DOI:
10.1039/C7RA11363C
(Paper)
RSC Adv., 2017,
7, 51313-51317
Cobalt(II)-catalyzed remote C5-selective C–H sulfonylation of quinolines via insertion of sulfur dioxide†
Received
15th October 2017
, Accepted 30th October 2017
First published on 3rd November 2017
Abstract
A novel and simple method for C–H sulfonylation of quinolines based on an inexpensive cobalt catalyst via insertion of sulfur dioxide is established. Excellent selectivity in the C5-position of quinolines is observed. This transformation has no need of oxidant and additive, affording sulfonated products in moderate to good yields. Furthermore, aromatic amines can displace aryldiazonium tetrafluoroborates as original materials via the in situ diazotization. The results of control experiments indicate that a radical pathway is involved in this sulfonylation.
Introduction
Heterocyclic aromatic sulfones are significant skeletons due to their extensive application in organic chemistry,1 and pharmaceutical chemistry2 as well as material chemistry.3 Hence, the development of procedures for sulfonylation has become increasingly significant in synthetic methodology. Classic synthetic routes to sulfones are the oxidation of thioether and the Friedel–Crafts reaction.4 Nevertheless, these typical reactions usually require harsh reaction conditions, including strong oxidants, strong acids and a high reaction temperature.
In recent decades, transition-metal-catalyzed C–H functionalization has become a novel and efficient strategy in the synthesis of various organic molecules.5 Especially, a series of synthetic methods have been exhibited for the preparation of sulfones by employing different substrates.6 In pioneering studies, Dong and co-workers disclosed a Pd(II)-catalyzed o-sulfonylation protocol which allowed the isolation of the o-sulfonylation products in good yields.7 As interesting as the former, Frost et al. developed Ru(II)-catalyzed sulfonylation of 2-phenylpyridines and obtained the m-sulfonylation product in considerable yield.8
For the past few years, owing to the special properties of quinolines,9 a series of researches were pursued by utilizing quinolines as raw materials for the C–H functionalization.10 Especially, the C5-functionalization of quinolines has achieved much attention. Prior works from many groups were focused on copper-catalyzed C–H functionalization11 or transition-metal-free oxidative coupling reaction with a stoichiometric amount of oxidants.12 But only a few examples were developed which employed iron,13 cobalt14 and nickel15 as catalyst.
Additionally, Among C5-functionalization of quinolines, the C5-sulfonylation has been successively reported by choosing sulfonyl chloride, sulfinates as well as sulfonhydrazide as the source of sulfonyl, respectively (Scheme 1, eqn (1)).16 Despite their utilities represent very inspiring progress, as mentioned above, almost all of them were catalyzed by copper catalyst. In addition, a stoichiometric amount of oxidants and additives were usually indispensable, not only increasing wastes, but also making this method inadaptable to large-scale synthesis. In recent years, the advance in the synthesis of sulfones via insertion of sulfur dioxide has been accomplished rapidly.17,18 Generally, the available DABCO·(SO2)2 and inorganic sulphites such as rongalite and potassium metabisulfite were used as the source of sulfur dioxide rather than toxic gaseous sulfur dioxide in organic reactions. Very recently, Wu and coworkers reported a copper-catalyzed sulfonylative C–H bond functionalization of quinolines from DABCO·(SO2)2 and aryldiazonium tetrafluoroborates.21l
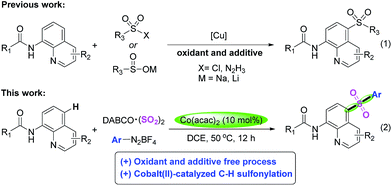 |
| Scheme 1 Summary of sulfonylation of quinoline amides. | |
Currently, the field of cobalt-catalyzed C–H functionalization has started to receive considerable attention due to its cheaper and more abundant characteristics.19 Herein, we report a cobalt(II)-catalyzed and convenient protocol for highly selective C5-sulfonylation of quinolines with DABCO·(SO2)2 and aryldiazonium salts to give the desired products in moderate to excellent yields under oxidant and additive free condition.
Results and discussion
Initially, the three-component reaction of N-(quinolin-8-yl)benzamide (1a), DABCO·(SO2)2 and p-tolyldiazonium tetrafluoroborate (2a) was selected as the model reaction for the development of the optimal reaction conditions. The desired C5-sulfonylated product (3a) was obtained in 49% yield by using CuI as catalyst in the presence of Na2CO3 in DCE for 12 h under N2 (Table 1, entry 1). Encouraged by this result, some metal catalysts including iron(III), iron(II), nickel(II), cobalt(II), copper(I) and copper(II) were studied (Table 1, entries 2–9), the yields of target product 3a was increased to 64% by using Co(acac)2 as catalyst (Table 1, entry 10). No product was formed in the absence of any metal catalyst (Table 1, entry 11). After that, we also screened several additives (Table 1, entries 12 and 13). Curiously, the higher yield was got in the absence of any additive (Table 1, entry 14). No better results were gained in further variations in solvents, temperature and so forth (Table 1, entries 15–20). Actually, we also screened the reaction condition by using Cu(acac)2 as a catalyst, the results were shown in ESI.†
Table 1 Screening of reaction conditions for sulfonylationa
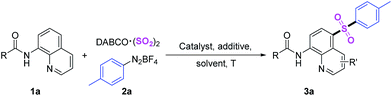
|
Entry |
Catalyst |
Additive |
Solvent |
Yieldb [%] |
Reaction conditions: 1a (0.2 mmol), catalyst (10 mol%), DABCO·(SO2)2 (1.2 equiv.), 2a (1.2 equiv.), DCE (1.0 mL), stirred at 50 °C, under N2, 12 h.
Isolated yields.
Under air.
Stirred at rt.
Stirred at 80 °C.
|
1 |
CuI |
Na2CO3 |
DCE |
49 |
2 |
Fe(OTf)3 |
Na2CO3 |
DCE |
13 |
3 |
Fe(OAc)2 |
Na2CO3 |
DCE |
Trace |
4 |
Ni(OTf)2 |
Na2CO3 |
DCE |
Trace |
5 |
CoF2 |
Na2CO3 |
DCE |
15 |
6 |
CoCl2 |
Na2CO3 |
DCE |
20 |
7 |
CoBr2 |
Na2CO3 |
DCE |
31 |
8 |
Co(NO3)2 |
Na2CO3 |
DCE |
43 |
9 |
Co(OAc)2 |
Na2CO3 |
DCE |
57 |
10 |
Co(acac)2 |
Na2CO3 |
DCE |
64 |
11 |
— |
Na2CO3 |
DCE |
0 |
12 |
Co(acac)2 |
NaHCO3 |
DCE |
62 |
13 |
Co(acac)2 |
AcOH |
DCE |
46 |
14
|
Co(acac)
2
|
—
|
DCE
|
80
|
15 |
Co(acac)2 |
— |
Dioxane |
53 |
16 |
Co(acac)2 |
— |
Toluene |
Trace |
17 |
Co(acac)2 |
— |
DMF |
14 |
18c |
Co(acac)2 |
— |
DCE |
42 |
19d |
Co(acac)2 |
— |
DCE |
53 |
20e |
Co(acac)2 |
— |
DCE |
78 |
After getting the optimized reaction condition, we next explored the scope of sulfonylation reaction of 2 with N-(quinolin-8-yl)benzamide and DABCO·(SO2)2 (Table 2). Numbers of aryl diazonium salts with different substituent groups were investigated. Overall, all the substrates could transform into corresponding products smoothly. By contrast, the compatibility of electron-donating groups on aryldiazonium tetrafluoroborates was better. Moreover, the molecular structure of product 3f was confirmed by X-ray crystallographic analysis. Product 3j was got in lower yield due to the steric-hindrance effect of 2,4,6-trimethylbenzene diazonium salt (Scheme 2).
Table 2 Substrate scope of aryl diazonium salts with 1aa
Reaction conditions: 1a (0.2 mmol), Co(acac)2 (10 mol%), DABCO·(SO2)2 (1.2 equiv.), 2 (1.2 equiv.), DCE (1.0 mL), stirred at 50 °C, under N2, 12 h, isolated yields.
|
|
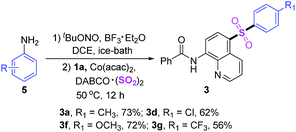 |
| Scheme 2 Sulfonylation of quinoline amides by using anilines as the starting materials. | |
After that, the sulfonylation reactions of p-tolyldiazonium tetrafluoroborate (2a), DABCO·(SO2)2 and quinoline amides were discussed (Table 3). The substituent effects on the benzene ring of quinoline amides revealed a lesser impact, both electron-donating and electron-withdrawing groups were tolerated in this reaction. The carboxamides with 2-thiazolyl, cyclohexyl as well as cyclopropyl furnished target products 3r, 3s and 3t in high yields too. In addition, the different substituent groups on quinoline ring were also researched. Corresponding products (3u–x) were got in ideal yield. Regrettably, product 3y was not detected because of the influence of ester group.
Table 3 Substrate scope of quinoline amides with 2aa
Reaction conditions: 1 (0.2 mmol), Co(acac)2 (10 mol%), DABCO·(SO2)2 (1.2 equiv.), 2a (1.2 equiv.), DCE (1.0 mL), stirred at 50 °C, under N2, 12 h, isolated yields.
|
|
Considering anilines are cheap and available materials, furthermore, the stability of aryldiazonium tetrafluoroborates are poor, therefore, we then investigated the possibility by using aromatic amines as original materials via the in situ diazotization. Interestingly, this reaction took place smoothly, which afforded desired products in moderate yields.
Subsequently, we studied the application values of this reaction (Scheme 3). Gram-scale synthesis was carried out under standard conditions, and sulfonated product was isolated in 69% yield. Obviously, the productive rate was reduced when the scale of reaction was amplified. Then hydrolysis reaction was performed, and the C5-sulfonated 8-aminoquinoline was acquired in 89% yield.
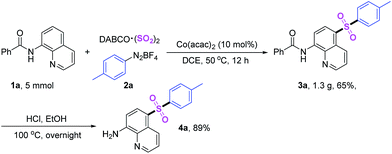 |
| Scheme 3 Gram-scale sulfonylation and synthetic applications. | |
Several control experiments were achieved in order to gain more deep understanding about the reaction mechanism. In the first place, three analogues (1x–z) were employed as substrates under the standard conditions and no products were detected, this result revealed that a free NH of amides and N atom of quinoline were crucial blocks for the sulfonylation (Scheme 4, eqn (1)). Next, TEMPO (2,2,6,6-tetramethyl-1-piperidinyloxy) and HQ (hydroquinone) were used as free radical inhibitor respectively, and the sulfonylation reaction was absolutely suppressed (Scheme 4, eqn (2)). Additionally, 32% yield of compound 7 was isolated when 1,1-diphenylethlene was utilized as trapping agent (Scheme 4, eqn (3)), declaring that a radical pathway was included. Finally, further test about kinetic isotope effects (KIE) gave a low ratio (k = 1.0) (Scheme 4, eqn (4)), suggesting that the rate determining step was not the process of cleavage of C–H bond.20
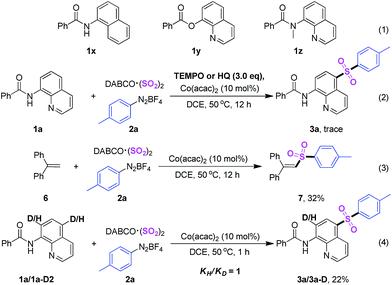 |
| Scheme 4 Investigation of the mechanism. | |
According to the experiment conclusions and previous reports,11–17,21 a plausible mechanism was proposed (Scheme 5). Initially, complex A was produced via the combination of LnCoII (D) and substrate 1. In the meantime, the sulfonyl radical was formed through insertion of sulphur dioxide.17f Subsequently, sulfonyl radical attacked intermediate A to afford complex B. After the generation of complex Cvia dehydrogenation process, desired product 3 was obtained through single electron transfer (SET) between complex C and tertiary amine cation radical.
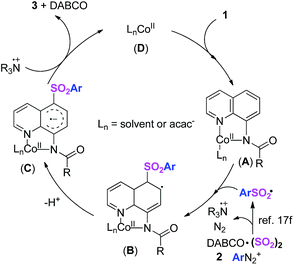 |
| Scheme 5 Plausible mechanism. | |
Conclusions
In conclusion, we have developed a cobalt(II)-catalyzed method for highly selective C5-sulfonylation of quinolines via insertion of sulfur dioxide under oxidant and additive free condition. This transformation proved a broad substrate scope and high efficiency. Furthermore, aromatic amines could displace aryldiazonium tetrafluoroborates as original materials via the in situ diazotization. Eventually, a single electron transfer (SET) mechanism was presented after verification of control experiments.
Conflicts of interest
There are no conflicts to declare.
Acknowledgements
This work was supported by the Projects of Medical and Health Technology Development Program in Shandong Province (No. 2015WS0102).
Notes and references
-
(a) Q. Lu, J. Zhang, F. Wei, Y. Qi, H. Wang, Z. Liu and A. Lei, Angew. Chem., Int. Ed., 2013, 52, 7156 CrossRef CAS;
(b) B. N. Rocke, K. B. Bahnck, M. Herr, S. Lavergne, V. Mascitti, C. Perreault, J. Polivkova and A. Shavnya, Org. Lett., 2014, 16, 154 CrossRef CAS;
(c) Q. Lu, J. Zhang, G. Zhao, Y. Qi, H. Wang and A. Lei, J. Am. Chem. Soc., 2013, 135, 11481 CrossRef CAS;
(d) T. Shen, Y. Yuan, S. Song and N. Jiao, Chem. Commun., 2014, 50, 4115 RSC.
-
(a) H. Rosen, R. Hajdu, L. Silver, H. Kropp, K. Dorso, J. Kohler, J. G. Sundelof, J. Huber, G. G. Hammond, J. J. Jackson, C. J. Gill, R. Thompson, B. A. Pelak, J. H. Epstein-Toney, G. Lankas, R. R. Wilkening, K. J. Wildonger, T. A. Blizzard, F. P. DiNinno, R. W. Ratcliffe, J. V. Heck, J. W. Kozarich and M. L. Hammond, Science, 1999, 283, 70 CrossRef;
(b) J. Colomb, G. Becker, S. Fieux, L. Zimmer and T. Billard, J. Med. Chem., 2014, 57, 3884 CrossRef CAS;
(c)
S. Patai, C. Z. Rappoport and J. M. Strirling, The Chemistry of Sulfones and Sulfoxides, Wiley, New York, 1988 Search PubMed;
(d) D. C. Meadows, T. Sanchez, N. Neamati, T. W. North and J. Gervay-Hague, Bioorg. Med. Chem., 2007, 15, 1127 CrossRef CAS.
- X. Codony, J. M. Vela and M. J. Ramírez, Curr. Opin. Pharmacol., 2011, 11, 94 CrossRef CAS.
-
(a) W. Su, Tetrahedron Lett., 1994, 35, 4955 CrossRef CAS;
(b) L. Xu, J. Cheng and M. L. Trudell, J. Org. Chem., 2003, 68, 5388 CrossRef CAS;
(c) N. Fukuda and T. Ikemoto, J. Org. Chem., 2010, 75, 4629 CrossRef CAS;
(d) L. Zhu, R. Qiu, X. Cao, S. Xiao, X. Xu, C.-T. Au and S.-F. Yin, Org. Lett., 2015, 17, 5528 CrossRef CAS;
(e) S. J. Nara, J. R. Harjani and M. M. Salunkhe, J. Org. Chem., 2001, 66, 8616 CrossRef CAS;
(f) R. P. Singh, R. M. Kamble, K. L. Chandra, P. Saravanan and V. K. Singh, Tetrahedron, 2001, 57, 241 CrossRef CAS;
(g) B. M. Graybill, J. Org. Chem., 1967, 32, 2931 CrossRef CAS;
(h) M. Ueda, K. Uchiyama and T. Kano, Synthesis, 1984, 323 CrossRef CAS.
-
(a) J. Yi, L. Yang, C.-G. Xia and F.-W. Li, J. Org. Chem., 2015, 80, 6213 CrossRef CAS;
(b) Z. Chen, B. Wang, J. Zhang, W. Yu, Z. Liu and Y. Zhang, Org. Chem. Front., 2015, 2, 1107 RSC;
(c) L. A. López and E. López, Dalton Trans., 2015, 44, 10128 RSC;
(d) M. Zhang, Y. Zhang, X. Jie, H. Zhao, G. Li and W. Su, Org. Chem. Front., 2014, 1, 843 RSC;
(e) Z. Huang, H. N. Lim, F. Mo, M. C. Young and G. Dong, Chem. Soc. Rev., 2015, 44, 7764 RSC;
(f) F. Wang, S. Yu and X. Li, Chem. Soc. Rev., 2016, 45, 6462 RSC;
(g) J. R. Hummel, J. A. Boerth and J. A. Ellman, Chem. Rev., 2017, 117, 9163 CrossRef CAS.
-
(a) C. Shen, P.-F. Zhang, Q. Sun, S.-Q. Bai, T. S. A. Hor and X.-G. Liu, Chem. Soc. Rev., 2015, 44, 291 RSC;
(b) S. Shaaban, S. Liang, N.-W. Liu and G. Manolikakes, Org. Biomol. Chem., 2017, 15, 1947 RSC;
(c) W.-H. Rao, B.-B. Zhan, K. Chen, P.-X. Ling, Z.-Z. Zhang and B.-F. Shi, Org. Lett., 2015, 17, 3552 CrossRef CAS.
- X. Zhao, E. Dimitrijević and V. M. Dong, J. Am. Chem. Soc., 2009, 131, 3466 CrossRef CAS.
- O. Saidi, J. Marafie, A. W. Ledger, P. M. Liu, M. F. Mahon, G. K. Kohn, M. K. Whittlesey and C. G. Frost, J. Am. Chem. Soc., 2011, 133, 19298 CrossRef CAS.
-
(a) J. P. Michael, Nat. Prod. Rep., 2008, 25, 166 RSC;
(b) K. Kaur, M. Jain, R. P. Reddy and R. Jain, Eur. J. Med. Chem., 2010, 45, 3245 CrossRef CAS;
(c) H. Jiang, J. E. Taggart, X. Zhang, D. M. Benbrook, S. E. Lind and W.-Q. Ding, Cancer Lett., 2011, 312, 11 CrossRef CAS;
(d) C. C. Hughes, J. B. MacMillan, S. P. Gaudencio, P. R. Jensen and W. Fenical, Angew. Chem., Int. Ed., 2009, 48, 725 CrossRef CAS;
(e) C. C. Hughe and W. Fenical, J. Am. Chem. Soc., 2010, 132, 2528 CrossRef;
(f) Y. Takayama, T. Yamada, S. Tatekabe and K. Nagasawa, Chem. Commun., 2013, 49, 6519 RSC;
(g) Y.-C. Liu, J.-H. Wei, Z.-F. Chen, M. Liu, Y.-Q. Gu, K.-B. Huang, Z.-Q. Li and H. Liang, Eur. J. Med. Chem., 2013, 69, 554 CrossRef CAS.
-
(a) C. Zhu, M. Yi, D. Wei, X. Chen, Y. Wu and X. Cui, Org. Lett., 2014, 16, 1840 CrossRef CAS PubMed;
(b) H. Wang, X. Cui, Y. Pei, Q. Zhang, J. Bai, D. Wei and Y. Wu, Chem. Commun., 2014, 50, 14409 RSC;
(c) G. Li, C. Jia and K. Sun, Org. Lett., 2013, 15, 5198 CrossRef CAS;
(d) L.-C. Campeau, D. R. Stuart, J.-P. Leclerc, M. Bertrand-Laperle, E. Villemure, H.-Y. Sun, S. Lasserre, N. Guimond, M. Lecavallier and K. Fagnou, J. Am. Chem. Soc., 2009, 131, 3291 CrossRef CAS;
(e) K. Sun, Y. Lv, J. Wang, J. Sun, L. Liu, M. Jia, X. Liu, Z. Li and X. Wang, Org. Lett., 2015, 17, 4408 CrossRef PubMed;
(f) X. Zhang, Z. Qi and X. Li, Angew. Chem., Int. Ed., 2014, 53, 10794 CrossRef CAS;
(g) J. Jeong, P. Patel, H. Hwang and S. Chang, Org. Lett., 2014, 16, 4598 CrossRef CAS;
(h) H. Hwang, J. Kim, J. Jeong and S. Chang, J. Am. Chem. Soc., 2014, 136, 10770 CrossRef CAS;
(i) Y. Su, X. Zhou, C. He, W. Zhang, X. Ling and X. Xiao, J. Org. Chem., 2016, 81, 4981 CrossRef CAS;
(j) K. Sun, X.-L. Chen, X. Li, L.-B. Qu, W.-Z. Bi, X. Chen, H.-L. Ma, S.-T. Zhang, B.-W. Han, Y.-F. Zhao and C.-J. Li, Chem. Commun., 2015, 51, 12111 RSC;
(k) B. Du, P. Qian, Y. Wang, H. Mei, J. Han and Y. Pan, Org. Lett., 2016, 18, 4144 CrossRef CAS;
(l) B. Ying, J. Xu, X. Zhu, C. Shen and P. Zhang, ChemCatChem, 2016, 8, 2604 CrossRef CAS.
-
(a) Y. Dou, Z. Xie, Z. Sun, H. Fang, C. Shen, P. Zhang and Q. Zhu, ChemCatChem, 2016, 8, 3570 CrossRef CAS;
(b) Y. Yin, J. Xie, F.-Q. Huang, L.-W. Qi and B. Zhang, Adv. Synth. Catal., 2016, 359, 1037 CrossRef;
(c) H. Yi, H. Chen, C. Bian, Z. Tang, A. K. Singh, X. Qi, X. Yue, Y. Lan, J.-F. Leec and A. Lei, Chem. Commun., 2017, 53, 6736 RSC;
(d) A. M. Suess, M. Z. Ertem, C. J. Cramer and S. S. Stahl, J. Am. Chem. Soc., 2013, 135, 9797 CrossRef CAS;
(e) X. He, Y.-Z. Xu, L.-X. Kong, H.-H. Wu, D.-Z. Ji, Z.-B. Wang, Y.-G. Xu and Q.-H. Zhu, Org. Chem. Front., 2017, 4, 1046 RSC;
(f) J. Xu, X. Zhu, G. Zhou, B. Ying, P. Ye, L. Su, C. Shen and P. Zhang, Org. Biomol. Chem., 2016, 14, 3016 RSC;
(g) C. Shen, J. Xu, B. Ying and P. Zhang, ChemCatChem, 2016, 8, 3560 CrossRef CAS;
(h) L.-K. Jin, G.-P. Lu and C. Cai, Org. Chem. Front., 2016, 3, 1309 RSC;
(i) C. Xia, K. Wang, J. Xu, C. Shen, D. Sun, H. Li, G. Wang and P. Zhang, Org. Biomol. Chem., 2017, 15, 531 RSC.
-
(a) D. Ji, X. He, Y. Xu, Z. Xu, Y. Bian, W. Liu, Q. Zhu and Y. Xu, Org. Lett., 2016, 18, 4478 CrossRef CAS;
(b) Y. Wang, Y. Wang, K. Jiang, Q. Zhang and D. Li, Org. Biomol. Chem., 2016, 14, 10180 RSC;
(c) Z. Wu, Y. He, C. Ma, X. Zhou, X. Liu, Y. Li, T. Hu, P. Wen and G. Huang, Asian J. Org. Chem., 2016, 5, 724 CrossRef CAS;
(d) Y. Wang, Y. Wang, Q. Zhang and D. Li, Org. Chem. Front., 2017, 4, 514 RSC.
-
(a) X. Cong and X. Zeng, Org. Lett., 2014, 16, 3716 CrossRef CAS;
(b) Y. He, N. Zhao, L. Qiu, X. Zhang and X. Fan, Org. Lett., 2016, 18, 6054 CrossRef CAS;
(c) H. Qiao, S. Sun, F. Yang, Y. Zhu, J. Kang, Y. Wu and Y. Wu, Adv. Synth. Catal., 2017, 359, 1976 CrossRef.
- C. J. Whiteoak, O. Planas, A. Company and X. Ribas, Adv. Synth. Catal., 2016, 358, 1679 CrossRef CAS.
-
(a) H. Chen, P. Li, M. Wang and L. Wang, Org. Lett., 2016, 18, 4794 CrossRef CAS;
(b) J. Ding, Y. Zhang and J. Li, Org. Chem. Front., 2017, 4, 1528 RSC.
-
(a) H.-W. Liang, K. Jiang, W. Ding, Y. Yuan, L. Shuai, Y.-C. Chen and Y. Wei, Chem. Commun., 2015, 51, 16928 RSC;
(b) J. Xu, C. Shen, X. Zhu, P. Zhang, M. J. Ajitha, K.-W. Huang, Z. An and X. Liu, Chem.–Asian J., 2016, 11, 882 CrossRef CAS;
(c) H. Qiao, S. Sun, F. Yang, Y. Zhu, W. Zhu, Y. Dong, Y. Wu, X. Kong, L. Jiang and Y. Wu, Org. Lett., 2015, 17, 6086 CrossRef CAS;
(d) J. Wei, J. Jiang, X. Xiao, D. Lin, Y. Deng, Z. Ke, H. Jiang and W. Zeng, J. Org. Chem., 2016, 81, 946 CrossRef CAS;
(e) J.-M. Li, J. Weng, G. Lu and A. S. C. Chan, Tetrahedron Lett., 2016, 57, 2121 CrossRef CAS;
(f) C. Xia, K. Wang, J. Xu, Z. Wei, C. Shen, G. Duan, Q. Zhu and P. Zhang, RSC Adv., 2016, 6, 37173 RSC;
(g) S. Liang and G. Manolikakes, Adv. Synth. Catal., 2016, 358, 2371 CrossRef CAS;
(h) G. Chen, X. Zhang, Z. Zeng, W. Peng, Q. Liang and J. Liu, ChemistrySelect, 2017, 2, 1979 CrossRef CAS.
-
(a) K. Zhou, H. Xia and J. Wu, Org. Chem. Front., 2017, 4, 1121 RSC;
(b) J. Sheng, Y. Li and G. Qiu, Org. Chem. Front., 2017, 4, 95 RSC;
(c) T. Liu, D. Zheng and J. Wu, Org. Chem. Front., 2017, 4, 1079 RSC;
(d) R. Mao, Z. Yuan, R. Zhang, Y. Ding, X. Fan and J. Wu, Org. Chem. Front., 2016, 3, 1498 RSC;
(e) Y. Chen and M. C. Willis, Chem. Sci., 2017, 8, 3249 RSC;
(f) D. Zheng, J. Yu and J. Wu, Angew. Chem., Int. Ed., 2016, 55, 11925 CrossRef CAS;
(g) A. L. Tribby, I. Rodríguez, S. Shariffudin and N. D. Ball, J. Org. Chem., 2017, 82, 2294 CrossRef CAS;
(h) B. Nguyen, E. J. Emmet and M. C. Willis, J. Am. Chem. Soc., 2010, 132, 16372 CrossRef CAS;
(i) A. S. Deeming, C. J. Russell and M. C. Willis, Angew. Chem., Int. Ed., 2016, 55, 747 CrossRef CAS;
(j) A. Shavnya, S. B. Coffey, K. D. Hesp, S. C. Ross and A. S. Tsai, Org. Lett., 2016, 18, 5848 CrossRef CAS;
(k) Y. Wang, B. Du, W. Sha, H. Mei, J. Han and Y. Pan, Org. Chem. Front., 2017, 4, 1313 RSC;
(l) A. Shavnya, S. B. Coffey, A. C. Smith and V. Mascitti, Org. Lett., 2013, 15, 6226 CrossRef CAS;
(m) M. W. Johnson, S. W. Bagley, N. P. Mankad, R. G. Bergman, V. Mascitti and F. D. Toste, Angew. Chem., Int. Ed., 2014, 53, 4404 CrossRef CAS;
(n) A. Shavnya, K. D. Hesp, V. Mascitti and A. C. Smith, Angew. Chem., Int. Ed., 2015, 54, 13571 CrossRef CAS;
(o) E. J. Emmet, C. S. Richards, D. Taylor, B. Nguyen, A. Garcia, D. Rubia, R. Hayter and M. C. Willis, Org. Biomol. Chem., 2012, 10, 4007 RSC;
(p) L. Martial, Synlett, 2013, 24, 1595 CrossRef;
(q) X. Wang, L. Xue and Z. Wang, Org. Lett., 2014, 16, 4056 CrossRef CAS;
(r) A. S. Deeming, C. J. Russell and M. C. Willis, Angew. Chem., Int. Ed., 2015, 54, 1168 CrossRef CAS;
(s) C. C. Chen and J. Waser, Org. Lett., 2015, 17, 736 CrossRef CAS;
(t) E. J. Emmett, B. R. Hayter and M. C. Willis, Angew. Chem., Int. Ed., 2013, 52, 12679 CrossRef CAS;
(u) E. J. Emmett, B. R. Hayter and M. C. Willis, Angew. Chem., Int. Ed., 2014, 53, 10204 CrossRef CAS;
(v) W. Zhang and M. Luo, Chem. Commun., 2016, 52, 2980 RSC;
(w) N. Wolff, J. Char, X. Frogneux and T. Cantat, Angew. Chem., Int. Ed., 2017, 56, 5616 CrossRef;
(x) H. Konishi, H. Tanaka and K. Manabe, Org. Lett., 2017, 19, 1578 CrossRef CAS;
(y) S. Ye and J. Wu, Chem. Commun., 2012, 48, 10037 RSC.
-
(a) G. Liu, C. Fan and J. Wu, Org. Biomol. Chem., 2015, 13, 1592 RSC;
(b) P. Bisseret and N. Blanchard, Org. Biomol. Chem., 2013, 11, 5393 RSC;
(c) A. S. Deeming, E. J. Emmett, C. S. Richards, D. Taylor and M. C. Willis, Synthesis, 2014, 46, 2701 CrossRef;
(d)
D. Zheng and J. Wu, Sulfur Dioxide Insertion Reactions for Organic Synthesis, Nature Springer, 2017 CrossRef.
-
(a) M. Moselage, J. Li and L. Ackermann, ACS Catal., 2016, 6, 498 CrossRef CAS;
(b) D. Wei, X. Zhu, J.-L. Niu and M.-P. Song, ChemCatChem, 2016, 8, 1242 CrossRef CAS;
(c) L. Ackermann, J. Org. Chem., 2014, 79, 8948 CrossRef CAS;
(d) J. Li, M. Tang, L. Zang, X. Zhang, Z. Zhang and L. Ackermann, Org. Lett., 2016, 18, 2742 CrossRef CAS;
(e) H. Wang, M. M. Lorion and L. Ackermann, ACS Catal., 2017, 7, 3430 CrossRef CAS;
(f) D. Zheng, M. Chen, L. Yao and J. Wu, Org. Chem. Front., 2016, 3, 985 RSC.
-
(a) M. Gûmez-Gallego and M. A. Sierra, Chem. Rev., 2011, 111, 4857 CrossRef;
(b) E. M. Simmons and J. F. Hartwig, Angew. Chem., Int. Ed., 2012, 51, 3066 CrossRef CAS.
-
(a) Y. Li, R. Mao and J. Wu, Org. Lett., 2017, 19, 4472 CrossRef CAS;
(b) Y. Xiang, Y. Li, Y. Kuang and J. Wu, Chem.–Eur. J., 2017, 23, 1032 CrossRef CAS PubMed;
(c) Y. Xiang, Y. Kuang and J. Wu, Chem.–Eur. J., 2017, 23, 6996 CrossRef CAS;
(d) R. Mao, Z. Yuan, Y. Li and J. Wu, Chem.–Eur. J., 2017, 23, 8176 CrossRef CAS;
(e) J. Zhang, Y. An and J. Wu, Chem.–Eur. J., 2017, 23, 9477 CrossRef CAS;
(f) T. Liu, D. Zheng, Z. Li and J. Wu, Adv. Synth. Catal., 2017, 359, 2653 CrossRef CAS;
(g) X. Gong, Y. Ding, X. Fan and J. Wu, Adv. Synth. Catal., 2017, 359, 2999 CrossRef CAS;
(h) T. Liu, D. Zheng, Y. Ding, X. Fan and J. Wu, Chem.–Asian J., 2017, 12, 465 CrossRef CAS;
(i) J. Yu, R. Mao, Q. Wang and J. Wu, Org. Chem. Front., 2017, 4, 617 RSC;
(j) Y. An, J. Zhang, H. Xia and J. Wu, Org. Chem. Front., 2017, 4, 1318 RSC;
(k) X. Wang, T. Liu, D. Zheng, Q. Zhong and J. Wu, Org. Chem. Front., 2017 10.1039/C7QO00787F;
(l) H. Xia, Y. An, X. Zeng and J. Wu, Org. Chem. Front., 2017 10.1039/C7QO00866J;
(m) K. Zhou, M. Chen, L. Yao and J. Wu, Org. Chem. Front., 2017 10.1039/C7QO00811B;
(n) D. Zheng, Y. An, Z. Li and J. Wu, Angew. Chem., Int. Ed., 2014, 53, 2451 CrossRef CAS.
Footnote |
† Electronic supplementary information (ESI) available: Detailed experimental procedures and analytical data. CCDC 1565132 for 3f. For ESI and crystallographic data in CIF or other electronic format see DOI: 10.1039/c7ra11363c |
|
This journal is © The Royal Society of Chemistry 2017 |
Click here to see how this site uses Cookies. View our privacy policy here.