DOI:
10.1039/C7SC01732D
(Edge Article)
Chem. Sci., 2017,
8, 5299-5304
Pivalophenone imine as a benzonitrile surrogate for directed C–H bond functionalization†
Received
19th April 2017
, Accepted 29th May 2017
First published on 30th May 2017
Abstract
Pivalophenone N–H imine has been found to serve as a prominent substrate for directed C–H alkylation and arylation reactions with alkyl bromides and aryl chlorides, respectively, under cobalt–N-heterocyclic carbene (NHC) catalysis. Unlike the case of the parent pivalophenone imine, the increased steric bulk of the resulting ortho-substituted pivalophenone imines allows them to undergo clean imine-to-nitrile conversion under peroxide photolysis or aerobic copper catalysis conditions. Overall, these two-step transformations offer convenient synthetic methods for ortho-functionalized benzonitriles.
Introduction
Transition metal-catalyzed, directing group-assisted C–H bond activation represents a powerful means to transform C–H bonds into C–C and C–heteroatom bonds with predictable regioselectivity.1 The utility of such transformations should largely depend on the availability and the versatility of the directing group. In this context, a cyano group can potentially be a very useful directing group, owing to its presence in a large number of commercial chemicals as well as to its applicability to various synthetic transformations. Following the seminal report of Murai and coworkers on the Ru-catalyzed addition of benzonitrile to vinylsilane,2 several examples of ortho-functionalization of benzonitriles, such as Pd-catalyzed arylation,3 alkoxylation,4 halogenation,5 and Ru-catalyzed olefination,6 have been reported to date (Scheme 1a). However, expansion of the scope of such transformations appears nontrivial because of intrinsic competition between π- (side-on) and σ- (end-on) coordination modes of the cyano group, only the former being suitable for ortho C–H activation (Scheme 1b).7 As such, the development of a readily accessible and transformable directing group that functions as a cyano group equivalent would be attractive.8 This would be particularly the case for arene C–H alkylation using alkyl electrophiles, because, when compared to C–H arylation and olefination,9 directing groups for this reaction manifold are still limited regardless of the recent progress made by palladium,10 ruthenium,11 nickel,12 cobalt,13 and iron14 catalysts.15
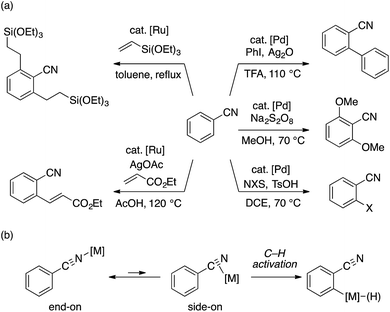 |
| Scheme 1 Cyano group-directed arene C–H bond functionalizations: examples and intrinsic difficulty. | |
Recently, we demonstrated that aryl N–H imines, typically derived from the corresponding aryl nitriles and organolithium or Grignard reagents, smoothly participate in the cobalt-catalyzed directed hydroarylation of alkenes (Scheme 2a),16 which represents a rare example of N–H imine-directed C–H functionalization that results in the retention of the imine directing group.17 On our way to extend the utility of the N–H imine as a directing group for C–H functionalization, we became interested in the potential utility of the N–H imine functional group as a nitrile surrogate. In particular, our attention was attracted to the seminal work of Ingold on the reaction of N–H imines under peroxide photolysis conditions (Scheme 2b).18 Their study revealed that di-tert-butyl imine ((t-Bu)2C = NH) undergoes facile fragmentation into pivalonitrile (t-BuCN) and tert-butyl radical, while sterically less hindered N–H imines undergo dimerization of the corresponding iminyl radicals to form azine derivatives.
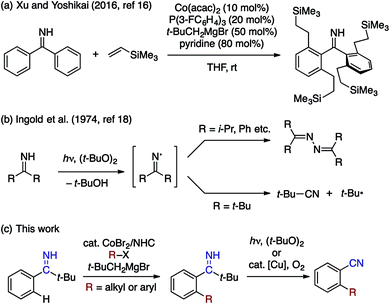 |
| Scheme 2 N–H imine as directing and transformable functional group for cobalt-catalyzed C–H activation. | |
Building on the above background, we have found that pivalophenone N–H imine serves as a benzonitrile surrogate for directed C–H functionalization reactions under cobalt catalysis,19 which is reported herein. Thus, the tert-butyl N–H imine group smoothly directs cobalt-catalyzed C–H alkylation13 and arylation20 with the corresponding organic halides, and then readily undergoes fragmentation into a cyano group under peroxide photolysis or aerobic copper catalysis (Scheme 2c). Interestingly, the fragmentation step has proved to become feasible only after the installation of the ortho-substituent, which prevents the undesirable iminyl radical dimerization. The overall process offers a complementary and convenient route to a variety of ortho-substituted aryl nitriles, especially ortho-alkylated aryl nitriles, for which no direct preparative method from the corresponding aryl nitriles exists.
Results and discussion
The feasibility of the C–H alkylation of pivalophenone N–H imine (1a) was initially explored using n-octyl bromide (2a, 1.5 equiv.) as an alkylating agent. As a result of screening experiments (Table S1†), a catalytic system comprising CoBr2 (10 mol%), N,N′-diisopropylimidazolinium tetrafluoroborate (L1·HBF4, 10 mol%), and t-BuCH2MgBr (2 equiv.) was found to promote the desired reaction at room temperature, exclusively affording the monoalkylation product 3aa in 90% yield (Table 1). As was the case with previously reported cobalt-catalyzed C–H alkylation reactions,13b–e the choice of the imidazol(in)ium salt as an N-heterocyclic carbene (NHC) precursor was crucial. The reaction using n-octyl chloride instead of 2a also afforded 3aa albeit in a modest yield.
Table 1
ortho-Alkylation of pivalophenone N–H iminesa
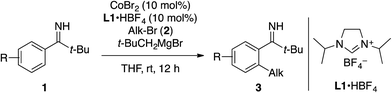
|
Reaction conditions: imine 1 (0.2 mmol), alkyl bromide 2 (1.5 equiv.), CoBr2 (10 mol%), L1·HBF4 (10 mol%), t-BuCH2MgBr (2 equiv.), THF, rt, 12 h.
The product was isolated in the form of ketone after acidic hydrolysis.
The major regioisomer is shown (r.r. = regioisomer ratio). The yield refers to the overall yield.
|
|
Table 1 summarizes the scope of the present C–H alkylation. The reaction of 1a with various primary alkyl bromides afforded the corresponding monoalkylation products 3aa–3al in good yields, tolerating alkyl chloride (3ad), alkyl fluoride (3ae), olefin moieties (3af–3ah), and bulky substituents at the α-position (3ai–3al). Note that the reaction of 6-bromo-1-hexene exclusively afforded the simple alkylation product 3ag, while the analogous reaction using acetophenone N-aryl imine was accompanied by a ring-closing alkylation (cyclopentylmethylation) product.13d Cyclic and acyclic secondary alkyl bromides also reacted with 1a to afford the corresponding products 3am–3aq in moderate to good yields.
A variety of substituted pivalophenone imines participated in the C–H alkylation using n-octyl bromide, tolerating substituents such as methoxy, trifluoromethoxy, methylthio, dimethylamino, fluoro, and trifluoromethyl groups (see 3da–3ha and 3ja). Imines bearing methyl, trifluoromethyl, or methoxy group at the meta position and 2-naphthyl imine underwent exclusive or selective alkylation at the less hindered position (see 3ia–3ka and 3ma), whereas a 3,4-methylenedioxy group directed the reaction to take place preferentially at its proximity, albeit with modest regioselectivity (see 3la). Unfortunately, ortho-substituted pivalophenone imine failed to participate in the reaction. Cyclohexylation reactions were also successfully performed using several substituted imines (see 3bn, 3fn, 3in, and 3mn). Note that, under the present conditions, the n-octylation reaction using valerophenone N–H imine instead of 1a took place rather sluggishly (<30% yield).
Next, we examined the feasibility of cobalt-catalyzed directed C–H arylation.13c,e,20 Upon screening conditions for the coupling of imine 1a with 4-chloroanisole (4a), imidazolium salt L2·HBr featuring 2,6-diethylphenyl groups and cyclohexyl backbone emerged as an effective NHC precursor among others such as commercially available IMes and IPr derivatives (see Table S2† for detail). Furthermore, the use of N,N,N′,N′-tetramethylethylenediamine (TMEDA) as an additive proved beneficial. Thus, a catalytic system comprised of these key components, Co(acac)3, and t-BuCH2MgBr promoted the arylation reaction at room temperature, affording the desired biaryl 5aa in 84% yield (Table 2). Note that the use of 4-bromoanisole instead of 4a failed to produce 5aa but resulted in the direct cross-coupling between 4-bromoanisole and t-BuCH2MgBr.
Table 2
ortho-Arylation of pivalophenone N–H iminesa
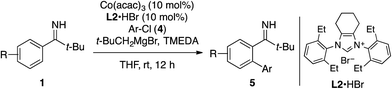
|
Reaction conditions: imine 1 (0.2 mmol), aryl chloride 4 (1.5 equiv.), CoBr2 (10 mol%), L2·HBr (10 mol%), t-BuCH2MgBr (2.5 equiv.), TMEDA (80 mol%), THF, rt, 12 h.
The major regioisomer is shown (r.r. = regioisomer ratio). The yield refers to the overall yield.
|
|
Under the optimized conditions, 1a reacted with various aryl chlorides to afford the corresponding biaryls 5ab–5at in moderate to good yields, tolerating substituents including methoxy, dimethylamino, siloxy, fluoro, and trifluoromethyl groups. A series of substituted pivalophenone imines could also be arylated with 4-chloroanisole in good yields (see 5ba–5ma). Note that the imine bearing a 3,4-methylenedioxy group underwent exclusive arylation at the proximal ortho position (see 5la), in contrast to the modest regioselectivity observed for the alkylation of the same imine (see 3la in Table 1). Also notable was the behavior of 2-naphthyl imine, which produced the arylation product 5ma as a regioisomeric mixture while undergoing exclusive alkylation at the less hindered position (see 3ma and 3mn). As was the case with the C–H alkylation, ortho-substituted pivalophenone imine was reluctant to undergo the C–H arylation.
To probe the feasibility of the imine-to-nitrile conversion, we initially subjected 1a to UV irradiation (254 nm) in di-tert-butyl peroxide. Not unexpectedly, the reaction mainly afforded azine 6via dimerization of the iminyl radical, accompanied by only a trace amount of benzonitrile (Scheme 3a). In contrast, the ortho-alkylated imine 3aa quantitatively furnished 2-alkylbenzonitrile 7a under the same conditions (Scheme 3b). The ortho-arylated imine 5aa also afforded 2-cyanobiaryl 7b, albeit in somewhat lower yield (79%) due to the formation of 6-(tert-butyl)-3-methoxyphenanthridine as a byproduct via intramolecular cyclization of the iminyl radical.21 Furthermore, alternative conditions employing catalytic Cu(OAc)2 under O2 atmosphere, originally developed by Chiba for the conversion of 2-biaryl N–H imines to phenanthridines,22 proved equally effective for the conversion of 3aa and 5aa to 7a and 7b, respectively, presumably via fragmentation of a putative iminylcopper species (Scheme 3c).
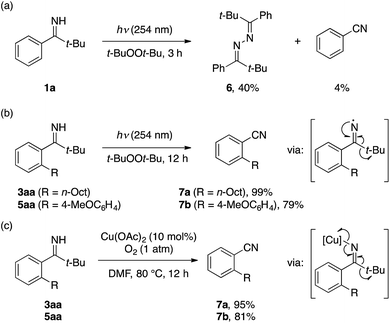 |
| Scheme 3 Decomposition reactions of pivalophenone imines. | |
With the imine-to-nitrile conversion methods in hand, we explored two-step synthesis of ortho-alkylated or -arylated benzonitriles (Table 3). Thus, alkylation or arylation reactions of pivalophenone N–H imines were followed by submission of the crude products to the peroxide photolysis (A) or the aerobic copper catalysis (B). To our satisfaction, these protocols worked particularly well for the preparation of ortho-alkylbenzonitriles 7c–7p, many of which were obtained in yields close to the yields of the corresponding C–H alkylation products (Table 1). On the other hand, the yields of the ortho-arylbenzonitriles 7q–7v (ca. 60%) were apparently lower than that of the corresponding C–H arylation products (ca. 80%; Table 2), reflecting the lower efficiency of the second step.
Table 3 Two-step synthesis of ortho-substituted benzonitrilesa
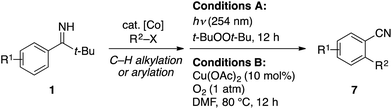
|
See the ESI for the detailed procedure. The yields refer to overall yields based on 1.
|
|
Several experiments were performed to gain mechanistic insight into the present reactions (Scheme 4). A treatment of imine 1i in [D8]-THF with excess t-BuCH2MgBr caused a near complete disappearance of the N–H proton signal and a sizable chemical shift change of the C
N carbon in the 1H and 13C NMR spectra (Fig. S1†), indicating formation of a magnesium alkylideneamide species (Scheme 4a). This suggests that the alkylideneamide anion, rather than the parent N–H imine,16,23 serves as the actual directing group for cobalt-mediated C–H activation, as is also the case for cobalt-catalyzed, secondary amide-directed C–H alkylation and arylation.13a,20c The superiority of this anionic directing group to an N-aryl imine directing group13b,20a was demonstrated for both the alkylation and arylation reactions by competition experiments (Scheme 4b). The reaction of 1a with a mixture of n-octyl bromide and 4-chloroanisole exclusively afforded the alkylation product under the Co–L1 system (Scheme 4c), which is consistent with the inability of L1 to promote the C–H arylation reaction (Table S2†). By contrast, the same reaction under the Co–L2 system produced none of the C–H functionalization products, presumably due to the interference of the C–H activation step by n-octyl bromide. The addition of TEMPO caused no apparent interference with the C–H alkylation using n-octyl bromide but substantially inhibited the reactions of cyclohexyl bromide and 4-chloroanisole (Scheme 4d). These observations suggest that, at least for the latter two cases, single electron transfer is involved in the carbon–halogen bond cleavage.13,19a–d
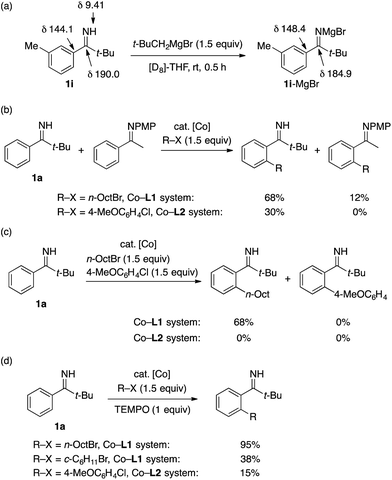 |
| Scheme 4 Mechanistic experiments (PMP = p-methoxyphenyl). The yields in Scheme 4b–d were determined by GC using n-tridecane as an internal standard. | |
Conclusions
In summary, we have demonstrated that pivalophenone N–H imine serves as an excellent substrate for the cobalt-catalyzed directed C–H alkylation and arylation reactions with alkyl bromides and aryl chlorides, respectively. Owing to the added steric bulk in the ortho position, the resulting unpurified ortho-alkylated or -arylated pivalophenone imines undergo clean fragmentation of the imine functionality to afford the corresponding ortho-functionalized aryl nitriles. The present two-step protocol would be particularly attractive for the preparation of ortho-alkylated aryl nitriles, which have not been directly accessed from the corresponding aryl nitriles via C–H activation. We anticipate that pivalophenone N–H imine could be exploited as a benzonitrile surrogate not only for cobalt catalysis but also for other transition metal-catalyzed C–H functionalization reactions under mild conditions.24 Studies in this direction are ongoing in our laboratory.
Acknowledgements
This work was supported by the Singapore Ministry of Education, Nanyang Technological University (RG 5/14, RG 114/15), and JST, CREST. We thank Dr Pin-Sheng Lee for the preparation of NHC precursors including L2·HBr.
Notes and references
- For selected reviews, see:
(a) D. A. Colby, R. G. Bergman and J. A. Ellman, Chem. Rev., 2010, 110, 624 CrossRef CAS PubMed;
(b) T. W. Lyons and M. S. Sanford, Chem. Rev., 2010, 110, 1147 CrossRef CAS PubMed;
(c) L. Ackermann, Chem. Rev., 2011, 111, 1315 CrossRef CAS PubMed;
(d) K. M. Engle, T. S. Mei, M. Wasa and J. Q. Yu, Acc. Chem. Res., 2012, 45, 788 CrossRef CAS PubMed;
(e) P. B. Arockiam, C. Bruneau and P. H. Dixneuf, Chem. Rev., 2012, 112, 5879 CrossRef CAS PubMed;
(f) D. A. Colby, A. S. Tsai, R. G. Bergman and J. A. Ellman, Acc. Chem. Res., 2012, 45, 814 CrossRef CAS PubMed;
(g) L. Ackermann, Acc. Chem. Res., 2014, 47, 281 CrossRef CAS PubMed.
- F. Kakiuchi, M. Sonoda, T. Tsujimoto, N. Chatani and S. Murai, Chem. Lett., 1999, 1083 CrossRef CAS.
-
(a) W. Li, Z. Xu, P. Sun, X. Jiang and M. Fang, Org. Lett., 2011, 13, 1286 CrossRef CAS PubMed;
(b) J.-C. Wan, J.-M. Huang, Y.-H. Jhan and J.-C. Hsieh, Org. Lett., 2013, 15, 2742 CrossRef CAS PubMed.
- W. Li and P. Sun, J. Org. Chem., 2012, 77, 8362 CrossRef CAS PubMed.
- B. Du, X. Jiang and P. Sun, J. Org. Chem., 2013, 78, 12280 CrossRef CAS.
- M. C. Reddy and M. Jeganmohan, Chem. Commun., 2015, 51, 10738 RSC.
- For the use of a cyano group as an end-on directing group for meta- or para-selective C–H activation, see:
(a) D. Leow, G. Li, T.-S. Mei and J.-Q. Yu, Nature, 2012, 486, 518 CrossRef CAS PubMed;
(b) R.-Y. Tang, G. Li and J.-Q. Yu, Nature, 2014, 507, 215 CrossRef CAS PubMed;
(c) G. Yang, P. Lindovska, D. Zhu, J. Kim, P. Wang, R.-Y. Tang, M. Movassaghi and J.-Q. Yu, J. Am. Chem. Soc., 2014, 136, 10807 CrossRef CAS PubMed;
(d) S. Bag, T. Patra, A. Modak, A. Deb, S. Maity, U. Dutta, A. Dey, R. Kancherla, A. Maji, A. Hazra, M. Bera and D. Maiti, J. Am. Chem. Soc., 2015, 137, 11888 CrossRef CAS PubMed;
(e) S. Li, L. Cai, H. Ji, L. Yang and G. Li, Nat. Commun., 2016, 7, 10443 CrossRef PubMed;
(f) T. Patra, S. Bag, R. Kancherla, A. Mondal, A. Dey, S. Pimparkar, S. Agasti, A. Modak and D. Maiti, Angew. Chem., Int. Ed., 2016, 55, 7751 CrossRef CAS PubMed.
- X. Yang, X. Jin and C. Wang, Adv. Synth. Catal., 2016, 358, 2436 CrossRef CAS.
- For selected reviews, see:
(a) D. Alberico, M. E. Scott and M. Lautens, Chem. Rev., 2007, 107, 174 CrossRef CAS PubMed;
(b) L. Ackermann, R. Vicente and A. R. Kapdi, Angew. Chem., Int. Ed., 2009, 48, 9792 CrossRef CAS PubMed;
(c) J. Le Bras and J. Muzart, Chem. Rev., 2011, 111, 1170 CrossRef CAS PubMed;
(d) C. S. Yeung and V. M. Dong, Chem. Rev., 2011, 111, 1215 CrossRef CAS PubMed;
(e) C. Liu, J. Yuan, M. Gao, S. Tang, W. Li, R. Shi and A. Lei, Chem. Rev., 2015, 115, 12138 CrossRef CAS PubMed;
(f) Y. Segawa, T. Maekawa and K. Itami, Angew. Chem., Int. Ed., 2015, 54, 66 CrossRef CAS PubMed.
-
(a) Y.-H. Zhang, B.-F. Shi and J.-Q. Yu, Angew. Chem., Int. Ed., 2009, 48, 6097 CrossRef CAS PubMed;
(b) D. Shabashov and O. Daugulis, J. Am. Chem. Soc., 2010, 132, 3965 CrossRef CAS PubMed;
(c) Y. Zhao and G. Chen, Org. Lett., 2011, 13, 4850 CrossRef CAS PubMed;
(d) E. T. Nadres, G. I. F. Santos, D. Shabashov and O. Daugulis, J. Org. Chem., 2013, 78, 9689 CrossRef CAS PubMed;
(e) B. Xiao, Z.-J. Liu, L. Liu and Y. Fu, J. Am. Chem. Soc., 2013, 135, 616 CrossRef CAS PubMed;
(f) R.-Y. Zhu, J. He, X.-C. Wang and J.-Q. Yu, J. Am. Chem. Soc., 2014, 136, 13194 CrossRef CAS PubMed;
(g) S.-Y. Zhang, Q. Li, G. He, W. A. Nack and G. Chen, J. Am. Chem. Soc., 2015, 137, 531 CrossRef CAS PubMed.
-
(a) L. Ackermann, P. Novak, R. Vicente and N. Hofmann, Angew. Chem., Int. Ed., 2009, 48, 6045 CrossRef CAS PubMed;
(b) L. Ackermann, N. Hofmann and R. Vicente, Org. Lett., 2011, 13, 1875 CrossRef CAS PubMed.
-
(a) Y. Aihara and N. Chatani, J. Am. Chem. Soc., 2013, 135, 5308 CrossRef CAS PubMed;
(b) W. Song, S. Lackner and L. Ackermann, Angew. Chem., Int. Ed., 2014, 53, 2477 CrossRef CAS PubMed;
(c) Z. Ruan, S. Lackner and L. Ackermann, Angew. Chem., Int. Ed., 2016, 55, 3153 CrossRef CAS PubMed.
-
(a) Q. Chen, L. Ilies and E. Nakamura, J. Am. Chem. Soc., 2011, 133, 428 CrossRef CAS PubMed;
(b) K. Gao and N. Yoshikai, J. Am. Chem. Soc., 2013, 135, 9279 CrossRef CAS PubMed;
(c) B. Punji, W. Song, G. A. Shevchenko and L. Ackermann, Chem.–Eur. J., 2013, 19, 10605 CrossRef CAS PubMed;
(d) K. Gao, T. Yamakawa and N. Yoshikai, Synthesis, 2014, 46, 2024 CrossRef CAS;
(e) R. Mei and L. Ackermann, Adv. Synth. Catal., 2016, 358, 2443 CrossRef CAS.
-
(a) E. R. Fruchey, B. M. Monks and S. P. Cook, J. Am. Chem. Soc., 2014, 136, 13130 CrossRef CAS PubMed;
(b) L. Ilies, T. Matsubara, S. Ichikawa, S. Asako and E. Nakamura, J. Am. Chem. Soc., 2014, 136, 13126 CrossRef CAS PubMed;
(c) B. M. Monks, E. R. Fruchey and S. P. Cook, Angew. Chem., Int. Ed., 2014, 53, 11065 CrossRef CAS PubMed;
(d) G. Cera, T. Haven and L. Ackermann, Angew. Chem., Int. Ed., 2016, 55, 1484 CrossRef CAS PubMed.
- For a recent review on C–H alkylation using olefins as alkylating agents, see: Z. Dong, Z. Ren, S. J. Thompson and G. Dong, Chem. Rev., 2017 DOI:10.1021/acs.chemrev.6b00574.
- W. Xu and N. Yoshikai, Angew. Chem., Int. Ed., 2016, 55, 12731 CrossRef CAS PubMed.
- For N–H imine-directed C–H activation/annulation reactions, see:
(a) T. Fukutani, N. Umeda, K. Hirano, T. Satoh and M. Miura, Chem. Commun., 2009, 5141 RSC;
(b) Z.-M. Sun, S.-P. Chen and P. Zhao, Chem.–Eur. J., 2010, 16, 2619 CrossRef CAS PubMed;
(c) D. N. Tran and N. Cramer, Angew. Chem., Int. Ed., 2010, 49, 8181 CrossRef CAS PubMed;
(d) D. N. Tran and N. Cramer, Angew. Chem., Int. Ed., 2011, 50, 11098 CrossRef CAS PubMed;
(e) D. N. Tran and N. Cramer, Angew. Chem., Int. Ed., 2013, 52, 10630 CrossRef CAS PubMed;
(f) J. Zhang, A. Ugrinov and P. Zhao, Angew. Chem., Int. Ed., 2013, 52, 6681 CrossRef CAS PubMed;
(g) R. He, Z.-T. Huang, Q.-Y. Zheng and C. Wang, Angew. Chem., Int. Ed., 2014, 53, 4950 CrossRef CAS PubMed;
(h) T. Jia, C. Zhao, R. He, H. Chen and C. Wang, Angew. Chem., Int. Ed., 2016, 55, 5268 CrossRef CAS PubMed;
(i) J. H. Kim, S. Gressies and F. Glorius, Angew. Chem., Int. Ed., 2016, 55, 5577 CrossRef CAS PubMed;
(j) X. Jin, X. Yang, Y. Yang and C. Wang, Org. Chem. Front., 2016, 3, 268 RSC;
(k) S. Gupta, J. Han, Y. Kim, S. W. Lee, Y. H. Rhee and J. Park, J. Org. Chem., 2014, 79, 9094 CrossRef CAS PubMed.
- D. Griller, G. d. Mendenha, W. Vanhoof and K. U. Ingold, J. Am. Chem. Soc., 1974, 96, 6068 CrossRef CAS.
-
(a) L. Ackermann, J. Org. Chem., 2014, 79, 8948 CrossRef CAS PubMed;
(b) K. Gao and N. Yoshikai, Acc. Chem. Res., 2014, 47, 1208 CrossRef CAS PubMed;
(c) N. Yoshikai, Bull. Chem. Soc. Jpn., 2014, 87, 843 CrossRef CAS;
(d) M. Moselage, J. Li and L. Ackermann, ACS Catal., 2016, 6, 498 CrossRef CAS;
(e) P. Gandeepan and C.-H. Cheng, Acc. Chem. Res., 2015, 48, 1194 CrossRef CAS PubMed;
(f) D. Wei, X. Zhu, J.-L. Niu and M.-P. Song, ChemCatChem, 2016, 8, 1242 CrossRef CAS.
-
(a) K. Gao, P.-S. Lee, C. Long and N. Yoshikai, Org. Lett., 2012, 14, 4234 CrossRef CAS PubMed;
(b) W. Song and L. Ackermann, Angew. Chem., Int. Ed., 2012, 51, 8251 CrossRef CAS PubMed;
(c) J. Li and L. Ackermann, Chem.–Eur. J., 2015, 21, 5718 CrossRef CAS PubMed.
- R. T. McBurney, A. M. Z. Slawin, L. A. Smart, Y. Yu and J. C. Walton, Chem. Commun., 2011, 47, 7974 RSC.
- L. Zhang, G. Y. Ang and S. Chiba, Org. Lett., 2010, 12, 3682 CrossRef CAS PubMed.
- H.-F. Klein, S. Camadanli, R. Beck, D. Leukel and U. Flörke, Angew. Chem., Int. Ed., 2005, 44, 975 CrossRef CAS PubMed.
-
(a) J. Wencel-Delord, T. Droge, F. Liu and F. Glorius, Chem. Soc. Rev., 2011, 40, 4740 RSC;
(b) T. Gensch, M. N. Hopkinson, F. Glorius and J. Wencel-Delord, Chem. Soc. Rev., 2016, 45, 2900 RSC.
Footnote |
† Electronic supplementary information (ESI) available. See DOI: 10.1039/c7sc01732d |
|
This journal is © The Royal Society of Chemistry 2017 |
Click here to see how this site uses Cookies. View our privacy policy here.