity of anaphylaxis.1 Compared with serum tryptase or histamine levels, high PAF levels are better correlated with severe anaphylaxis.13 These animal and human data support the prominent role of PAF in the pathogenesis of anaphylaxis and vascular hyperpermeability.
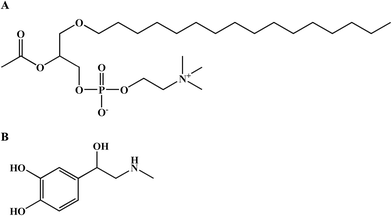 |
| Fig. 1 The molecular structures of PAF (A) and epinephrine (B). | |
Epinephrine is a life-saving agent in treating fatal anaphylaxis (Fig. 1B).2,14,15 Epinephrine rescues patients with anaphylaxis through multiple pharmacological effects, including peripheral vasoconstriction effects via α1 adrenergic receptors, rate and force increases in cardiac contractions via β1 adrenergic receptors, bronchodilation and inhibition of histamine release from mast cells via β2 adrenergic receptors.16 However, the effect of epinephrine in inhibiting PAF-induced vascular hyperpermeability has not been investigated.
Transcellular and paracellular pathways regulate vascular permeability, and paracellular pathways play an important role in the rapid pathophysiological development of anaphylaxis.17 The paracellular permeability of the endothelium is regulated by the interendothelial junctions, including adherens junctions (AJs) and tight junctions (TJs). VE-cadherin is believed to play a prominent role in AJ integrity.18,19 On the one hand, PAF can induce endothelial barrier disruption by breaking the interendothelial junctions, especially the VE-cadherin connection between adjacent vascular endothelial cells.6 On the other hand, an increase in cAMP level in endothelial cells promotes protective barrier function and attenuates endothelial permeability, resulting in decreased vascular leakage.20–22 The beta-adrenergic activation-stimulated cAMP-Epac-Rap1 signal pathway enhanced VE-cadherin-dependent cell adhesion.23 Epinephrine can contribute to maintain the baseline endothelial barrier via increasing cAMP levels by stimulating β adrenergic receptors on endothelial cells.22 However, whether epinephrine can inhibit the PAF-induced endothelial barrier disruption is still unclear.
Thus, the objective of this study was to investigate the protective effect of epinephrine on vascular permeability following PAF- or OVA-induced anaphylaxis in vivo and to explore the effect of epinephrine on endothelial barrier function under PAF challenge in human umbilical vein endothelial cells (HUVECs).
Materials and methods
Reagents and antibodies
Reagents and antibodies PAF (Cat: #P4904), Evans blue dye (Cat: #E2129), and aluminum potassium sulfate adjuvant (Cat: #A6435) were obtained from Sigma-Aldrich (St. Louis, MO, USA); OVA (Cat: #vac-pova-100) was from InvivoGen (San Diego, CA, USA). Epinephrine was purchased from Shanghai Harvest Pharmaceutical Co., Ltd. (Shanghai, China); Darapladib (Cat: #HY-10521) was from Medchem Eexpress (Monmouth junction, NJ, USA). The enzyme-linked immunosorbent assay (ELISA) kit for PAF was purchased from Keboshengchuang Biotechnology (Beijing, China), and VascuLife VEGF Cell Culture Medium was purchased from Lifeline Cell Technology (Frederick, MD, USA). Rabbit anti-phospho-VE-cadherin (Tyr658) antibody (Cat #: 44-1144G) was from Invitrogen (Carlsbad, CA, USA); rabbit anti-phospho-VE-cadherin (Tyr 685) antibody (Cat #: ab 119785) was from Abcam (Cambridge, MA, USA); rabbit anti-phospho-VE-cadherin (Tyr 731) antibody (Cat #: SAB4504676) was from Sigma-Aldrich; rabbit anti-VE-cadherin antibody (Cat: #2500s) was from Cell Signaling Technology (Danvers, MA, USA) and mouse anti-β-actin antibody (Cat: #A5441) was from Sigma-Aldrich (St. Louis, MO, USA); Alexa Fluor 555-labeled goat anti-rabbit IgG secondary antibody (Cat: #A21430) and Hoechst 33342 (Cat: #62249) were obtained from Thermo Fisher (Rockford, IL, USA); and Alexa Fluor 488-labeled rhodamine-phalloidin (Cat: #PHDG1) was from Cytoskeleton (Denver, CO, USA).
Animals
Male BALB/c mice (10–12 weeks old and 25.0 ± 2.0 g) were purchased from Beijing Wei Tong Li Hua Experimental Animal Technology Co., Ltd. (Beijing, China) with the certificate number SCXK (Beijing) 2012-0001. The mice were housed in a specific pathogen-free (SPF) environment at 24 °C under a 12 hour/12 hours light–dark cycle. Mice were given ad libitum access to a normal diet and water. In the PAF-induced anaphylaxis model, male BALB/c mice were randomly divided into the control, PAF or PAF + EPI groups for vascular permeability and hematocrit measurements (n = 18), blood pressure and body temperature measurements (n = 18), survival rate and ear thickness measurements (n = 48) and determination of brain blood supply (n = 18). In the OVA-induced anaphylaxis model, male BALB/c mice (n = 54) were randomly divided into the control, OVA or OVA + EPI groups. The experimental protocol for the model and measurement methods are described below. All procedures were conducted in accordance with the requirements of the Experimentation Ethics Committee on Animal Use of the College of Medicine, Peking University, Beijing, China and the United States National Academy of Sciences Guide for the Care and Use of Laboratory Animals (National Research Council [US] Committee for the Update of the Guide for the Care and Use of Laboratory Animals, 2011). An ethics approval number for all animal studies was granted by the Experimentation Ethics Committee of the Peking University Health Science Center (LA2016110).
Anaphylaxis
Anaphylaxis was induced via intravenous injection of PAF through the tail vein. Three different PAF doses were used for different type of studies. In the measurement of anaphylactic symptoms, 10 μg kg body wt−1 PAF was used. In the measurement of brain blood supply by Laser Doppler Line Scanner, 40 μg kg body wt−1 PAF was selected. In the survival experiment, 80 μg kg body wt−1 PAF was administered. The epinephrine intervention group was injected with epinephrine (100 μg kg body wt−1 in 200 μL of physiologic saline, i.v.) immediately after PAF injection. The control group was injected with 200 μL of physiologic saline. The dose of PAF for the induction of anaphylaxis was selected according to Macro's method.24 The dose of epinephrine was selected according to the veterinary formulary on the website of research animal resources of the University of Minnesota.25 Body temperature and blood pressure were monitored every 10 minutes until 60 minutes after the challenge.
Alternatively, anaphylaxis was induced via OVA with two-week duration.26 Mice were sensitized with 0.1 mg of OVA and 2 mg of aluminum potassium sulfate adjuvant dissolved in 200 μL of physiologic saline via subcutaneous injection. The antigen was injected twice at a one-week interval. Nonsensitized mice were injected with aluminum potassium sulfate and OVA-free saline (i.v.). At 2 weeks after the first injection, mice were challenged by i.v. OVA through the tail vein. The OVA model group and nonsensitized mice were both challenged with OVA (0.1 mg in 200 μL of physiologic saline), and the epinephrine intervention group was injected with epinephrine (100 μg kg body wt−1 in 200 μL of physiologic saline, i.v.) immediately after 0.1 mg OVA injection. Body temperature, ear thickness, and hematocrit of mice were monitored at 15, 30, and 60 minute time points after OVA challenge, and plasma PAF levels were measured 15 minutes after OVA challenge. The ear thickness was measured using digital caliper. The detailed methods were described as below. Mice were euthanized with CO2 1 hour after OVA challenge.
Vascular permeability and hematocrit measurements
In the PAF-induced anaphylaxis model, mice were injected with PAF in 0.5% Evans blue dye solution diluted in 200 μL physiologic saline (i.v.). Ten minutes later, the mouse ears were photographed by 5 megapixel camera with a 3.85 mm f/2.8 lens, and mice were anesthetized with ketamine (100 mg kg body wt−1) and xylazine (2.5 mg kg body wt−1). Blood samples were obtained from the right ventricle to determine hematocrit. The ears were removed, and the ear weight was determined. After ears were dried at 60 °C overnight, ear tissues were sonicated in 0.5 mL formamide for 30 seconds. Four days later, the Evans blue in formamide extract was measured as OD620 nm.27
Blood pressure and body temperature measurements
Nonanesthetized mice were used to simulate realistic conditions. Mice were trained in three days, 15 minutes each day, before beginning blood pressure measurement, so that they were accustomed to being restrained in the holder. Systolic and diastolic blood pressure was measured using a volume–pressure recording noninvasive blood pressure monitoring system (CODA, Kent Scientific, Torrington, CT, USA). Blood pressure was measured at baseline and every 10 minutes for 1 hour after challenge. Rectal body temperature was recorded using an electronic thermometer (HI 98509, Hanna instrument, Woonsocket, RI, USA) at baseline and every 10 minutes for 1 hour after challenge. Mice were euthanized with CO2 after blood pressure and body temperature measurements.
Survival rate and ear thickness measurements
In survival experiments, mice were intravenously injected with PAF (80 μg kg body wt−1) alone or PAF (80 μg kg body wt−1) with epinephrine (100 μg kg body wt−1) simultaneously or epinephrine 1, 5, and 10 minutes after PAF injection. The PAF dose for the survival experiment was selected according to a previously published paper.24 The survival rate was monitored every 10 minutes until 120 minutes after challenge, and then mice were euthanized with CO2. The thickness of both ears was measured using digital caliper (0–200 mm, blue light machinery and electricity instrument, Beijing, China) on the day before intervention. Thirty minutes later, after the injection of PAF or when the mice were dead within 30 minutes after injection, the thickness of both ears was measured.
Determination of brain blood supply by laser doppler line scanner (LDLS)
In the PAF-induced anaphylaxis model, mice were administered intravenously with PAF (40 μg kg body wt−1) with/without epinephrine (100 μg kg body wt−1) through the tail vein. Then, mice were anesthetized by an intraperitoneal injection of ketamine (100 mg kg body wt−1) with xylazine (2.5 mg kg body wt−1). The scalp was incised after mice received anesthesia. The brain blood supply was measured by LDLS (Moor Instruments Ltd, UK) 10, 20, 30, and 60 minutes after the challenge. The mode of image scan measurement was used. The blood flow image is in the range of 0 to 600 flux units.
Determination of PAF levels
Considering the release of PAF occurs within minutes in the early stages of anaphylaxis,28 plasma PAF level 15 minutes after OVA challenge was measured by an ELISA kit according to the manufacturer's protocol. Given the unstable characteristics of PAF in the blood sample due to degradation by PAF acetylhydrolase, the PAF-AH inhibitor Darapladib (20 μL of 100 nM Darapladib in 50 mg mL−1 EDTA) was added to 300 μL blood samples and gently mixed. Samples were centrifuged for 15 minutes at 1000 × g at 4 °C within 30 minutes of collection. The plasma was removed, and the assay was immediately performed using a microplate reader (Thermo Fisher Scientific Waltham, MA, USA) at a wavelength of 450 nm.
Cell lines and cell culture
HUVECs were obtained from Lifeline Cell Technology (Frederick, MD, USA). The cells were incubated in VascuLife VEGF Cell Culture Medium plus 2% (v/v) heat-inactivated fetal calf serum at 37 °C with 5% CO2 and 95% air in a humidified atmosphere and used between passages 2 to 8. Passages 4 and 6 of HUVECs were used for trans-endothelial electrical resistance junction permeability assay and immunofluorescence. Passages 7 and 8 of HUVECs were used for Western blot.
Trans-endothelial electrical resistance (TER) junction permeability assay
An electrical cell-substrate impedance system (ECIS 1600R, Applied BioPhysics Inc., NY, USA) was used to measure the TER of endothelial junction integrity. First, 5 × 104 HUVECs were seeded in gelatin-coated 8-well arrays (Cat #: 8 W10E+, Applied BioPhysics) and reached confluence in three days. After the HUVECs were serum starved for two hours, the resistance between the baseline and 1.5 hours post PAF (2 μM) with/without epinephrine (10 μM) was measured by ECIS 1600R. The dose of PAF and epinephrine was selected according to published papers.28,29
Immunofluorescence
Monolayer-cultured HUVECs (5 × 104 cells) were seeded on collagen-coated glass-base dishes and cultured for approximately 48 hours. A confluent monolayer of HUVECs was challenged by 2 μM PAF with/without 10 μM epinephrine for 10 minutes. Immunofluorescence was performed according to Mikelis' method.7 After challenge, HUVECs were fixed with 2% formaldehyde in PBS for 30 minutes at 4 °C, permeabilized with 0.05% Triton X-100 for 25 minutes at 4 °C and blocked with 10% sheep serum for 1 hour at 37 °C. The cells were immunostained with anti-VE-cadherin antibodies at 4 °C overnight. Then, HUVECs were incubated with species-matched Alexa Fluor 555-labeled secondary antibody for 2 hours at 37 °C. At the same time, Alexa Fluor 488-labeled rhodamine-phalloidin was added to stain the F-actin. Then, cells were stained with Hoechst 33342 (1
:
1000) for 5 minutes at 37 °C. Fluorescence images were recorded with a confocal microscope (A1, Nikon, Japan) with ×60 oil immersion objective lenses and ×2.5 zoom in on some images.
For High-Content Imaging Assays, HUVECs (2 × 104 cells) were seeded in a 96-well plate and incubated for 2 days to achieve 90–100% confluence. HUVECs were challenged with 2 μM PAF with/without 10 μM epinephrine for 10 minutes. After stimulation, HUVECs were fixed and permeabilized as described in Mikelis' method.7 Then, HUVECs were stained with Alexa Fluor 488-labeled rhodamine-phalloidin for 2 hours at 37 °C and stained with Hoechst 33342 (1
:
1000) for 5 minutes at 37 °C. During acquisition, 25 fields per well were randomly imaged using ×60 objective for the following channels: UV/blue for Hoechst nuclear stain, FITC/FITC for Alexa Fluor 488-labeled rhodamine-phalloidin. Data analysis was then performed via imaging analysis of the Operetta high-content imaging system (Operetta, PerkinElmer, Santa Clara, CA, USA).
Western blot
SDS-PAGE and western blotting were performed according to established protocols.30,31 HUVECs were treated with 2 μM PAF for 1, 5, and 15 minutes. Cells were lysed directly in culture dishes on ice by adding RIPA buffer containing 1% protease and phosphatase inhibitor cocktail (P8340, P5726, P0044, Sigma-Aldrich, St. Louis, MO, USA). The protein concentration was determined using a BCA protein assay (Cat: #23225, Thermo Fisher, Rockford, IL, USA). Thirty micrograms from each sample of lysed HUVECs was loaded into each well of 8% SDS-PAGE gels. Samples were separated by SDS-PAGE gels, transferred to nitrocellulose membranes, and immunoblotted with the following primary antibodies: rabbit anti-phospho-VE-cadherin (Tyr658) antibody (1
:
500), rabbit anti-phospho-VE-cadherin (Tyr 685) antibody (1
:
500), rabbit anti-phospho-VE-cadherin (Tyr 731) antibody (1
:
500), rabbit anti-VE-cadherin antibody (1
:
3000) and mouse anti-β-actin antibody (1
:
5000). After the incubation of primary antibody, membranes were washed with PBST and incubated with anti-rabbit (1
:
2500) or anti-mouse secondary antibody (1
:
2500). Blots were scanned as grayscale images and quantified using Quantity One software (version 4.6.2, Hercules, CA, USA). The optical density of each band was quantified.
Statistics
All values are expressed as the mean ± SEM. Statistical comparisons of data between two groups were analyzed using the 2-tailed Student's t test, and comparisons between three or more groups were analyzed using one-way ANOVA, followed by a Newman–Keuls post hoc test. Two-way ANOVA was used to analyze differences between different challenges in the time course. A value of p < 0.05 was used to indicate a significant difference.
Results
Epinephrine protects mice from vascular hyperpermeability in PAF-induced anaphylaxis
After injection of PAF, there was obvious infiltration of blue dye into the skin tissue of the ears, forelimbs and eyelids (Fig. 2A and B). There was a more than threefold increase in Evans blue in the ear tissue of the PAF group compared with that in the control group (p < 0.001). Evans blue infiltration in the ear tissue of the PAF + EPI group was significantly less than that in the PAF group (p < 0.05). Ear thickness of the mice in the PAF group was significantly larger than the thickness in the control group (p < 0.001, Fig. 2C). Administration of epinephrine inhibited the increase in ear thickness in a time-dependent manner. Simultaneously injecting epinephrine with PAF or injecting epinephrine 1 minute after PAF injection significantly suppressed ear thickness (p < 0.001). However, the effectiveness of epinephrine was decreased when epinephrine was injected 5 or 10 minutes after PAF challenge. To confirm that PAF can cause rapid transfer of intravascular fluid into the extravascular space, we measured hematocrit levels 10 minutes after PAF challenge. PAF-challenged mice showed higher hematocrit levels (p < 0.001), which could be inhibited by the administration of epinephrine (p < 0.001, Fig. 2D).
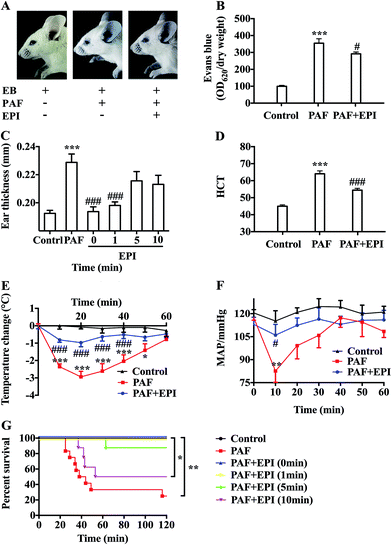 |
| Fig. 2 Epinephrine alleviates PAF-induced vascular hyperpermeability and anaphylactic symptoms. Ten minutes after the intravenous injection of 200 μL 0.5% Evans blue with/without PAF (10 μg kg body wt−1) and epinephrine (100 μg kg body wt−1) through the tail vein, the mice were assayed. (A) The BALB/c mice were photographed. (B) The ear tissues were harvested for the calculation of the ratio of OD620 to dry ear weight to determine Evans blue leakage. (C) Ear thickness at baseline was measured the day before challenge and 30 minutes after challenge. (D) The blood samples were obtained from the right ventricle for the HCT levels. (E and F) Within 1 hour after intravenous injection of 200 μL PAF (10 μg kg body wt−1) with/without epinephrine (100 μg kg body wt−1) through the tail vein, body temperature and MAP were measured every 10 minutes. (G) The survival rate was monitored every 10 minutes until 120 minutes after challenge, and then mice were euthanized with CO2. In the survival experiment, mice were challenged with PAF (80 μg kg body wt−1) alone or PAF (80 μg kg body wt−1) with epinephrine (100 μg kg body wt−1) simultaneously or epinephrine 1, 5, and 10 minutes after PAF injection. EPI: epinephrine; HCT: hematocrit; MAP: mean arterial blood pressure. The data are presented as the mean ± SEM (n = 6–12); *p < 0.05; **p < 0.01; ***p < 0.001, compared with the control group; #p < 0.05; ###p < 0.001, compared with the PAF group; if not marked, differences were not statistically significant. | |
Epinephrine attenuates PAF-induced anaphylactic symptoms
PAF can induce many anaphylactic symptoms, including dyspnea (Appendix Video 1†), hypothermia, hypotension and even death. From 10 to 50 minutes after PAF challenge, the rectal temperatures of the mice in the PAF group were significantly lower than those in the control group (p < 0.001, p < 0.001, p < 0.001, p < 0.001, p < 0.05, respectively, Fig. 2E). Ten minutes after PAF challenge, the mean arterial blood pressures of the mice in the PAF group were dramatically lower than those in the control group (p < 0.01, Fig. 2F). The administration of epinephrine effectively increased the rectal temperature from 10 to 40 minutes after PAF challenge (p < 0.001, p < 0.001, p < 0.001, p < 0.001, Fig. 2E) and increased mean arterial blood pressure at 10 minute intervals (p < 0.05, Fig. 2F). Epinephrine time-dependently reduced the mortality rate caused by the injection of a lethal dose of PAF (80 μg kg body wt−1) (Fig. 2G). Both simultaneous injection of epinephrine and injection of epinephrine 1 minute after PAF injection effectively increased the survival rate from 25% (3/12) to 100% (8/8). However, the delayed injection of epinephrine was less effective. The injection of epinephrine 5 or 10 minutes after PAF injection only increased the survival rate to 87.5% (7/8) or 50% (4/8).
In preliminary experiments, we observed that the administration of lethal-dose PAF (i.v.) induced opisthotonus or generalized tonic symptoms lasting for 10 to 20 seconds (Appendix Video 2†). We hypothesized that these manifestations were related to the low blood supply in the brain. Therefore, we tested the bloodstream volume of the brain by LDLS, which presents the blood perfusion volume of the brain as a deep blue to a deep red color (Fig. 3A and B). The blood supply of the brain was significantly decreased at 10, 20, 30 and 60 minutes after PAF challenge (p < 0.001, p < 0.001, p < 0.001, p < 0.01, respectively, Fig. 3C). Simultaneous injection of epinephrine with PAF effectively inhibited the PAF-induced decrease in the brain blood supply at the 10, 20, 30 and 60 minute time points (p < 0.001, p < 0.01, p < 0.05, p < 0.05, respectively).
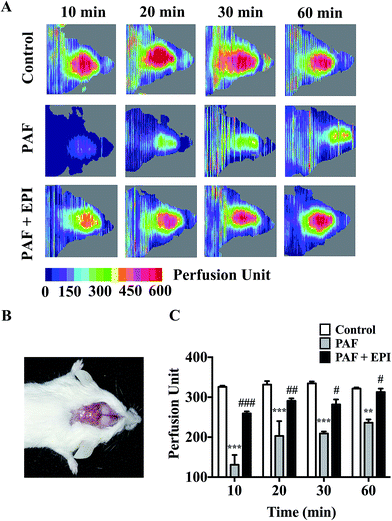 |
| Fig. 3 Epinephrine inhibits PAF-induced reduction of brain blood supply. (A) The mice were intravenously injected with 200 μL physiologic saline or PAF (40 μg kg body wt−1) with/without epinephrine (100 μg kg body wt−1). Then, mice were anesthetized, and the brain blood supply was measured by LDLS at 10, 20, 30, and 60 minutes after challenge. (B) Image of a scalp incised after mice were anesthetized. (C) The bloodstream perfusion unit at 10, 20, 30, and 60 minutes after intravenous injection. EPI: epinephrine. The data are presented as the mean ± SEM (n = 3–6); **p < 0.01; ***p < 0.001, compared with the control group; #p < 0.05; ##p < 0.01; ###p < 0.001, compared with the PAF group. If not marked, differences were not statistically significant. | |
Epinephrine protects mice from vascular hyperpermeability and hypothermia in OVA-induced anaphylaxis
OVA-induced active systemic anaphylaxis is a classical model for the investigation of systemic anaphylaxis. The protocol of OVA-induced active anaphylaxis is shown (Fig. 4A). Ear thickness was significantly augmented at 15, 30 and 60 minutes after OVA stimulation (p < 0.001, p < 0.001, p < 0.001, respectively, Fig. 4B). The injection of epinephrine effectively suppressed the ear swelling induced by OVA (p < 0.01, p < 0.001, p < 0.05, respectively, Fig. 4B). Hematocrit levels were significantly increased at the 15 minute time point and gradually decreased from 30 to 60 minutes after OVA stimulation (p < 0.001, p < 0.01, p < 0.01, respectively, Fig. 4C). Although it was not significant, the average HCT levels in the OVA + EPI group were lower than those in the OVA group (Fig. 4C). The PAF levels in the serum of mice with OVA-induced anaphylaxis were significantly higher than those in the control group (p < 0.01, Fig. 4D). The administration of epinephrine with OVA effectively reduced the elevated PAF level at the 15 minute time point after OVA stimulation (p < 0.01, Fig. 4D). Given that hypothermia serves as an important indicator of anaphylaxis in the OVA-induced active anaphylaxis model, we examined temperature change after OVA stimulation. The data showed that OVA significantly decreased body temperature 15, 30 and 60 minutes after OVA challenge (p < 0.001, p < 0.001, p < 0.01, Fig. 4E). The results indicated that epinephrine could significantly attenuate OVA-induced hypothermia at 15 and 30 minute time points (p < 0.05, p < 0.05, respectively, Fig. 4E).
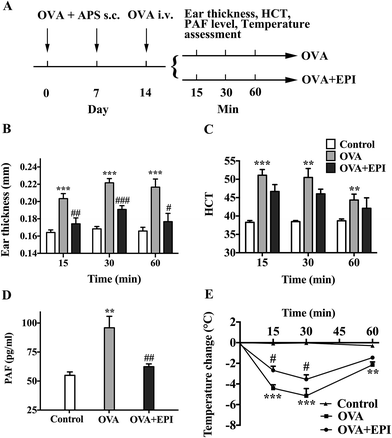 |
| Fig. 4 Epinephrine alleviates OVA-induced active systemic anaphylaxis. (A) Scheme of two-week OVA sensitization. BALB/c mice were subcutaneously injected with 0.1 mg OVA and 2 mg aluminum potassium sulfate on day 0 and day 7. On day 14, mice were intravenously injected with 0.1 mg OVA in 200 μL physiologic saline with/without epinephrine (80 μg kg body wt−1). Mice in the control group were only intravenously injected with 0.1 mg OVA in 200 μL physiologic saline. (B and C) After challenge on day 14, ear thickness and HCT levels were measured at 15, 30, and 60 minute time points. (D) Fifteen minutes after OVA challenge, blood samples were obtained to measure PAF levels. (E) Rectal temperature was measured at 15, 30, and 60 minute time points. HCT: hematocrit. The data are presented as the mean ± SE (n = 6); **p < 0.01; ***p < 0.001, compared with the control group; #p < 0.05; ##p < 0.01; ###p < 0.001, compared with the PAF group. If not marked, differences were not statistically significant. | |
Epinephrine protects HUVECs from PAF-induced severe endothelial barrier disruption
In confluent HUVEC monolayers, TER decreased to 73% of baseline levels (from 2138 ± 68 ohms to 1568 ± 125 ohms, n = 3) after 15 minutes of PAF incubation. TER increased to 118% of baseline levels (from 2177 ± 43 ohms to 2575 ± 41 ohms, n = 3) after 10 minutes of epinephrine incubation. The effect of PAF on the endothelial barrier was inhibited by co-incubation of epinephrine with PAF, resulting in values similar to baseline levels. As epinephrine acts faster than PAF, TER increased slightly and then decreased to baseline levels after co-incubation of epinephrine and PAF (Fig. 5A). As shown in Fig. 5B, PAF induced disruption and disturbance of VE-cadherin, which was inhibited by co-incubation of epinephrine with PAF (Fig. 5B, line 1, PAF + EPI). When HUVECs were stimulated with PAF, we observed the formation of radial actin bundles (Fig. 5B line 2, PAF). When HUVECs were stimulated with epinephrine and PAF, we observed the existence of more cytoplasmic fibers scattered in the center of the HUVECs (Fig. 5B line 2, PAF + EPI).
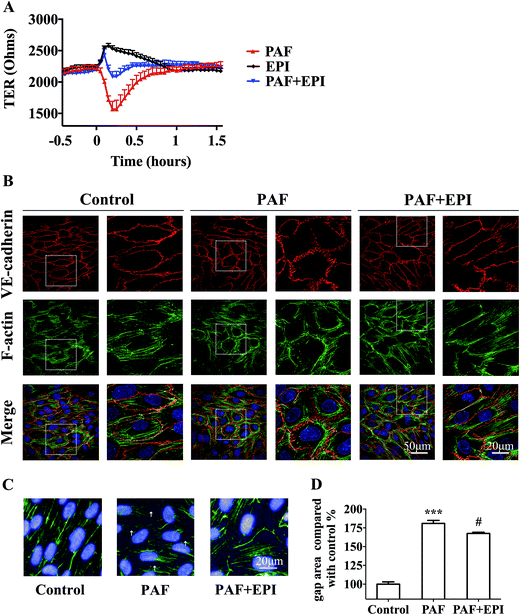 |
| Fig. 5 Epinephrine protects HUVECs from PAF-induced endothelial barrier disruption. (A) TER junction permeability assay. After the HUVECs were serum starved, the TER was measured from 0.5 hour before the stimulation to 1.5 hours after stimulation with 2 μM PAF with/without 10 μM epinephrine by ECIS 1600R. (B) Immunofluorescence staining of VE-cadherin and F-actin was photographed with a confocal microscope. HUVECs were treated with 2 μM PAF with/without 10 μM epinephrine for 10 minutes. The data are presented as the mean ± SEM (n = 3). (C and D) Staining images of F-actin and nuclei were photographed by Operetta High-Content Assay (HCA). Gap areas of confluent HUVECs monolayer were calculated by HCA. The gaps are marked with white arrowheads. The data are presented as the mean ± SEM (n = 5); ***p < 0.001, compared with the control group; #p < 0.05, compared with the PAF group. If not marked, differences were not statistically significant. | |
For the quantification of the change in gap areas, high-content imaging screening was used. Incubation of PAF for 10 minutes disrupted the interendothelial junctions and significantly increased the gap areas of confluent HUVECs (p < 0.001, Fig. 5C and D). Co-incubation of epinephrine significantly inhibited the PAF-induced increase in gap areas (p < 0.05, Fig. 5C and D).
Several studies have reported that PAF can induce tyrosine phosphorylation of VE-cadherin and can promote its internalization, resulting in an increase in vascular permeability.18 We first reported that PAF induced the phosphorylation of Y658, Y685 and Y731 of VE-cadherin in HUVECs by using phosphorylation-specific antibodies (Fig. 6A). In HUVECs, incubation with 2 μM PAF induced phosphorylation of VE-cadherin at the Y658 residue after 15 minutes (p < 0.01, Fig. 6B), at the Y685 residue after 1 minute (p < 0.05, Fig. 6C) and at the Y731 residue at 1 and 5 minutes (p < 0.05, p < 0.01, respectively, Fig. 6D). Whether epinephrine protects VE-cadherin from phosphorylation by PAF remains to be answered.
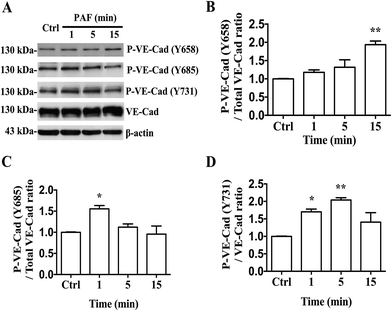 |
| Fig. 6 PAF induces VE-cadherin phosphorylation. (A) Western blot analysis. HUVECs were treated with 2 μM PAF for the indicated times. PAF can induce the phosphorylation of VE-cadherin at the Y658, Y685 and Y731 residues. (B) The ratio of the protein levels of VE-cadherin phosphorylated at Y658 to total VE-cadherin protein levels. (C) The ratio of the protein levels of phosphorylated VE-cadherin at Y685 to total VE-cadherin protein expression levels. (D) The ratio of the protein levels of VE-cadherin phosphorylated at Y731 to total VE-cadherin protein expression levels. Data are expressed as the mean ± SEM (n = 3). *p < 0.05, **p < 0.01 compared with the control. If not marked, differences were not statistically significant. | |
Discussion
PAF is one of the most potent inflammatory mediators that is responsible for anaphylactic symptoms.9 Traditionally, researchers have viewed OVA or bovine serum albumin (BSA) sensitization as a classical model of active systemic anaphylaxis.32 In our study, we demonstrated that PAF (i.v.) induced similar anaphylactic symptoms to those exhibited in OVA- or BSA-induced models, including tissue edema, rapid and profound drops in body temperature and blood pressure, dyspnea and even death. Therefore, PAF-induced anaphylaxis may be a standard model for anaphylaxis. In addition, we demonstrated that increased vascular permeability plays an important role in the early stages of PAF-induced anaphylaxis. Using Evans blue, we observed that PAF can cause the rapid transfer of intravascular fluid into the extravascular space in 10 minutes. By using a Doppler scanner, we observed that increased vascular permeability is responsible for hypoperfusion in the brain. The mice that died from PAF-induced anaphylaxis suffered from more severe tissue edema than other mice. Therefore, the inhibition of increased vascular permeability should be viewed as an important treatment strategy for anaphylaxis.
Epinephrine should be administered early to anaphylaxis patients because the delayed administration of epinephrine is associated with mortality.33,34 However, as far as we know, the current recommendation for early administration of epinephrine is based on retrospective studies33,34 or in vitro studies.28 We demonstrated with in vivo data that the early use of epinephrine was vital for treating PAF-induced anaphylaxis. As time increased, epinephrine was progressively less effective in treating lethal-dose PAF-challenged mice and inhibiting PAF-induced tissue swelling. It was shown that epinephrine can inhibit the PAF release in the early stage of OVA-induced anaphylaxis, indicating the important inhibitory effect of epinephrine on the release of mediators from mast cells. It has been reported that aggregation of IgE-occupied FcεRI can activate mast cells, resulting in the liberation of intracellular calcium and the release of proinflammatory mediators from secretary granules, including PAF, histamine and leukotrienes.35–37 Recently, it has been reported that stimulation of β2 adrenergic receptors on mast cells can increase cytosolic cAMP level and activate cAMP-PKA signaling pathway, resulting in suppressing IgE/antigen-dependent signaling and calcium influx in mast cell degranulation.38,39 However, the detailed molecular mechanism responsible for the regulation of mast cell activation by β2 adrenoceptor agonists remains to be studied further.
The currently recognized effects of epinephrine in treating anaphylaxis include vasoconstriction, cardiac inotropic effects and bronchodilation.40 However, whether epinephrine contributes to the protection of vascular permeability during anaphylaxis is not well understood. Epinephrine was shown to stimulate the endothelium via β adrenergic receptors, which contribute to the maintenance of endothelial barrier properties under baseline conditions.22 We demonstrated that epinephrine can alleviate endothelial barrier disruption under PAF-induced pathological conditions. PAF reduced TER of confluent HUVECs and induced the disintegration of VE-cadherin staining. By contrast, epinephrine increased TER and enhanced linearized VE-cadherin distribution. Epinephrine presumably protects the endothelial barrier function by increasing intracellular cAMP levels.22 In ischemia-reperfusion hyperpermeability, endothelial cells can be deactivated by the stimulation of cAMP production through cAMP-Epac-Rap1 signaling.20 cAMP-Epac-Rap1 has been shown to promote the formation of VE-cadherin-based junctions.41 Several studies have demonstrated that the phosphorylation of VE-cadherin tyrosine residues promotes its internalization, resulting in vascular hyperpermeability.41,42 Vascular permeability-increasing factors such as VEGF, TNF or histamine, induce the phosphorylation of VE-cadherin at Y685, resulting in an increase in vascular permeability.42 Wessel et al. generated knock-in mice expressing VE-cadherin mutants with the replacement of tyrosine by phenylalanine at position 685 (Y685F) and demonstrated that Y685 regulates vascular permeability.43 Several studies have reported that PAF increases the phosphorylation of VE-cadherin tyrosine residues.18,44 Here, we first demonstrated that PAF increased the phosphorylation of the Y658, Y685 and Y731 residues. Whether epinephrine protects VE-cadherin from being phosphorylated by vascular permeability-increasing factors such as PAF or histamine remains to be answered. Elucidation of the mechanisms that influence the functions of VE-cadherin, which would allow the discovery of new specific targets, should be carried out.
The doses of PAF and epinephrine were selected according to published papers and our pilot study.24,25,28 The intravenous injection of the 10 μg kg body wt−1 PAF can induce mild to moderate anaphylactic symptoms without resulting in the mortality of BALB/c mice, which was suitable for the measurement of tissue edema, rapid and profound drops in body temperature and blood pressure. However, this dose was not potent enough for the survival experiment since all the injected mice can survive. According to Macro's study, the LD80 of PAF was 80 μg kg body wt−1 (i.v.) in Swiss mice.24 We performed a pilot study with 4 BALB/c mice and found that this dose was lethal in 3 of 4 mice. Therefore, we selected a dose of 80 μg kg body wt−1 (i.v.) PAF in the survival experiment. For measuring the brain blood supply by LDLS, we adjusted PAF dose from 80 to 40 μg kg body wt−1 (i.v.), in order to keep the sufficient number of alive mice to complete the experiment. The dose of epinephrine was selected according to the veterinary formulary on the website of research animal resources of the University of Minnesota,25 which noted that the dose of epinephrine is 0.2 mg kg body wt−1 (i.v.) for treating anaphylaxis and 0.1 mg kg body wt−1 (i.v.) for cardiac resuscitation of mice. In our preliminary experiment, we found that mice intravenously injected with 0.2 mg kg body wt−1 epinephrine presented anxiety, restlessness and respiratory difficulty. Therefore, we decreased the dose of epinephrine and selected 0.1 mg kg body wt−1 (i.v.) as the dose for the experiments. In the in vitro study, the concentration of PAF was extrapolated from the PAF dose of the survival study, and the concentration of epinephrine was chosen according to Peter's study.28
Conclusions
In conclusion, we first reported that (1) epinephrine can inhibit PAF-induced vascular hyperpermeability in vivo and in vitro; (2) epinephrine can inhibit PAF or OVA-induced anaphylactic indicators; and (3) epinephrine can inhibit the release of PAF during OVA-induced anaphylaxis. Further illustration of the molecular mechanisms of endothelial barrier protection of epinephrine will assist in the development of drugs with more specific targets and fewer unexpected adverse events in treating anaphylaxis.
Author contributions
XM, XP and SZ conceived and designed the study. XM, YX, HL, and JX performed the in vivo studies. WL, YS, and XZ performed the in vitro studies. XM and YX interpreted the data and drafted the manuscript. XP and SZ revised the manuscript. All other authors critically reviewed the manuscript.
Conflict of interest
The authors have no conflict of interest to declare.
Nonstandard abbreviations and acronyms
AJs | Adherens junctions |
BSA | Bovine serum albumin |
HUVECs | Human umbilical vein endothelial cells |
i.v. | Intravenous injection |
LDLS | Laser doppler line scanner |
OVA | Ovalbumin |
PAF | Platelet-activating factor |
PAFR | Platelet-activating factor receptor |
PKA | Protein kinase A |
Rap 1 | Ras-related protein 1 |
TJs | Tight junctions |
VEGF | Vascular endothelial growth factor |
Acknowledgements
This work was supported by National Special Equipment Development Program (No. 2013YQ030651-06), Science and Technology Major Projects: Significant New-Drugs Creation (No. 2017ZX09101003-009-006).
References
- P. Vadas, M. Gold, B. Perelman, G. M. Liss, G. Lack, T. Blyth, F. E. Simons, K. J. Simons, D. Cass and J. Yeung, N. Engl. J. Med., 2008, 358, 28–35 CrossRef CAS PubMed.
- A. Muraro, G. Roberts, M. Worm, M. B. Bilò, K. Brockow, R. M. Fernández, A. F. Santos, Z. Q. Zolkipli, A. Bellou, K. Beyer, C. Bindslev-Jensen, V. Cardona, A. T. Clark, P. Demoly, A. E. Dubois, A. DunnGalvin, P. Eigenmann, S. Halken, L. Harada, G. Lack, M. Jutel, B. Niggemann, F. Ruëff, F. Timmermans, B. J. Vlieg-Boerstra, T. Werfel, S. Dhami, S. Panesar, C. A. Akdis and A. Sheikh, Allergy, 2014, 69, 1026–1045 CrossRef CAS PubMed.
- N. Faye, L. Fournier, D. Balvay, R. Thiam, G. Orliaguet, O. Clément and P. Dewachter, Anesthesiology, 2012, 117, 1072–1079 CrossRef CAS PubMed.
- C. C. Mariana, Anaphylaxis and hypersensitivity reactions, Humana Press, New York, 2011, pp. 33–46 Search PubMed.
- I. Toyota, M. Tanida, T. Shibamoto, M. Wang, Y. Kurata and H. Tonami, Allergy, Asthma Immunol. Res., 2013, 5, 389–396 CrossRef PubMed.
- I. I. Knezevic, S. A. Predescu, R. F. Neamu, M. S. Gorovoy, N. M. Knezevic, C. Easington, A. B. Malik and D. N. Predescu, J. Biol. Chem., 2009, 284, 5381–5394 CrossRef CAS PubMed.
- C. M. Mikelis, M. Simaan, K. Ando, S. Fukuhara, A. Sakurai, P. Amornphimoltham, A. Masedunskas, R. Weigert, T. Chavakis, R. H. Adams, S. Offermanns, N. Mochizuki, Y. Zheng and J. S. Gutkind, Nat. Commun., 2015, 6, 6725 CrossRef CAS PubMed.
- R. P. Naikawadi, N. Cheng, S. M. Vogel, F. Qian, D. Wu, A. B. Malik and R. D. Ye, Circ. Res., 2012, 111, 1517–1527 CrossRef CAS PubMed.
- P. Gill, N. L. Jindal, A. Jagdis and P. Vadas, J. Allergy Clin. Immunol., 2015, 135, 1424–1432 CrossRef CAS PubMed.
- K. Arias, M. Baig, M. Colangelo, D. Chu, T. Walker, S. Goncharova, A. Coyle, P. Vadas, S. Waserman and M. Jordana, J. Allergy Clin. Immunol., 2009, 124, 307–314 CrossRef CAS PubMed.
- T. Shibamoto, W. Liu, S. Cui, W. Zhang, H. Takano and Y. Kurata, Pharmacology, 2008, 82, 114–120 CrossRef CAS PubMed.
- S. Ishii, T. Kuwaki, T. Nagase, K. Maki, F. Tashiro, S. Sunaga, W. H. Cao, K. Kume, Y. Fukuchi, K. Ikuta, J. Miyazaki, M. Kumada and T. Shimizu, J. Exp. Med., 1998, 187, 1779–1788 CrossRef CAS PubMed.
- P. Vadas, B. Perelman and G. Liss, J. Allergy Clin. Immunol., 2013, 131, 144–149 CrossRef CAS PubMed.
- F. E. Simons, L. R. Ardusso, M. B. Bilo, V. Cardona, M. Ebisawa, Y. M. El-Gamal, P. Lieberman, R. F. Lockey, A. Muraro, G. Roberts, M. Sanchez-Borges, A. Sheikh, L. P. Shek, D. V. Wallace and M. Worm, World Allergy Organ. J., 2014, 7, 9 CrossRef PubMed.
- F. E. Simons, L. R. Ardusso, V. Dimov, M. Ebisawa, Y. M. El-Gamal, R. F. Lockey, M. Sanchez-Borges, G. E. Senna, A. Sheikh, B. Y. Thong and M. Worm, Int. Arch. Allergy Immunol., 2013, 162, 193–204 CrossRef CAS PubMed.
- F. E. Simons, J. Allergy Clin. Immunol., 2004, 113, 837–844 CAS.
- Y. A. Komarova and A. B. Malik, Annu. Rev. Physiol., 2010, 72, 463–493 CrossRef CAS PubMed.
- A. Guequén, R. Carrasco, P. Zamorano, L. Rebolledo, P. Burboa, J. Sarmiento, M. P. Boric, A. Korayem, W. N. Durán and F. A. Sánchez, Am. J. Physiol.: Heart Circ. Physiol., 2016, 310(8), H1039–H1044 Search PubMed.
- Y. A. Komarova, K. Kruse, D. Mehta and A. B. Malik, Circ. Res., 2017, 120(1), 179–206 CrossRef CAS PubMed.
- A. H. Korayem, P. E. Mujica, H. Aramoto, R. G. Durán, P. R. Nepali, D. D. Kim, A. L. Harris, F. A. Sánchez and W. N. Durán, Am. J. Physiol.: Heart Circ. Physiol., 2017, 313(1), H179–H189 CrossRef PubMed.
- N. Schlegel and J. Waschke, Cell Tissue Res., 2014, 355, 587–596 CrossRef CAS PubMed.
- V. Spindler and J. Waschke, Microcirculation, 2011, 18, 118–127 CrossRef CAS PubMed.
- K. Noda, J. Zhang, S. Fukuhara, S. Kunimoto, M. Yoshimura and N. Mochizuki, Mol. Biol. Cell, 2010, 21, 584–596 CrossRef CAS PubMed.
- C. Macro and S. Alessandro, Br. J. Pharmacol., 1987, 90, 203–209 CrossRef.
- Veterinary Formulary for epinephrine, http://www.ahc.umn.edu/rar/umnuser/formulary.html#Epinephrine, accessed March 3 2016.
- H. Cui, Y. Okamoto, K. Yoshioka, W. Du, N. Takuwa, W. Zhang, M. Asano, T. Shibamoto and Y. Takuwa, J. Allergy Clin. Immunol., 2013, 132, 1205–1214 CrossRef CAS PubMed.
- T. L. Phung, K. Ziv, D. Dabydeen, G. Eyiah-Mensah, M. Riveros, C. Perruzzi, J. Sun, R. A. Monahan-Earley, I. Shiojima, J. A. Nagy, M. I. Lin, K. Walsh, A. M. Dvorak, D. M. Briscoe, M. Neeman, W. C. Sessa, H. F. Dvorak and L. E. Benjamin, Cancer Cell, 2006, 10(2), 159–170 CrossRef CAS PubMed.
- P. Vadas and B. Perelman, J. Allergy Clin. Immunol., 2012, 129(5), 1329–1333 CrossRef CAS PubMed.
- T. Kursad, Permeability Barrier Methods and Protocols, Springer Protocols, Humana Press, New York, 2011, pp. 258–260 Search PubMed.
- N. Ablat, D. Lv, R. Ren, Y. Xiaokaiti, X. Ma, X. Zhao, Y. Sun, H. Lei, J. Xu, Y. Ma, X. Qi, M. Ye, F. Xu, H. Han and X. Pu, Molecules, 2016, 21(9), E1107 CrossRef PubMed.
- A. T. Robinson, I. S. Fancher, V. Sudhahar, J. T. Bian, M. D. Cook, A. M. Mahmoud, M. M. Ali, M. Ushio-Fukai, M. D. Brown, T. Fukai and S. A. Phillips, Am. J. Physiol.: Heart Circ. Physiol., 2017, 312(5), H896–H906 CrossRef PubMed.
- C. C. Mariana, Anaphylaxis and hypersensitivity reactions, Humana Press, New York, 2011, pp. 55–57 Search PubMed.
- S. A. Bock, A. Munoz-Furlong and H. A. Sampson, J. Allergy Clin. Immunol., 2007, 119, 1016–1018 CrossRef PubMed.
- T. Wang, X. Ma, Y. Xing, S. Sun, H. Zhang, T. Stürmer, B. Wang, X. Li, H. Tang, L. Jiao and S. Zhai, Int. Arch. Allergy Immunol., 2017, 173(1), 51–60 CrossRef CAS PubMed.
- T. Kishi, H. Kawana, M. Sayama, K. Makide, A. Inoue, Y. Otani, T. Ohwada and J. Aoki, Biochem. Biophys. Rep., 2016, 8, 346–351 Search PubMed.
- S. Chakraborty, N. Kar, L. Kumari, A. De and T. Bera, Int. J. Nanomed., 2017, 12, 4849–4868 CrossRef PubMed.
- D. D. Metcalfe, R. D. Peavy and A. M. Gilfillan, J. Allergy Clin. Immunol., 2009, 124(4), 639–646 CrossRef CAS PubMed.
- O. Barreiro-Costa, A. Tobío, A. Alfonso and L. M. Botana, J. Cell. Biochem., 2014, 115(5), 896–909 CrossRef CAS PubMed.
- M. Serra-Pages, A. Olivera, R. Torres, C. Picado, F. Mora and J. Rivera, J. Leukocyte Biol., 2012, 92(6), 1155–1165 CrossRef CAS PubMed.
- R. L. Campbell, R. A. Nicklas and A. T. Sadosty, Ann. Allergy, Asthma, Immunol., 2014, 113(6), 599–608 CrossRef PubMed.
- J. Gavard, Cell Adhes. Migr., 2014, 8(2), 158–164 CrossRef.
- A. Sidibé and B. A. Imhof, Nat. Immunol., 2014, 15(3), 215–217 CrossRef PubMed.
- F. Wessel, M. Winderlich, M. Holm, M. Frye, R. Rivera-Galdos, M. Vockel, R. Linnepe, U. Ipe, A. Stadtmann, A. Zarbock, A. F. Nottebaum and D. Vestweber, Nat. Immunol., 2014, 15(3), 223–230 CrossRef CAS PubMed.
- H. Hudry-Clergeon, D. Stengel, E. Ninio and I. Vilgrain, FASEB J., 2005, 19(6), 512–520 CrossRef CAS PubMed.
Footnote |
† Electronic supplementary information (ESI) available. See DOI: 10.1039/c7ra09268g |
|
This journal is © The Royal Society of Chemistry 2017 |
Click here to see how this site uses Cookies. View our privacy policy here.