DOI:
10.1039/C7RA09608A
(Paper)
RSC Adv., 2017,
7, 50441-50448
Rheological properties of a β-1,3-polyglucuronic acid derivative from 4-acetamido-TEMPO-mediated oxidation of curdlan
Received
30th August 2017
, Accepted 24th October 2017
First published on 31st October 2017
Abstract
The rheological properties of a carboxylic curdlan bearing a β-1,3-polyglucuronic acid structure were investigated as a function of concentration and temperature. Carboxylic curdlan solutions exhibited shear thinning behavior that became more evident with increasing concentration. This behavior was further described by a consistent index (k) and a flow behavior index (n) based on the power law model. Activation energy (Ea) decreased with increasing concentration, as calculated by the Arrhenius rule. The mechanical spectra of carboxylic curdlan were classified as dilute solution (<1.0%, w/v), entanglement network (2.0%, w/v), and weak gel (4.0%, w/v or above) according to frequency sweep, complex viscosity η*, and tan
δ results. Moreover, changes in the storage modulus (G′) and the loss modulus (G′′) were found to be dependent on frequency and concentration. This finding suggested that carboxylic curdlan could be explored as a potential thickener or stabilizer for various applications in the food industry.
Introduction
Polysaccharides represent a major class of bioactive macromolecules derived from renewable sources, including plants, animals, and microorganisms. Owing to their excellent biocompatibility, biodegradability and biodiversity, a considerable number of polysaccharides and their derivatives, such as agar, alginate, carrageenan, xanthan, pectin, and carboxymethyl cellulose, among others, have been frequently employed in food systems as an important type of food hydrocolloid.1 These polysaccharides are used as thickening, stabilizing, gelling, texture-producing, emulsifying, crystallizing, and syneresis-preventing agents to improve food shelf life and maintain food flavor compounds.2–4 The functional properties of these hydrocolloids are closely related to their unique rheological properties. Rheological properties are generally defined as mechanical properties that cause deformation and the flow of material in the presence of a stress.5,6 Because a great number of foods are frequently in the liquid form during production and processing, rheological behavior is widely studied in the food science.7 The study of rheological characterizations is important for expanding the potential applications of polysaccharides in functional properties (such as in manufacture, storage, and texture). Moreover, the rheological properties of polysaccharides considerably influence their molecular structures and chain conformations.8,9 Specifically, in recent years, many naturally and synthetically occurring polyanion polysaccharides and their derivatives such as okra pectins,10 low-methoxyl pectin from sunflower head,11 fucoidan from sea cucumber Apostichopus japonicas,12 sulphated polysaccharides from chlorophytan seaweeds Ulva fasciata,13 acidic polysaccharide from boat-fruited sterculia seeds,14 phosphorylated exopolysaccharide produced by Sporidiobolus pararoseus JD-2,15 carboxymethyl curdlan,16 carboxymethyl guar gum,17 have been employed as gelling agents, thickeners, and emulsifiers owing to their unique rheological properties under various conditions. Therefore, to explore and facilitate industrial applications in food, medicine, and cosmetics, researchers should focus on searching for new sources of food hydrocolloids with greater efficiency and economy from natural or chemically synthesized polysaccharides and their derivatives.
Curdlan, a bacterial exopolysaccharide produced by Alcaligenes faecalis, possesses a linear structure composed entirely of β-(1,3)-D-glucan.18,19 Curdlan is widely used in food, pharmaceutical, and cosmetic applications because of its excellent bioactivity and characteristic rheological properties.20,21 This substance has been widely utilized as a food additive because of its unique ability to form either low-set (thermo-reversible) or high-set (thermo-irreversible) hydrogels based on heating temperature alone. In general, the low-set gel is typically obtained when the aqueous suspension is heated between 55 and 60 °C and then cooled below 40 °C, whereas the high-set gel is obtained when the aqueous suspension is heated above 80 °C.22 Zhang & Nishinari (2009) reported that DMSO solutions of curdlan at a critical concentration of 0.2% exhibited shear thinning behavior in shear flow.23 By contrast, the solutions exhibited strain thinning following strain thickening at concentrations above 0.2% but only strain thickening below 0.2%. Below their critical concentration, curdlan chains are not entangled; above this critical concentration, the chains are both entangled and associated. The insolubility of curdlan in water considerably limits its applications in food industry and biomedicine. Our group recently prepared water-soluble carboxylic curdlan derivatives bearing a β-1,3-polyglucuronic acid structure by using 4-acetamido-TEMPO/NaClO/NaClO2 system under mild conditions.24 The carboxylic curdlan produced by 4-acetamido-TEMPO-mediated oxidation at pH 4.8 and 40 °C for 4 h possessed a high molecular weight (MW) (>500 kDa) and exhibited a compact random coil structure in aqueous medium. To the best of our knowledge, however, no or limited efforts have been made to characterize the rheological properties of carboxylic curdlan obtained from 4-acetamido-mediated oxidation in aqueous solution.
In the present study, the rheological properties of carboxylic curdlan aqueous solutions at different concentrations were characterized on the basis of its corresponding flow and viscoelastic behaviors by using steady shear and dynamic oscillatory shear measurements. The temperature dependence of the rheological behaviors of carboxylic curdlan solutions was also evaluated in this paper.
Materials and methods
Carboxylic curdlan and solution preparation
Carboxylic curdlan bearing a β-1,3-polyglucuronic acid structure was prepared through 4-acetamido-TEMPO-mediated oxidation according to our previous study.24 The carboxylate content and weight-average MW of carboxylic curdlan are 2.07 mmol g−1 and 5.7 × 105 Da, respectively. The carboxylic curdlan exhibited a random coil conformation in aqueous solution.
Carboxylic curdlan aqueous solutions with various concentrations ranging from 0.25% to 6.0% (w/v) were prepared by dispersing a certain amount of the solid in deionized water under mild continuous, stirring for 3 h at room temperature, and then storing at 4 °C overnight for complete hydration prior to rheological measurements.
Steady shear test
All rheological measurements (steady shear and dynamic oscillatory tests) were conducted on a Discovery HR-1 hybrid rheometer (TA instruments, New Castle, USA) equipped with a cone and plane geometry system (cone diameter, 40 mm; angle, 1°; gap, 29 μm). The volume of each sample used was approximately 0.30 mL. The sample was equilibrated for 10 min prior to the test. During the experiments, a thin layer of silicon oil was applied to the edge of the sample to prevent sample dehydration. All rheological tests were conducted in triplicate, and Trios 3.0 software (available online) was utilized for data collection and analysis. For steady shear tests, carboxylic curdlan solutions of different concentrations (0.25–6.0%, w/v) were sheared continuously at rates ranging from 0.1 s−1 to 200 s−1 at 25 °C, and the apparent viscosity (η) was determined. Temperature ramp tests were conducted within the temperature range of 20–80 °C with a heating rate of 5 °C min−1 at the same shear rate (γ) range (0.1–200 s−1).
Dynamic oscillatory test
Dynamic strain sweeps between 0.1% and 200% for the studied concentrations were previously determined at a fixed angular frequency (ω, rad s−1) of 1 rad s−1 and 25 °C to establish the region of linear viscoelastic response, where the shear stress varies linearly with the applied strain. For oscillatory frequency sweep measurements, the storage modulus (G′, Pa), loss modulus (G′′, Pa), complex viscosity (η*, Pa) and tan
δ (G′′/G′) of each carboxylic curdlan solution were determined as a function of ω ranging from 0.1 rad s−1 to 100 rad s−1 with a strain of 2% and at 25 °C. Dynamic temperature sweeps were measured in the temperature range of 20–80 °C with a heating rate of 5 °C min−1 at a fixed ω of 1 rad s−1 and a strain of 2%.
Results and discussion
Steady shear properties
Fig. 1 shows the dependence of η on γ in carboxylic curdlan aqueous solutions at different concentrations ranging from 0.25% to 6.0% (w/v) at 25 °C. As expected, the apparent viscosity exhibited both concentration and shear rate dependence. Apparent viscosity increased with increasing polymer concentration, thereby demonstrating that viscosity strongly depends on concentration. At lower concentration (<0.5%, w/v), apparent viscosity virtually remained unchanged with increasing shear rate, thus suggesting that the carboxylic curdlan aqueous solution exhibits Newtonian flow behavior. With increasing concentration (>0.5%, w/v), viscosity decreased dramatically as the shear rate increased. Moreover, carboxylic curdlan aqueous solutions showed shear thinning behavior, and the non-Newtonian pseudoplastic behavior became more significant at higher concentrations (1.0%, w/v or above). In the dilute regime, individual polymer coils are so distantly located that the coils exert negligible influences on each other and are free to move independently. Thus, no reduction in apparent viscosity is observed, leading to the presence of Newtonian plateaus in the viscosity shear rate plots.25 However, in more concentrated solutions, the carboxylic curdlan chain inter- and/or intra-molecular interactions, including hydrogen bonds, hydrophobic interactions, van der Waals forces, among others, between hydroxyl and carboxylic groups become stronger; this observation is particularly true when the interactions involve hydrogen bonds.
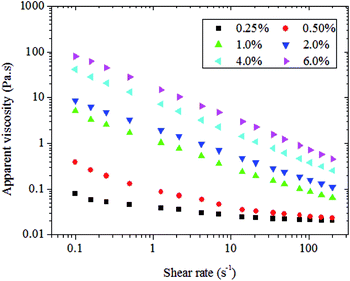 |
| Fig. 1 Dependence of apparent viscosity η on shear rate γ in carboxylic curdlan solutions under different conditions and at 25 °C. | |
The random coils of carboxylic curdlan can form a more entangled network or aggregate by intertwining with each other, leading to higher viscosity.26 At a certain shear rate, inter- and/or intra-molecular interactions were mechanically disrupted, resulting in apparent viscosity reductions, and a shear thinning nature was observed. As the shear rate increased, the crossing degree with each other further decreased, as confirmed by the decrease in apparent viscosity. Compared with carboxymethylated curdlan (CMc),16 the carboxylic curdlan aqueous solutions reported in this work exhibited more pronounced shear thinning behaviors and showed typical characteristics of weak gels. Our result suggested that such rheological feature played an important role in food and cosmetic products since it enhance sensory qualities and ensured a high degree of mixability, pumpability, and pourability during the processing and/or actual use of these products.27
The shear thinning behavior of carboxylic curdlan can be extensively described according to the power law model (eqn (1)) as follows:
where
σ is the shear stress (Pa),
k is the consistency coefficient (Pa s
n),
γ is the shear rate (s
−1), and
n is the flow behavior index. Power law fluids can be classified into three different types of fluids according to the value of
n. In general,
n < 1 for pseudoplastic or shear thinning fluids,
n = 1 for Newtonian fluid, and
n > 1 for dilatants or shear thickening fluids.
28 Table 1 summarizes the values of
k and
n of the carboxylic curdlan aqueous solutions as a function of polysaccharide concentration. The coefficients of determination (
R2) are close to 1 for the carboxylic curdlan solutions at various concentrations, thus suggesting that the power law model is highly workable for describing the flow behaviors of carboxylic curdlan solutions. At different concentrations, the values of
n were less than 1, indicating that the carboxylic curdlan solutions are all pseudoplastic or shear thinning fluids.
28,29 Furthermore, higher concentrations of the polysaccharide translated into smaller
n values. This trend reveals that the solutions deviated more from Newtonian fluids. Specifically, when the concentration exceeded 1.0%, the
n values of carboxylic curdlan solutions in the range of 0.30–0.51 suggested the stronger shear dependence of the solutions and a more pronounced pseudoplastic nature. These properties indicate potential applications in the food industry because carboxylic curdlan solutions offer a good perception of food in the mouth.
2,30 The
n values (0.30–0.51) of carboxylic curdlan solutions at concentrations exceeding 1.0% (w/v) were considerably lower than those of CMc solutions (0.75–0.86) at higher concentrations (>3.0%, w/v) and similar to those of curdlan suspensions (0.22–0.39).
16 These results show that the carboxylic curdlan solutions are more pronounced as pseudoplastic or shear thinning fluids than CMc solutions. In general,
k implies the viscous nature of the system. In other words, larger
k values indicate higher solid contents.
31 We can clearly see in
Table 1 that the
k values of the carboxylic curdlan solutions evidently increased with increasing concentration, which can be attributed to their increasing viscosity. With increasing concentration of the carboxylic curdlan solutions,
k values (from 0.027 Pa s
n to 18.59 Pa s
n) increased, whereas
n values (from 0.95 to 0.30) decreased. This result confirms that the carboxylic curdlan solutions show higher viscosity and a more remarkable pseudoplastic or shear thinning nature with increasing polysaccharide concentration. Similar phenomena have been observed in other polysaccharides.
29,32,33
Table 1 Power law parameters for carboxylic curdlan aqueous solutions under different conditions and at 25 °C
Concentration (%, w/v) |
k (Pa sn) |
n |
R2 |
6.0 |
18.59 |
0.30 |
0.999 |
4.0 |
7.34 |
0.36 |
0.998 |
2.0 |
1.84 |
0.47 |
0.999 |
1.0 |
0.85 |
0.51 |
0.999 |
0.5 |
0.05 |
0.85 |
0.999 |
0.25 |
0.027 |
0.95 |
0.999 |
Viscoelastic properties
To obtain valuable information on the molecular chain conformational characteristics of polysaccharides in solution, the viscoelastic behavior of the studied carboxylic curdlan aqueous solutions were investigated via dynamic oscillatory shear measurements. G′ and G′′ are indicative of the elasticity and viscosity of polymers, respectively, in dynamic oscillatory measurements. Fig. 2 shows the dependence of G′ and G′′ as a function of ω for carboxylic curdlan aqueous solutions at different concentrations (1.0–6.0%, w/v) and 25 °C. We clearly observed that both G′ and G′′ are strongly dependent on ω and that the effect of concentration on both moduli are significant. At lower concentrations (≤1.0%, w/v), G′′ always larger than G′ over the entire accessible frequency range; this result indicates that carboxylic curdlan aqueous solutions exhibit a dilute solution and cannot form a gel network in the dilute regime.34 Such finding may be due to the random coil chains of carboxylic curdlan being relatively free of each other, as well as weak or negligible interactions between chains in dilute solution. This result is in agreement with the results of steady shear tests. As the solution concentration increased to 2.0% (w/v), a crossover between G′ and G′′ occurred in the low ω range, and G′ became higher than G′′ as the ω increased. These findings imply that the transition from liquid-like to solid-like behaviors of carboxylic curdlan solutions occurred and indicate that carboxylic curdlan at 2.0% (w/v) features an entanglement network.9
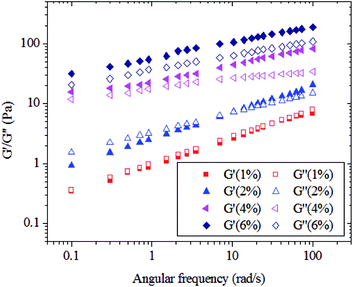 |
| Fig. 2 Plots of storage modulus G′ (solid symbols) and loss modulus G′′ (open symbols) against angular frequency ω in carboxylic curdlan solutions at different concentrations and 25 °C. | |
As concentration increased to 4.0% (w/v) or above, the crossover between G′ and G′′ disappeared, and G′ became higher than G′′ in the test frequency range. Moreover, the two moduli are consistently nearly parallel to each other as frequency increased. This result reveals that the carboxylic curdlan solution shows weak gel behavior and strongly implies that the carboxylic curdlan at or above 4.0% (w/v) is not only entangled but also mostly likely associated.35 Such association may subsequently lead to formation of weak networks in the aqueous solution because of strong interactions between carboxylic curdlan molecules. Similar results have been observed for galactomannan,36 exopolysaccharides from the fermentation medium of a Cordyceps sinensis fungus Cs-HK1,37 tara gum,29 and curdlan in DMSO,23 among others. Therefore, based on the above results, the mechanical spectra of carboxylic curdlan solution might be classified as a dilute solution (<1.0%, w/v), entanglement network (2.0%, w/v), and weak gel (4.0%, w/v or above).
The Cox–Merz plot can be used to compare steady shear and dynamic oscillatory shear measurements.38 The applicability of this approach may be attributed to the correlations between the shear rate dependence of η and the frequency dependence of η*. The η* parameter is derived from small-amplitude oscillatory shear testing that incorporates dynamic viscosity and dynamic rigidity. Moreover, the Cox–Merz function can be employed as an empirical equation to study the microstructure and/or interactions of polymer chains in terms of the degree of conformity with the function, as the plot suggests that η* can be superimposed on η.10 As shown in Fig. 3, the carboxylic curdlan solutions did not fulfill the Cox–Merz function at all concentrations studied because the steady viscosity differed from complex viscosity.
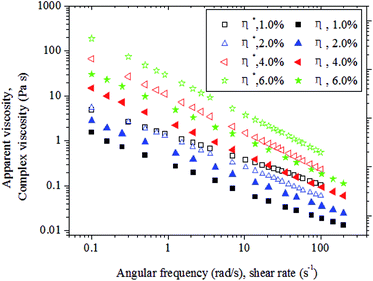 |
| Fig. 3 Cox–Merz plots of the carboxylic curdlan solutions at different concentrations and 25 °C. | |
Deviations from the Cox–Merz rule are usually due to the presence of hyper-entanglements and/or intermolecular chain aggregated structures that are broken down under an applied strain. Furthermore, in this work, η* consistently dominated η over the entire range of shear rates and angular frequencies studied. This finding indicates that the interactions of carboxylic curdlan chains can be broken down during steady shear measurements but remain unperturbed under oscillatory testing.39 As shown in Fig. 3, carboxylic curdlan solutions at the tested concentrations exhibit a shear dependent flow behavior, as η* decreased linearly with increasing ω in a double logarithmic scale. Therefore, we can conclude that the carboxylic curdlan solutions shows non-Newtonian shear thinning behavior at the tested concentrations; these results are accordance with those reported in previous literatures.10,40–42 More interestingly, it's worth noting that the departure deviation between η* and η of carboxylic curdlan aqueous solution at the tested concentrations was larger than those of okra pectins, tamarind seed gum and β-D-glucan from Ganoderma lucidum.10,42,43 This finding suggested that the carboxylic curdlan exhibited more prominent elastic gel-like structure as compared to those reported polysaccharides. The values of η* increased as concentration increased from 1.0% to 4.0% (w/v), thereby confirming the considerable potential of this hydrocolloid as a good thickener or stabilizer to increase the consistency of food systems.40 Higher solid contents have been reported to generally result in an increase in viscosity because of inhomogeneous flow and interfacial film formation.31
The tangent of the phase angle (tan
δ) is a common approach used to study the viscoelastic behavior of a polymer solution and is defined as the ratio of G′′ to G′. In general, tan
δ > 1 for predominantly viscous behavior, but <1 for predominantly elastic behavior. As reported in the literature,44 polymer solution systems present four common classifications according to the value of tan
δ. These classifications include dilute solutions, with high values of tan
δ that usually exceed 3, amorphous polymers, with values that can oscillate in the range of 0.2–0.3, and glassy crystalline polymers and gels, with low values near 0.01. Fig. 4 shows tan
δ as a function of ω for carboxylic curdlan at different concentrations and 25 °C. The tan
δ values of these aqueous solutions decreased with increasing ω, and the decrease in values of tan
δ became more obvious at higher concentrations. These results indicate that the gel structure of the carboxylic curdlan solution was formed and strengthened. A similar result has been observed for exopolysaccharides from fermentation medium of a C. sinensis fungus Cs-HK1.37
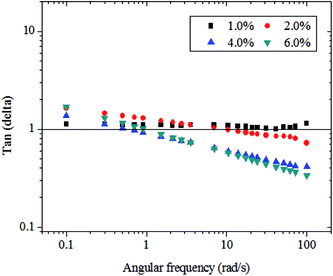 |
| Fig. 4 Plots of tan δ against angular frequency ω of carboxylic curdlan aqueous solutions at different concentrations and 25 °C. | |
When concentration was controlled to 1.0% and/or 2.0% (w/v), the mean values of tan
δ for carboxylic curdlan solutions exceeded 1.0 in the low frequency range, and tan
δ values slightly decreased as frequency increased. As concentration increased to 4.0% and 6.0% (w/v), the values of tan
δ were almost less than 1.0 within the frequency range of 1–100 rad s−1, maintaining G′ > G′′, and higher tested frequencies yielded smaller tan
δ values. This result suggests that carboxylic curdlan solutions at higher concentrations may display weak elastic gel-like behavior. Therefore, as mentioned above, the solid-like behavior of carboxylic curdlan may be expected to be more considerable with increasing concentration, which further implies the possible formation of weak gels for carboxylic curdlan at concentrations > 2.0% (w/v).
Temperature dependence in rheological properties of carboxylic curdlan
In food systems, temperature exerts an important influence on the rheological characterizations of food hydrocolloids. This influence may be attributed to temperature changes involved in the entire heating processes. Moreover, various food hydrocolloids display different temperature responses and vice versa. For instance, curdlan is widely used as a food additive for its unique capacity to form either thermo-reversible (<60 °C) or thermo-irreversible (>80 °C) hydrogels depending on heating temperature alone.22 Thus, the effects of temperature ranging from 20 °C to 80 °C on the apparent viscosity of carboxylic curdlan solutions at different concentrations were investigated in this study.
Fig. 5A shows the temperature dependence of the apparent viscosity of carboxylic curdlan solutions. We can clearly see that the apparent viscosity of carboxylic curdlan solutions decrease with increasing temperature. This tendency becomes more significant at higher concentrations, thus revealing that the carboxylic curdlan solutions are sensitive to temperature. This sensitivity may be due to enlargement of intermolecular chains and decreases in intermolecular associations, such as hydrogen bonds, hydrophobic interactions, and van der Waals forces, with intensifying thermal motion.41,45 As well, the molecular chains or conformational structures of carboxylic curdlan collapse or disintegrate with increasing temperature, possibly resulting in a decrease in viscosity. Similar results have been reported in previous studies.17,29
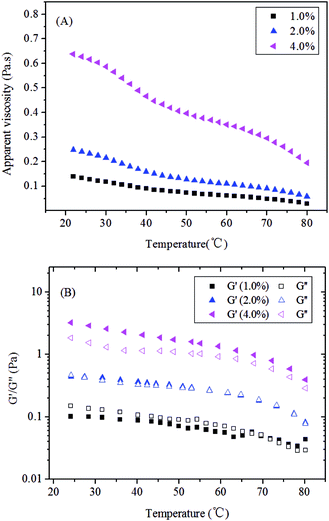 |
| Fig. 5 Temperature dependence of (A) apparent viscosity η at a shear rate of 50 s−1, (B) storage modulus G′, and loss modulus G′′ of carboxylic curdlan solutions at different concentrations. | |
In general, the Arrhenius relationship (eqn (2)) is often employed to evaluate the dependence of temperature on the flow behavior of various food hydrocolloids.2 The equation is as follows:
|
η = A exp(Ea/RT)
| (2) |
where
Ea is the activation energy of viscous flow (J mol
−1),
A is the frequency factor,
R is the universal gas constant, 8.314 (J mol
−1 K
−1), and
T is the temperature (K). A linear relationship between ln(
η) against (1/
T) was obtained according to
eqn (2), and the value of
Ea can be estimated from the slope of the fitting line. In the present study, the Arrhenius model (
eqn (2)) can reasonably describe the relationship between temperature and the viscosity of carboxylic curdlan solutions, yielding high
R2 values (>0.95). Based on the slope of the plots (
Table 2), the obtained
Ea values were calculated to be about 22.22, 21.18, and 19.27 kJ mol
−1 for carboxylic curdlan solutions of 1.0%, 2.0%, and 4.0% (w/v), respectively.
Table 2 Simulation results of carboxylic curdlan on the basis of the Arrhenius equation at various concentrations
Concentration (%, w/v) |
A0 |
Ea (kJ/mol) |
R2 |
1.0 |
1.66 × 10−5 |
22.22 |
0.958 |
2.0 |
4.45 × 10−5 |
21.18 |
0.978 |
4.0 |
2.43 × 10−4 |
19.27 |
0.949 |
Evidently, the value of Ea gradually decreased from 22.22 kJ mol−1 to 19.27 kJ mol−1 with increasing concentration from 1.0% to 4.0% (w/v), thereby suggesting greater temperature dependence of the carboxylic curdlan at lower concentrations. Ea generally reflects the degree of molecule movement, and higher Ea values show greater temperature dependence in flow viscosity.17 Interestingly, in agreement with our results, the Ea values of xanthan, gelation, and tara gum reportedly decreased with increasing concentration. However, the Ea values of several gums, such as carrageenan, starch, and pectin, increased with increasing concentration.2,29 This variation may be attributed to differences in the inherent structural characteristics of different polymers, which could lead to varied temperature effects on viscosities.
As reported in a previous study,46 the melting temperature of a food hydrocolloid may be defined as the temperature at which the dynamic shear G′ is equivalent to the shear G′′ (the sol–gel transition). This temperature can be easily measured in a controlled stress rheometer in oscillatory mode. The viscoelastic moduli are frequency dependent, and this dependence must be recognized when measuring the melting or gelling points.46 In this work, Fig. 5B shows the temperature dependence of the G′ and G′′ of the carboxylic curdlan solutions of 1.0%, 2.0%, and 4.0% (w/v) during heating at a constant heating rate (5 °C min−1). We observed that G′ decreased with increasing temperature from 20 °C to 80 °C, regardless of sample concentration, thereby implying melting of the network structure (or aggregates). Increasing temperature induced more effective destruction of intermolecular interactions, especially hydrogen bonds between carboxylic curdlan chains. Thus, the molecular mobility of the chains is increased. Similarly, Jin et al. has reported that the G′ of a curdlan aqueous suspension decreased within the temperature range of 40–50 °C. This trend is related with the swelling of the curdlan because of the breakup of hydrogen bonds.16 Additionally, the results presented in Fig. 5B are agreement with temperature stability experiments reported for galactomannans, that describe with temperature increase, a decrease in G′, attributed to heat induced intra- and inter-chain hydrogen bonds breakage.47,48
Possible mechanism of rheological behavior for carboxylic curdlan
As reported in our previous study,24 carboxylic curdlan bearing the β-1,3-polyglucuronic acid structure and obtained through 4-acetamido-TEMPO-mediated oxidation of curdlan exhibits a random coil conformation in aqueous solutions. Moreover, the material shows potential in the production of gene carriers, bio-nanomaterials, and other chiral nanowires through hydrogen bonding interactions, hydrophobic interactions, and electrostatic attraction.49 Thus, a possible mechanism underlying the rheological behavior of the carboxylic curdlan is proposed in Fig. 6.
 |
| Fig. 6 Schematic mechanism of rheological behavior for carboxylic curdlan. | |
In the present work, the studied carboxylic curdlan systems could be classified into three regions, namely, dilute solution (<1.0%, w/v), entanglement network (2.0%, w/v), and weak gel (4.0%, w/v or above), based on frequency sweep, η*, and tan
δ results. In the dilute regime, random carboxylic curdlan coils are relatively separate and isolated from each other. Therefore, the coils exert negligible influences on each other and are free to move independently. Thus, solution viscosity is independent of the shear rate, and G′′ is consistently higher than G′ over the entire frequency range studied. However, with increasing concentration (2.0%, w/v), molecular collisions become more frequent, and a point wherein the molecules begin to come into contact or overlap with one another and entangle permanently is eventually achieved.
The transition from a dilute solution of independently moving coils to an entangled network is usually accompanied by variations in the concentration dependence of solution viscosity (η) and moduli (G′ and G′′). At higher concentrations, intermolecular entanglements are strengthened, and aggregates are formed. In general, aggregates in higher polymer concentrations are mainly attributed to four different types of interactions, including hydrophobic interactions, hydrogen bonding, electrostatic interactions, and covalent bonds.50 In carboxylic curdlan, hydroxyl and carboxylate groups are exposed on the surface of coil chains. A high polymer concentration provides an approach to promote inter-polymer chain associations because of the high concentration of hydrogen bonding sites, which results in polysaccharide molecules being driven together to form agglomerates. Formation of junction zones is promoted, and relatively strong gels are obtained. Therefore, hydrogen bonds and van der Waals forces between molecules perform an important function in maintaining the viscoelasticity of carboxylic curdlan solutions.
Conclusions
In conclusion, the rheological properties of carboxylic curdlan bearing a β-1,3-polyglucuronic acid structure at different concentrations and temperatures were investigated by using rheological measurements under steady and oscillatory shear states. Steady shear tests demonstrated that aqueous carboxylic curdlan solution exhibits shear thinning behavior, which becomes more pronounced with increasing concentration. The power law model was used to describe the shear thinning behavior, and the k and n values were obtained. The Arrhenius equation was found to be highly workable for describing the effect of temperature on viscosity, and the obtained Ea decreased with increasing concentration. Dynamic shear oscillatory measurements showed both fluid-like (G′′ dominant) and solid-like (G′ dominant) behaviors depending on the concentration and frequency applied. The mechanical spectra of the carboxylic curdlan solutions were classified as dilute solution (<1.0%, w/v), entanglement network (2.0%, w/v), and weak gel (4.0%, w/v or above) according to the frequency sweep, η*, and tan
δ results. A possible mechanism of rheological behavior for carboxylic curdlan was also proposed. The results imply that carboxylic curdlan could be developed as a novel thickener or stabilizer for various applications in the food industry.
Conflicts of interest
There are no conflicts to declare.
Acknowledgements
This work was supported financially by the National Natural Science Foundation of China (31671812), the Natural Science Foundation of Jiangsu Province (BK20140542), the Priority Academic Program Development (PAPD) of Jiangsu Higher Education Institutions and Jiangsu Overseas Research & Training Program for University Prominent Young & Middle-aged Teachers and Presidents.
References
- S. W. Cui, Food Carbohydrates: Chemistry, physical properties, and applications, Taylor & Francis Group CRC Press, Boca Raton, 2005 Search PubMed
. - M. Marcotte, A. R. Taherian and H. S. Ramaswamy, Food Res. Int., 2001, 34, 695–704 CrossRef CAS
. - N. Rajapakse and S. K. Kim, Adv. Food Nutr. Res., 2011, 64, 17–28 CAS
. - J. R. Stokes, L. Macakova, A. Chojnicka-Paszun, C. G. de Krluif and H. de Jongh, Langmuir, 2011, 27, 3474–3484 CrossRef CAS PubMed
. - M. A. Rao, Flow and functional models for rheological properties of fluid foods, Springer, New York, 2014 Search PubMed
. - S. Sahin and S. G. Sumnu, Rheological properties of foods, Springer, New York, 2007 Search PubMed
. - G. Tabilo-Munizaga and G. V. Barbosa-Cánovas, J. Food Eng., 2005, 67, 147–156 CrossRef
. - Y. Tao and D. Feng, Food Hydrocolloids, 2012, 28, 151–158 CrossRef CAS
. - X. Xu, J. Xu, Y. Zhang and L. Zhang, Food Hydrocolloids, 2008, 22, 735–741 CrossRef CAS
. - V. Kontogiorgos, I. Margelou, N. Georgiadis and C. Ritzoulis, Food Hydrocolloids, 2012, 29, 356–362 CrossRef CAS
. - X. Hua, K. Wang, R. Yang, J. Kang and J. Zhang, Food Hydrocolloids, 2015, 44, 122–128 CrossRef CAS
. - L. Yu, C. Xue, Y. Chang, Y. Hu, X. Xu, L. Ge and G. Liu, Food Chem., 2015, 180, 71–76 CrossRef CAS PubMed
. - P. Shao, M. Qin, L. Han and P. Sun, Carbohydr. Polym., 2014, 113, 365–372 CrossRef CAS PubMed
. - Y. Wu, S. W. Cui, J. Wu, L. Ai, Q. Wang and J. Tang, Carbohydr. Polym., 2012, 88, 926–930 CrossRef CAS
. - M. Han, C. Du, Z. Y. Xu, H. Qian and W. G. Zhang, Int. J. Biol. Macromol., 2016, 88, 603–613 CrossRef CAS PubMed
. - Y. Jin, H. Zhang, Y. Yin and K. Nishinari, Carbohydr. Res., 2006, 341, 90–99 CrossRef CAS PubMed
. - H. Gong, M. Liu, J. Chen, F. Han, C. Gao and B. Zhang, Carbohydr. Polym., 2012, 88, 1015–1022 CrossRef CAS
. - T. Harada, M. Masada, K. Fujimori and I. Maeda, Agric. Biol. Chem., 1996, 30, 196–199 Search PubMed
. - T. Harada, A. Misaki and H. Saito, Arch. Biochem. Biophys., 1968, 124, 292–298 CrossRef CAS PubMed
. - B. C. Lehtovaara and F. X. Gu, J. Agric. Food Chem., 2011, 59, 6813–6828 CrossRef CAS PubMed
. - R. Zhang and K. J. Edgar, Biomacromolecules, 2014, 15, 1079–1096 CrossRef CAS PubMed
. - T. Funami, M. Funami, H. Yada and Y. Nakao, Food Hydrocolloids, 1999, 13, 317–324 CrossRef CAS
. - H. Zhang and K. Nishinari, Food Hydrocolloids, 2009, 23, 1570–1578 CrossRef CAS
. - J. K. Yan, H. L. Ma, P. F. Cai, H. N. Zhang, Q. Zhang, N. Z. Hu, X. B. Feng and J. Y. Wu, Food Chem., 2014, 14, 530–535 CrossRef PubMed
. - L. S. Lai, J. Tung and P. S. Lin, Food Hydrocolloids, 2000, 14, 287–294 CrossRef CAS
. - S. Fijan, R. J. Sostar-Turk and R. Lapasin, Carbohydr. Polym., 2007, 68, 708–717 CrossRef
. - L. Yu, X. Xu, J. Zhou, G. Lv and J. Chen, Food Hydrocolloids, 2017, 65, 165–174 CrossRef CAS
. - A. Haddarah, A. Bassal, A. Ismail, C. Gaiani, I. Ioannou, C. Charbonnel, T. Hamieh and M. Ghoul, J. Food Eng., 2014, 120, 204–214 CrossRef CAS
. - Y. Wu, W. Ding, L. Jia and Q. He, Food Chem., 2015, 168, 366–371 CrossRef CAS PubMed
. - F. L. Muller, J. P. Pain and P. Villon, On the behavior of non-newtonian liquids in collinear ohmic heaters, in Proceedings of the 10th International Heat Transfer Conference. Freezing, Melting, Internal Forces Convection and Heat Exhchangers, Brighton, UK, 1994, vol. 4, pp. 285–290 Search PubMed
. - M. Maskan and F. Gogus, J. Food Eng., 2000, 43, 173–177 CrossRef
. - M. I. Capitani, L. J. Corzo-Rios, L. A. Chel-Guerrero, D. A. Betancur-Ancona, S. M. Nolasco and M. C. Tomás, J. Food Eng., 2015, 149, 70–77 CrossRef
. - A. Farahnaky, E. Shanesazzadeh, G. Mesbahi and M. Majzoobi, J. Food Eng., 2013, 116, 782–788 CrossRef CAS
. - K. Nishinari, Colloid Polym. Sci., 1997, 275, 1093–1107 CAS
. - H. R. Moreira, F. Munarin, R. Gentilini, L. Visai, P. L. Granja, M. C. Tanzi and P. Petrini, Carbohydr. Polym., 2014, 103, 339–347 CrossRef CAS PubMed
. - P. B. S. Albuquerque, W. Barros, G. R. C. Santos, M. T. S. Correia, P. A. S. Mourão, J. A. Teixeira and M. G. Carneiro-da-Cunha, Carbohydr. Polym., 2014, 104, 127–134 CrossRef CAS PubMed
. - F. Sun, Q. Huang and J. Wu, Carbohydr. Polym., 2014, 114, 506–513 CrossRef CAS PubMed
. - W. P. Cox and E. H. Merz, J. Polym. Sci., 1958, 28, 619–622 CrossRef CAS
. - M. A. Rao, Measurement of flow and viscoelastic properties, in Rheology of fluid and semisolid foods: Principles and applications, ed. M. A. Rao, Gaithersburg: Aspen, 1999, pp. 59–152 Search PubMed
. - M. A. Hesarinejad, A. Koocheki and S. M. A. Razavi, Food Hydrocolloids, 2014, 35, 583–589 CrossRef CAS
. - W. W. Kim and B. Yoo, Int. J. Food Sci. Technol., 2009, 44, 503–509 CrossRef CAS
. - Y. Liu, J. Zhang, Q. Tang, Y. Yang, Y. Xia, S. Zhou, D. Wu, Z. Zhang, L. L. Dong and S. W. Cui, Food Hydrocolloids, 2016, 58, 120–125 CrossRef CAS
. - K. Khounvilay and W. Sittikijyothin, Food Hydrocolloids, 2012, 26, 334–338 CrossRef CAS
. - J. F. Steffe, Rheological Methods in Food Process Engineering, Freeman Press, East Lansing, MI, 1996, pp. 17–23 Search PubMed
. - R. Farhoosh and A. Riazi, Food Hydrocolloids, 2007, 21, 660–666 CrossRef CAS
. - M. Djabourov, J. Leblond and P. Papon, J. Phys., 1988, 49, 319–332 CAS
. - A. I. Bourbon, A. C. Pinheiro, C. Ribeiro, C. Miranda, J. M. Maia, J. A. Teixeira and A. A. Vicente, Food Hydrocolloids, 2010, 24, 184–192 CrossRef CAS
. - M. Rinaudo and A. Moroni, Food Hydrocolloids, 2009, 23, 1720–1728 CrossRef CAS
. - L. T. N. Lien, T. Shiraki, A. Dawn, Y. Tsuchiya, D. Tokunaga, S. Tamaru, N. Enomoto, J. Hojo and S. Shinkai, Org. Biomol. Chem., 2011, 9, 4266–4275 CAS
. - C. M. Bryant and D. J. McClements, Trends Food Sci. Technol., 1998, 9, 143–151 CrossRef CAS
.
|
This journal is © The Royal Society of Chemistry 2017 |
Click here to see how this site uses Cookies. View our privacy policy here.