DOI:
10.1039/C7RA09846D
(Paper)
RSC Adv., 2017,
7, 47848-47853
Anthocyanins from black wolfberry (Lycium ruthenicum Murr.) prevent inflammation and increase fecal fatty acid in diet-induced obese rats†
Received
4th September 2017
, Accepted 6th October 2017
First published on 11th October 2017
Abstract
This study aimed to determine whether black wolfberry (Lycium ruthenicum Murr.) anthocyanin (BWA) consumption can alleviate oxidative stress and reduce inflammation in high-fat diet-induced obese male Sprague-Dawley rats. In the present study, BWA at doses of 50, 100, and 200 mg kg−1 were supplemented in the daily food of obese rats during an eight-week experiment. Consumption of BWA at doses of 50, 100, and 200 mg kg−1 reduced bodyweight gain by 17.4%, 18.7 and 38.3%, respectively, in HFD-fed SD rats. BWA could effectively decrease Lee's index, improve the biochemical profiles, elevate superoxide dismutase activities and fecal fatty acid content, but significantly reduce malondialdehyde (MDA) production and down-regulate the expression of the TNFα, IL-6, NF-κB, IFN-γ and iNOS genes. Therefore, BWA potentially prevents diet-induced obesity by alleviating oxidative stress, reducing inflammation and accelerating fat decomposition in diet-induced obesity.
1. Introduction
Obesity and its associated metabolic disorders, including coronary heart disease, type II diabetes mellitus, reproductive and gastrointestinal cancers, have become global diseases that carry considerable morbidity and mortality.1–3 Obesity results from a prolonged energy imbalance, which is influenced by unhealthy eating habits, mood, and environmental as well as genetic factors.4 The currently available drugs for obesity exhibit adverse effects and high rates of secondary failure.5–7 Therefore, food ingredients with anti-obesity effects need to be developed.
Anthocyanin belongs to the widespread flavonoid group of polyphenols, which are commonly found in our daily diets, especially in berry fruits. In recent years, many animal studies have suggested that anthocyanin can decrease body weight, and reduce the risk for diabetes and cardiovascular diseases. Anthocyanin from black rice (Oryza sativa L.),8 black soybean (Glycine max L.),9,10 purple corn (Zea mays L.),11,12 purple sweet potato (Ipomoea batatas L.),13,14 blueberry (Vaccinium angustifolium),15,16 or mulberry (Morus australis Poir)17,18 prevented bodyweight gain and metabolic aberrations in the diet-induced obese animal models. In spite of the numerous published studies, the underlying fundamental mechanisms are still not fully understood.
Black wolfberry (Lycium ruthenicum Murr.) is a unique nutritional food because of the human health benefits, which widely distributes in the northwest of China.19,20 The ripe black wolfberries are rich in anthocyanins. To the best of our knowledge, the effects of black wolfberry anthocyanin (BWA) on weight loss have not been reported. Therefore, the present study aimed to investigate whether BWA alter bodyweight by alleviating oxidative stress and inflammation in diet-induced obesity.
2. Materials and methods
2.1 Materials
Black wolfberry was obtained from the agricultural logistics center in Qinghai. BWA was purified according to the process described by Wu et al.21 BWA is composed of petunidin-3-rutinoside-5-glucosides (78.6%), petunidin-3-glucoside-5-glucoside (8.7%), and delphinidin-3-rutinoside-5-glucoside (12.7%). All other chemicals were of reagent grade.
2.2 Experimental animals and supplement
All the experimental procedures were approved by the Committee on the Ethics of Animal Experiments of Tianjin University of Science & Technology (TUST20160202) and according to the National Institutes of Health Guide for Care and Use of Laboratory Animals. Sixty male Sprague-Dawley (SD) rats (SPF grade, body weight ∼ 130 g) were purchased from the Beijing Laboratory Animal Center of the Academy of Military Medical Sciences, and maintained in a room with an alternating 12 h/12 h light/dark cycle at 23 ± 3 °C, and provided free access to water and food.
After 7 days acclimation, the rats were divided in two groups including normal control group (NC, 12 rats) fed low fat diet (LFD) and high fat diet group (48 rats) based on a randomized block design. Rats in the HFD group were supplied with a HFD for 2 weeks to induce obesity, and then divided into 4 subgroups (12 rats): a control group provided with distilled water (MC), a group fed with HFD added with BWA at 50 mg kg−1, a group fed with HFD added with BWA at 100 mg kg−1, and a group fed with HFD added with BWA at 200 mg kg−1, respectively, for another 8 week experiment. During the experimental periods, no rat died and no untoward effects were observed. At the end of experiment, all rats were anesthetized with ketamine-HCl following a 12 h fast and then sacrificed by decapitation. Samples of serum, liver and adipose tissues were immediately collected and stored at −80 °C until the analytical procedures were carried out.
2.3 Serum parameter analyses
The rat serum parameters, including triglyceride (TG), total cholesterol (TC), high density lipoprotein cholesterol (HDLC), low density lipoprotein cholesterol (LDLC), malondialdehyde (MDA) levels, and superoxide dismutase (SOD) activities, were determined using commercially available kits (BioSino, China) in accordance with the manufacturer's instructions.
2.4 Hepatic biochemical analysis
Rat liver samples were homogenized in PBS, and lipids were subsequently determined using Folch et al. method.22 The concentrations of hepatic TG, TC, MDA levels and SOD activities were estimated using the same kit for serum analysis.
2.5 Fecal fatty acid analysis
The feces samples grinded and treated with chloroform–methanol (2
:
1, V/V), and then derived by sulfuric acid–methanol methyl esterification. The fatty acid composition and concentration of rat feces were analyzed according to Ahrens et al. method.23
2.6 Quantitative real-time PCR
The total RNA from the rat liver samples was extracted by trizol (Invitrogen Technologies, USA) according to the manufacturer's instruction. Then they were reverse transcribed into cDNA, and the expression of tumor necrosis factor α (TNFα), interleukin-6 (IL-6), interferon gamma (IFN-γ), nuclear factor κB (NF-κB), and inducible nitric oxide synthase (iNOS) genes were examined by PCR (Bio-Rad) using the One Step SYBR Prime Script PLUS RT-PCR kit (TaKaRa, Japan).
2.7 Statistical analysis
All experimental data were expressed as mean ± standard deviation (SD). The significance of treatment effects was analyzed using Duncan's multiple range tests after SPSS one-way ANOVA (SPSS PASW Statistic 19.0, SPSS Inc. Chicago, IL, USA). p < 0.05 was considered significant.
3. Results
3.1 Effects of BWA on the bodyweight gain of rats
When the rat fed with HFD were administered with BWA at 50 mg kg−1, 100 mg kg−1, 200 mg kg−1 for eight weeks, the body weight gain was effectively reduced by 17.4%, 18.7 and 38.3%, respectively, compared with MC group. No significant difference in the daily food intake was indicated among the HFD-fed group throughout the experimental period. However, BWA significantly decrease food utilization, and effectively low Lee's index compared with the MC group (Fig. 1). These data may suggest that the weight-reducing effects by BWA was not caused by appetite suppression, but rather attributed to decreasing food efficiency.24 In addition, the weights of the heart and kidney of the rat remained constant after the experiment. However, the liver and fat indexes of rat in the MC groups were higher than that of NC groups, feeding with BWA significantly improved these symptoms.
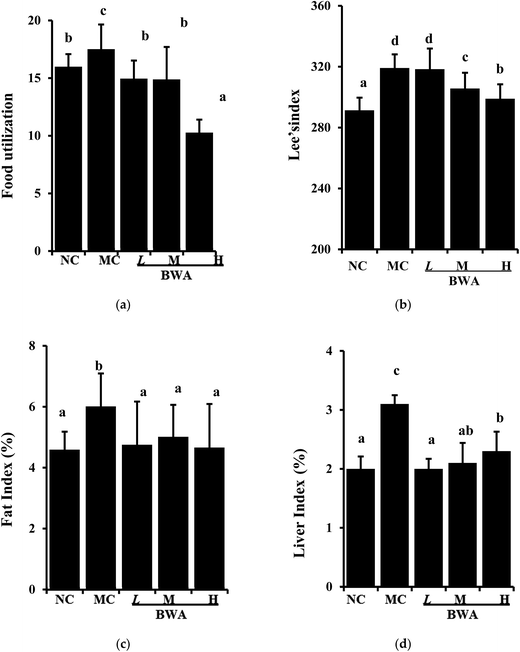 |
| Fig. 1 Effects of BWA on food utilization, Lee's index, fat and live index of male Sprague-Dawley rats. Fat index and liver index are referring to liver/fat weight/percentage. NC, normal control group; MC, high-fat diet control; LBWA, high-fat diet plus BWA at 50 mg kg−1; MBWA, high-fat diet plus BWA at 100 mg kg−1; HBWA, high-fat diet plus BWA at 200 mg kg−1. The means marked with different letters (a–d) are significantly differences compared with the other groups (p < 0.05). | |
3.2 Effects of BWA on serum parameters
Fig. 2 suggested that BWA at high dose effectively attenuated the serum TC and LDLC levels and significantly increased the concentration of HDLC levels and SOD activities relative to those in the MC group. Intake of BWA at middle dose remarkably elevated the HDLC levels and SOD activities, but did not alter the TC and LDLC levels. Furthermore, we found an interesting phenomenon that BWA at low dose notably decreased serum MDA levels than the other dose, which is contrary to the previous experiment. The reason for such differences remain unclear, they may be attributed to the composition and structure variations.25–27
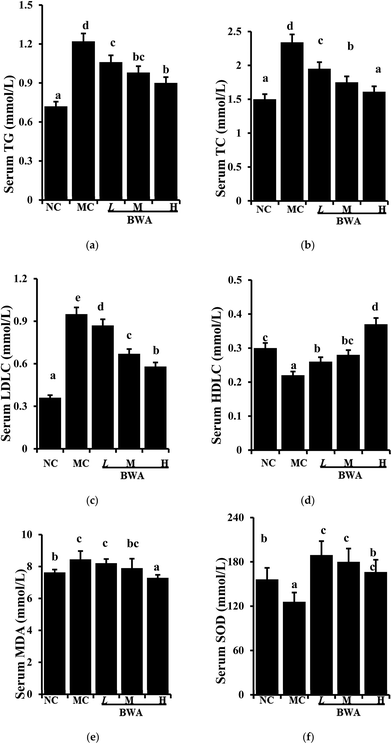 |
| Fig. 2 Effects of BWA on serum TG, TC, LDLC, HDLC, MDA, and SOD. The means marked with different letters (a–e) are significantly differences compared with the other groups (p < 0.05). | |
3.3 Effects of BWA on hepatic lipids and antioxidants
Fig. 3 showed the increased hepatic lipids of the rat fed with HFD relative to those fed with LFD; however, BWA consumption could decrease HFD-induced hepatic lipids. By contrast, BWA at high dose significantly decreased lipid TG and TC levels in the HFD-fed rat, but BWA at middle dose only decreased the TC levels. Moreover, SOD activities in the liver of rat in the BWA groups markedly increased relative to those in the MC groups. MDA levels, as an indicator of lipid peroxidation, were significantly higher in the MC group than those in the NC controls. However, MDA levels significantly decreased in the BWA treatment groups.
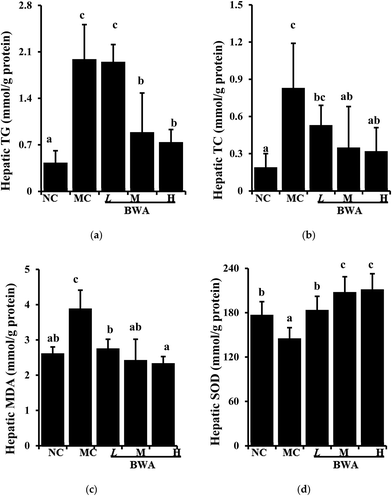 |
| Fig. 3 Effects of BWA on hepatic lipids and antioxidants. The means marked with different letters (a–e) are significantly differences compared with the other groups (p < 0.05). | |
3.4 Effects of BWA on fecal lipids
Fig. 4 suggested that the rat feces contained four types of long-chain fatty acids including palmitic acid, stearic acid, oleic acid and linoleic acid. The least amounts of these fatty acids were found in NC, followed by MC. The fecal lipid contents in the BWA groups were significantly higher than those in the MC group, which indicated BWA could inhibit the absorption of lipids in the gut.
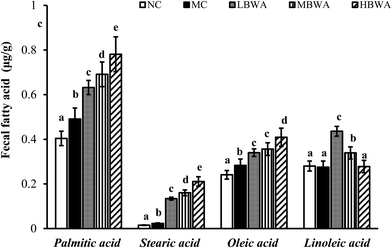 |
| Fig. 4 Effects of BWA on fecal fatty acid composition. The means marked with different letters (a–e) are significantly differences compared with the other groups (p < 0.05). | |
3.5 Effects of BWA on inflammation
Fig. 5 showed that rat fed with HFD significant increased the TNFα, IL-6, IFN-γ, iNOS, and NF-κB genes compared with those fed with LFD. BWA supplementation remarkably down-regulated expression levels of TNFα, IL-6, NF-κB, IFN-γ and iNOS genes compared with the MC group.
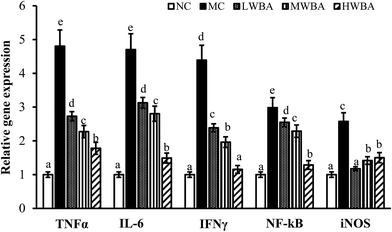 |
| Fig. 5 Effects of BWA on the expression of TNFα, IL-6, NF-κB, IFN-γ and iNOS genes in the liver tissue. The means marked with different letters (a–e) are significantly differences compared with the other groups (p < 0.05). | |
4. Discussion
Many studies have shown that fruit anthocyanin may contribute to reducing the risk of cardiovascular disease and can exert a variety of other human beneficial effects.27–31 However, studies on the specific mechanisms of how BWA exert anti-obesity have not been fully conducted. In the present study, we investigated whether BWA alter bodyweight by alleviating oxidative stress and inflammation in diet-induced obese male Sprague-Dawley rats.
Diets with relatively high-fat content long-term can induce overweight and adiposity in humans and animals.32,33 The SD rats fed with an HFD exhibited a significant bodyweight gain, higher Lee's index, an elevation of lipid profiles, a marked up-regulation of the expression of inflammatory cytokine levels, increased lipid deposition, a reduction in SOD activities. There might be dose-dependent effects of anthocyanin consumption on body weight gain.18,34 Supplementation with BWA at 200 mg kg−1 effectively attenuated body weight gain, markedly elevated the serum HDLC levels and total SOD activities, increased the fecal lipid contents, significantly decreased the hepatic lipids and MDA levels, markedly down-regulated expression levels of TNFα, IL-6, NF-κB, IFN-γ and iNOS genes.
Consistently, our current study demonstrated that BWA significantly decrease food utilization, and markedly increased the fecal lipid contents. This phenomenon suggests BWA may prevent bodyweight gain by inhibiting lipids absorption and accelerate fat decomposition in diet-induced obesity.
Epidemiological studies demonstrated that HFD-induced obesity and associated hepatic fat accumulation could increase oxidative stress, which increased MDA levels and decreased antioxidant enzyme activities.28,35,36 Numerous studies have documented the beneficial effects of polyphenols against oxidative stresses.37,38 In the present study, antioxidant properties in liver were significantly enhanced by BWA. Therefore, BWA can block the pathological processes of obesity by oxidative stress alleviation.
Accumulated evidence indicates that obesity is associated with a state of chronic inflammation in visceral adipose tissues, which elevate the inflammatory cytokine levels, such as TNFα, IL-6, NF-κB, IFN-γ and iNOS genes, as well as down-regulated expression levels of anti-inflammatory cytokines.39–41 In the current study, the HFD up-regulated expression levels of TNFα, IL-6, NF-κB, IFN-γ and iNOS genes, whereas BWA treatment significantly improved the inflammation. The mechanisms whereby anthocyanins exert anti-obesity effects are still not clear. Possible mechanisms suggested have included: attenuation the lipopolysaccharides (LPS)-induced nuclear factor-kappa B (NF-jB) translocation to the nucleus cellular and mediate inflammatory responses,42,43 reduction in food efficiency,44 inhibitory effects on adipogenesis,27 normalize the ratio between beneficial and pathogenic bacteria and plasma endotoxemia and reduce the impact of a high-fat diet on the host's metabolism.45,46
5. Conclusions
In conclusion, our results showed that supplementation with BWA at 200 mg kg−1 effectively attenuated body weight gain, markedly elevated the serum HDLC and T-SOD levels, increased the fecal fatty acid excretion, significantly decreased the hepatic lipids and MDA levels, marked down-regulated expression levels of TNFα, IL-6, NF-κB, IFN-γ and iNOS genes. Therefore, BWA can alter bodyweight by alleviating oxidative stress, reducing inflammation and accelerating fat decomposition in diet-induced obesity.
Conflicts of interest
There are no conflicts to declare.
Acknowledgements
This work is supported by the National Natural Science Foundation of China (31501475), the Youth Innovation Fund of Tianjin University of Science and Technology (2016LG16), Tianjin Natural Science Foundation (15JCQNJC46300), Tianjin Fundamental Research Funds for the Universities (2017KDZD02) and SKLCBSC-2014011.
References
- S. Yoshimoto, T. M. Loo, K. Atarashi, H. Kanda, S. Sato, S. Oyadomari, Y. Iwakura, K. Oshima, H. Morita, M. Hattori, K. Honda, Y. Ishikawa, E. Hara and N. Ohtani, Nature, 2013, 499, 97–101 CrossRef CAS PubMed.
- P. G. Kopelman, Nature, 2000, 404, 635–643 CrossRef CAS PubMed.
- K. Aleksandrova, M. Stelmach-Mardas and S. Schlesinger, in Obesity and Cancer, Springer, 2016, pp. 177–198 Search PubMed.
- A. R. Saltiel, Sci. Transl. Med., 2016, 8, 323 Search PubMed.
- K. Casazza, K. R. Fontaine, A. Astrup, L. L. Birch, A. W. Brown, M. M. Bohan Brown, N. Durant, G. Dutton, E. M. Foster, S. B. Heymsfield, K. McIver, T. Mehta, N. Menachemi, P. K. Newby, R. Pate, B. J. Rolls, B. Sen, D. L. Smith Jr, D. M. Thomas and D. B. Allison, N. Engl. J. Med., 2013, 368, 446–454 CrossRef CAS PubMed.
- E. E. Ladenheim, Drug Des., Dev. Ther., 2015, 9, 1867–1875 CrossRef PubMed.
- K. H. Kim and Y. Park, Annu. Rev. Food Sci. Technol., 2011, 2, 237–257 CrossRef CAS PubMed.
- S. L. Yao, Y. Xu, Y. Y. Zhang and Y. H. Lu, Food Funct., 2013, 4, 1602–1608 CAS.
- S. H. Kwon, I. S. Ahn, S. O. Kim, C. S. Kong, H. Y. Chung, M. S. Do and K. Y. Park, J. Med. Food, 2007, 10, 552–556 CrossRef CAS PubMed.
- H. K. Kim, J. N. Kim, S. N. Han, J. H. Nam, H. N. Na and T. J. Ha, Nutr. Res., 2012, 32, 770–777 CrossRef CAS PubMed.
- T. Tsuda, F. Horio, K. Uchida, H. Aoki and T. Osawa, J. Nutr., 2003, 133, 2125–2130 CAS.
- T. Wu, X. Guo, M. Zhang, L. Yang, R. Liu and J. Yin, Food Funct., 2017, 8, 3178–3186 CAS.
- S. J. Shi, U. J. Bae, S. O. Ka, S. J. Woo, S. O. Noh, Y. S. Kwon, K. H. Jung, J. H. Wee and B. H. Park, Int. J. Pharm., 2013, 9, 42–49 Search PubMed.
- Y. P. Hwang, J. H. Choi, E. H. Han, H. G. Kim, J. H. Wee, K. O. Jung, K. H. Jung, K. I. Kwon, T. C. Jeong, Y. C. Chung and H. G. Jeong, Nutr. Res., 2011, 31, 896–906 CrossRef CAS PubMed.
- I. C. Lee, D. Y. Kim and B. Y. Choi, J. Cancer Prev., 2014, 19, 209–215 CrossRef PubMed.
- T. Wu, Z. Jiang, J. Yin, H. Long and X. Zheng, Int. J. Food Sci. Nutr., 2016, 67, 257–264 CrossRef CAS PubMed.
- J. J. Chang, M. J. Hsu, H. P. Huang, D. J. Chung, Y. C. Chang and C. J. Wang, J. Agric. Food Chem., 2013, 61, 6069–6076 CrossRef CAS PubMed.
- T. Wu, X. Qi, Y. Liu, J. Guo, R. Zhu, W. Chen, X. Zheng and T. Yu, Food Chem., 2013, 141, 482–487 CrossRef CAS PubMed.
- J. Zheng, C. Ding, L. Wang, G. Li, J. Shi, H. Li, H. Wang and Y. Suo, Food Chem., 2011, 126, 859–865 CrossRef CAS.
- Y. Duan, F. Chen, X. Yao, J. Zhu, C. Wang, J. Zhang and X. Li, Int. J. Environ. Res. Public Health, 2015, 12, 8332–8347 CrossRef CAS PubMed.
- T. Wu, Q. Tang, Z. Gao, Z. Yu, H. Song, X. Zheng and W. Chen, PLoS One, 2013, 8, e77585 CAS.
- L. Folch, M. Lees and G. H. Sloane Stanley, J. Biol. Chem., 1957, 226, 497–509 Search PubMed.
- E. H. Ahrens Jr, W. Insull Jr, J. Hirsch, W. Stoffel, M. L. Peterson, J. W. Farquhar, T. Miller and H. J. Thomasson, Lancet, 1959, 1, 115–119 CrossRef CAS.
- H. Pan, Y. Gao and Y. Tu, Molecules, 2016, 21, 1659 CrossRef PubMed.
- K. Thompson, W. Pederick and A. B. Santhakumar, Food Funct., 2016, 7, 2169–2178 CAS.
- J. Overall, S. A. Bonney, M. Wilson, A. Beermann, M. H. Grace, D. Esposito, M. A. Lila and S. Komarnytsky, Int. J. Mol. Sci., 2017, 18, 422 CrossRef PubMed.
- D. Li, P. Wang, Y. Luo, M. Zhao and F. Chen, Crit. Rev. Food Sci. Nutr., 2017, 57, 1729–1741 CrossRef CAS PubMed.
- V. E. Atabay, M. Lutfioglu, B. Avci, E. E. Sakallioglu and A. Aydogdu, J. Periodontal Res., 2017, 52, 51–60 CrossRef CAS PubMed.
- A. Cassidy, G. Rogers, J. J. Peterson, J. T. Dwyer, H. Lin and P. F. Jacques, Am. J. Clin. Nutr., 2015, 102, 172–181 CrossRef CAS PubMed.
- B. Yousuf, K. Gul, A. A. Wani and P. Singh, Crit. Rev. Food Sci. Nutr., 2016, 56, 2223–2230 CrossRef CAS PubMed.
- Q. Ma, Y. Guo, L. Sun and Y. Zhuang, Nutrients, 2017, 9, 801 CrossRef PubMed.
- M. White, Obes. Rev., 2007, 8(suppl. 1), 99–107 CrossRef PubMed.
- D. Zhang, J. Christianson, Z. X. Liu, L. Tian, C. S. Choi, S. Neschen, J. Dong, P. A. Wood and G. I. Shulman, Cell Metab., 2010, 11, 402–411 CrossRef CAS PubMed.
- T. Wu, Z. Yu, Q. Tang, H. Song, Z. Gao, W. Chen and X. Zheng, Food Funct., 2013, 4, 1654–1661 CAS.
- A. Fernandez-Sanchez, E. Madrigal-Santillan, M. Bautista, J. Esquivel-Soto, A. Morales-Gonzalez, C. Esquivel-Chirino, I. Durante-Montiel, G. Sanchez-Rivera, C. Valadez-Vega and J. A. Morales-Gonzalez, Int. J. Mol. Sci., 2011, 12, 3117–3132 CrossRef CAS PubMed.
- I. Savini, V. Gasperi and M. V. Catani, in Obesity, Springer, 2016, pp. 65–86 Search PubMed.
- S. Khurana, M. Piche, A. Hollingsworth, K. Venkataraman and T. C. Tai, Can. J. Physiol. Pharmacol., 2013, 91, 198–212 CrossRef CAS PubMed.
- A. Zdarilova, A. Rajnochova Svobodova, K. Chytilova, V. Simanek and J. Ulrichova, Food Chem. Toxicol., 2010, 48, 1555–1561 CrossRef CAS PubMed.
- E. J. Park, J. H. Lee, G. Y. Yu, G. He, S. R. Ali, R. G. Holzer, C. H. Osterreicher, H. Takahashi and M. Karin, Cell, 2010, 140, 197–208 CrossRef CAS PubMed.
- T. Deng, C. J. Lyon, S. Bergin, M. A. Caligiuri and W. A. Hsueh, Annu. Rev. Phytopathol., 2016, 11, 421–449 CrossRef CAS PubMed.
- Y. Yu, Y. Wu, A. Szabo, Z. Wu, H. Wang, D. Li and X. F. Huang, Endocrinology, 2013, 154, 3130–3140 CrossRef CAS PubMed.
- R. J. Thoppil, D. Bhatia, K. F. Barnes, E. Haznagy-Radnai, J. Hohmann, A. S. Darvesh and A. Bishayee, Curr. Cancer Drug Targets, 2012, 12, 1244–1257 CAS.
- S. G. Lee, B. Kim, Y. Yang, T. X. Pham, Y.-K. Park, J. Manatou, S. I. Koo, O. K. Chun and J.-Y. Lee, J. Nutr. Biochem., 2014, 25, 404–411 CrossRef CAS PubMed.
- D. Esposito, T. Damsud, M. Wilson, M. H. Grace, R. Strauch, X. Li, M. A. Lila and S. Komarnytsky, J. Agric. Food Chem., 2015, 63, 6172–6180 CrossRef CAS PubMed.
- G. Jamar, D. Estadella and L. P. Pisani, BioFactors, 2017, 43, 507–516 CrossRef CAS PubMed.
- C. A. Morais, V. V. de Rosso, D. Estadella and L. P. Pisani, J. Nutr. Biochem., 2016, 33, 1–7 CrossRef CAS PubMed.
Footnote |
† Electronic supplementary information (ESI) available. See DOI: 10.1039/c7ra09846d |
|
This journal is © The Royal Society of Chemistry 2017 |
Click here to see how this site uses Cookies. View our privacy policy here.