DOI:
10.1039/C7SC02249B
(Edge Article)
Chem. Sci., 2017,
8, 5962-5968
Borylation of fluorinated arenes using the boron-centred nucleophile B(CN)32− – a unique entry to aryltricyanoborates†
Received
18th May 2017
, Accepted 24th June 2017
First published on 26th June 2017
Abstract
The potassium salt of the boron-centred nucleophile B(CN)32− (1) readily reacts with perfluorinated arenes, such as hexafluorobenzene, decafluorobiphenyl, octafluoronaphthalene and pentafluoropyridine, which results in KF and the K+ salts of the respective borate anions with one {B(CN)3} unit bonded to the (hetero)arene. An excess of K21 leads to the successive reaction of two or, in the case of perfluoropyridine, even three C–F moieties and the formation of di- and trianions, respectively. Moreover, all of the 11 partially fluorinated benzene derivatives, C6F6−nHn (n = 1–5), generally react with K21 to give new tricyano(phenyl)borate anions with high chemo- and regioselectivity. A decreasing number of fluorine substituents on benzene results in a decrease in the reaction rate. In the cases of partially fluorinated benzenes, the addition of LiCl is advantageous or even necessary to facilitate the reaction. Also, pentafluorobenzenes R–C6F5 (R = –CN, –OMe, –Me, or –CF3) react via C–F/C–B exchange that mostly occurs in the para position and to a lesser extent in the meta or ortho positions. Most of the reactions proceed via an SNAr mechanism. The reaction of 1,4-F2C6H4 with K21 shows that an aryne mechanism has to be considered in some cases as well. In summary, a wealth of new stable tricyano(aryl)borates have been synthesised and fully characterized using multi-NMR spectroscopy and most of them were characterised using single-crystal X-ray diffraction.
Introduction
Anionic boron-centred nucleophiles are of growing interest as boron centres are usually electrophilic (Lewis-acidic). Therefore, boron-centred nucleophiles have unusual reactivity and are expected to have large synthetic potential.1–4 The isolation of the first boron-centred nucleophile in 2006, the lithiated boryl anion A that is stable in THF at −45 °C for months, was an important development in this field that stimulated intensive further research (Scheme 1).5 The chemistry of the lithiated boryl anion A and closely related species3,6–8 was studied in detail, and these compounds were found to be versatile starting compounds for the introduction of boryl moieties.1,3–14 Although some other boron-centred nucleophiles have been described, there is still a limited number of them.15–26 Boryl anions were found to be stabilized by carbene ligands such as NHCs (NHC = N-heterocyclic carbene) and cAACs (cAAC = cyclic (alkyl)(amino)carbene), as demonstrated in B27 and C28 (Scheme 1). An alternative approach for the stabilization of boron-centred nucleophiles is the introduction of cyano groups at the boron atom, as demonstrated in C28 and the tricyanoborate dianion B(CN)32− (1).29–32 The latter is the only dianionic boryl anion known to date, and its alkali metal salts are indefinitely stable at room temperature under an inert atmosphere. The first syntheses towards the alkali metal salts of 1 started from M[B(CN)4] (M = alkali metal).29 Recently, we reported convenient, high-yield and large-scale entries towards the salts of 1 starting from the readily available tricyanoborates M[BF(CN)3]33,34 and M[BH(CN)3]35,36 (Scheme 1).30–32 The unprecedented formation of dianion 1 from [BH(CN)3]− is the first example of the deprotonation of a hydridoborate anion.31,32 The conversion of a hydridoborane into monoanion C is the only related reaction28 known to date.
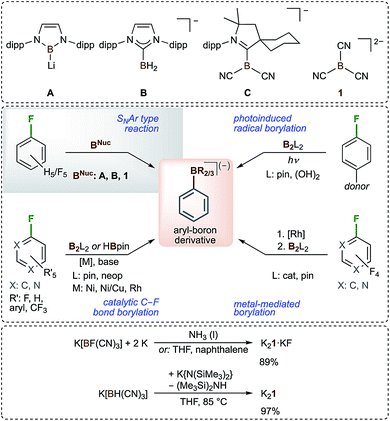 |
| Scheme 1 Selected boron-centred nucleophiles (top), the different types of conversion of C–F into C–B moieties of arenes and heteroarenes (middle), and convenient syntheses of K21 (bottom); (dipp = 2,6-diisopropylphenyl, cat = catecholato, pin = pinacolato, and neop = neopentyl glycolato). | |
As mentioned previously, boron-centred nucleophiles have large synthetic potential. Surprisingly, only very few examples of reactions of such nucleophiles with fluorinated arenes have been reported. Boryl lithium A was found to react with C6F6 or C6H5F to obtain the corresponding monoborylated benzenes.6 Similar reactions have been reported for B27 and 130 with C6F6 only (Scheme 1). In general, only a limited number of transformations of C–F into C–B bonds of arenes or heteroarenes are known. They are either metal-catalyzed37–42 or metal-mediated43 reactions, or photoinduced radical borylations44,45 (Scheme 1).
Herein, we report on the SNAr reactions of the boryl dianion B(CN)32− (1) with selected fully and partially fluorinated arenes46–51 including perfluorinated pyridine, naphthalene and biphenyl as well as fluorinated arenes with a functional group. All of the reactions proceeded via the exchange of fluorine with the tricyanoboryl moiety and most of them were chemo- and regioselective. Even multiple exchange reactions that provide access to bis- and tris(tricyanoborate) anions have been achieved.
Results and discussion
Reactions of K2B(CN)3 (K21) with perfluoropyridine
As outlined in the introduction, K2B(CN)3 (K21) was found to react with an excess of C6F6 at room temperature in THF to give the salts of the [C6F5B(CN)3]− anion (B6a) in 67% yield.30 A related reaction of perfluoropyridine with K21 was found to start at temperatures below 0 °C, as evidenced by the rapid decolorization of the suspension. The [4-{(NC)3B}-C5F4N]− anion (Py1) was obtained as the main product of the reaction with an excess of perfluoropyridine (Table 1 and Fig. 1). The exchange of the fluorine in the 4-position is typical for the SNAr reactions of C5F5N.52–55 The dianion [2,4-{(NC)3B}2-C5F3N]2− (Py2), a different isomer of Py1, most likely [2-{(NC)3B}-C5F4N]−, and small amounts (<1%) of further tricyano(fluoropyridinyl)borate anions, including the trianion [2,4,6-{(NC)3B}3-C5F3N]3− (Py3), were obtained as the side products.
Table 1 Reactions of K21 with selected fluoro(hetero)arenes
Entry |
Substrate |
LiCla |
K21b |
Conditions |
[BH(CN)3]−c |
Major tricyanoborate anion(s) formed |
Isolated yield |
Whether LiCl was added to the reaction mixture.
Equivalents of K21.
The percentage that was formed as a side product; [BH(CN)3]− was removed during the work-up.
The ratio B2c : B2b was 6 : 4 in the reaction mixture and 7 : 3 in the isolated material.
11% of K[BH(CN)3].
[Et3NH]+ salt.
The internal yield was 75% Py1, 16% Py2, 8% another isomer (probably [2-{(NC)3B}-C5F4N]−), and 1% Py3 and unknown tricyano(fluoropyridinyl)borate anions.
Purity ca. 85% (11B/19F NMR); it contained 15% other tricyano(fluoropyridinyl)borates.
The internal yield (11B/19F NMR) was 60% Py2, 30% Py3, and 10% another tricyano(fluoropyridinyl)borate anion.
Purity ca. 75% (11B/19F NMR); it contained 15% K3Py3 and 10% another tricyano(fluoropyridinyl)borate.
N2 : N3 = 1.0 : 0.8 (N3 = [2,7-{(NC)3B}2-C10F6]2−).
The ratio of the reaction mixture: N1 : N2 : N3 = 0.4 : 1.0 : 0.7; N2 is hardly soluble and was obtained as a pure K+ salt.
K[B(CN)4] was mostly removed via fractional precipitation.
|
1 |
C6FH5 |
Yes |
<1 |
80 °C, 2 d |
25% |
[1-{(NC)3B}-C6H5]− (B1) |
Sole isomer |
45% |
2 |
1,2-C6F2H4 |
Yes |
<1 |
r.t., 16 h |
9% |
[1-{(NC)3B}-2-F-C6H4]− (B2a) |
Sole isomer |
58% |
3 |
1,3-C6F2H4 |
Yes |
<1 |
r.t., 3 d |
<5% |
[1-{(NC)3B}-3-F-C6H4]− (B2b) |
Sole isomer |
70% |
4 |
1,4-C6F2H4 |
Yes |
<1 |
75 °C, 30 h |
28% |
[1-{(NC)3B}-4-F-C6H4]− (B2c) + B2b (6 : 4d)e |
45%e |
5 |
1,2,3-C6F3H3 |
Yes |
<1 |
r.t., 3 d |
<5% |
[1-{(NC)3B}-2,3-F2-C6H3]− (B3a) + [1-{(NC)3B}-2,6-F2-C6H3]− (B3b) (4 : 1) |
66% |
6 |
1,2,4-C6F3H3 |
Yes |
<1 |
r.t., 2 h |
<5% |
[1-{(NC)3B}-2,5-F2-C6H3]− (B3c) |
Sole isomer |
76% |
7 |
1,3,5-C6F3H3 |
Yes |
<1 |
r.t., 16 h |
<5% |
[1-{(NC)3B}-3,5-F2-C6H3]− (B3d) |
Sole isomer |
63% |
8 |
1,2,3,4-C6F4H2 |
Yes |
<1 |
r.t., <1 h |
<5% |
[1-{(NC)3B}-2,3,6-F3-C6H2]− (B4a) |
Sole isomer |
77% |
9 |
1,2,3,5-C6F4H2 |
Yes |
<1 |
r.t., 2 h |
<5% |
[1-{(NC)3B}-2,3,5-F3-C6H2]− (B4b) |
Sole isomer |
63% |
10 |
1,2,3,5-C6F4H2 |
No |
<1 |
75 °C, 30 h |
10% |
B4b
|
8% of other isomers |
51% |
11 |
1,2,4,5-C6F4H2 |
Yes |
<1 |
r.t., 30 min |
30% |
[1-{(NC)3B}-2,4,5-F3-C6H2]− (B4c) |
Sole isomer |
50% |
12 |
1,2,4,5-C6F4H2 |
Yes |
2 |
r.t., 16 h |
n.d. |
[1,4-{(NC)3B}2-2,5-F2-C6H2]2− (B4d) |
Sole isomer |
42%f |
13 |
C6F5H |
Yes |
<1 |
r.t., 10 min |
10% |
[1-{(NC)3B}-2,3,5,6-F4-C6H]− (B5) |
6% of one other isomer |
62% |
14 |
C6F5H |
No |
<1 |
r.t., 2 d |
48% |
B5
|
20% of other anions |
39% |
15 |
C6F6 |
No |
<1 |
0 °C |
— |
[1-{(NC)3B}-C6F5]− (B6a)29 |
Sole isomer |
67% (ref. 30) |
16 |
C6F6 |
No |
2.2 |
Reflux, 20 h |
— |
[1,4-{(NC)3B}2-C6F4]2− (B6b) |
Sole isomer |
74% |
17 |
C5F5N |
No |
0.33 |
r.t., 12 h |
— |
[4-{(NC)3B}-C5F4N]− (Py1)g |
Other isomer(s), Py2, Py3 |
81%h |
18 |
C5F5N |
No |
2 |
r.t., 4 d |
— |
[2,4-{(NC)3B}2-C5F3N]2− (Py2)i |
Other isomer(s), Py3 |
59%j |
19 |
C5F5N |
No |
3 |
r.t., 4 d |
— |
[2,4,6-{(NC)3B}3-C5F2N]3− (Py3) |
<10% Py1 and Py2 |
31% |
20 |
C12F10 |
No |
0.8 |
r.t., 2 h |
— |
[4-{(NC)3B}-C12F9]− (BP1) |
<20% of BP2 |
49% |
21 |
C12F10 |
No |
2.2 |
50 °C, 1 h |
— |
[4,4′-{(NC)3B}2-C12F8]2− (BP2) |
Sole isomer |
90% |
22 |
C10F8 |
No |
0.5 |
r.t., 3 d |
— |
[2-{(NC)3B}-C10F7]− (N1) |
8% of other isomersk |
55% |
23 |
C10F8 |
No |
2 |
60 °C, 16 h |
— |
[2,6-{(NC)3B}2-C10F6]2− (N2) |
Mixture of N1, N2 and N3l |
24% |
24 |
F3C–C6F5 |
No |
<1 |
r.t., 3 h |
— |
[1-F3C-4-{(NC)3B}-C6F4]− (B7) |
Sole isomer |
68% |
25 |
Me–C6F5 |
No |
<1 |
90 °C, 3 d |
— |
[1-Me-4-{(NC)3B}-C6F4]− (B8a) + [1-Me-3-{(NC)3B}-C6F4]− (B8b) (9 : 1) |
n.d. |
26 |
1-F3C-4-H-C6F4 |
No |
<1 |
r.t., 2 h |
75% |
Unidentified borate anions |
n.d. |
27 |
1-F3C-6-H-C6F4 |
No |
<1 |
r.t., 4 d |
2% |
[1-F3C-4-{(NC)3B}-2,3,5-F3-C6H]− (B9) |
7% of other isomers |
78% |
28 |
MeO–C6F5 |
No |
<1 |
60 °C, 16 h |
— |
[1-MeO-4-{(NC)3B}-C6F4]− (B10a) + [1-MeO-3-{(NC)3B}-C6F4]− (B10b) (1 : 1) |
82% |
29 |
NC-C6F5 |
No |
<1 |
r.t., <1 h |
— |
[1-NC-4-{(NC)3B}-C6F4]− (B11a) + [1-NC-2-{(NC)3B}-C6F4]− (B11b) + [B(CN)4]− (2.5 : 1.5 : 1)m |
n.d. |
30 |
Cl–C6F5 |
No |
<1 |
r.t., <1 h |
— |
[BCl(CN)3]−, [B2(CN)6]2−, … |
n.d. |
31 |
O2N–C6F5 |
No |
<1 |
r.t., 16 h |
— |
[B2(CN)6]2−, … |
n.d. |
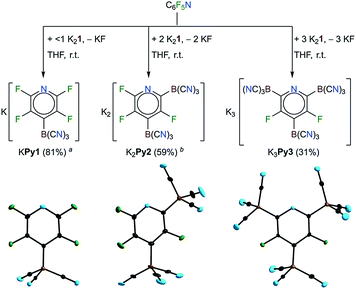 |
| Fig. 1 Top: the reactions of K21 with pentafluoropyridine to give Py1, Py2 and Py3 (acontained 15% other tricyano(fluoropyridinyl)borates; bcontained 15% K3Py3 and 10% another tricyano(fluoropyridinyl)borate). Bottom: the anions in crystals of their K+ salts (the displacement ellipsoids are at the 25% probability level, except for Py3 where they are at 50%). | |
An excess of K21 yielded larger amounts of the dianion Py2 and the trianion Py3 and the reaction of perfluoropyridine with two equivalents of K21 gave K2Py2 as the major product. Three equivalents of the potassium salt resulted in K3Py3 in 31% yield. A related, successive replacement of 1, 2 and 3 fluorine substituents of perfluoropyridine via nucleophilic replacement has been previously reported for the methoxy anion.54 In contrast, alkyl and aryl Grignard reagents were reported to result in only mono-substitutions in the para position of perfluoropyridine.55 The decreasing solubility of the potassium borates KPy1, K2Py2 and K3Py3 with the increasing charge of the anion enabled the enrichment of K2Py2 and the purification of K3Py3 by precipitation from the THF solutions via the slow addition of CH2Cl2. Pure KPy1 and K2Py2 were obtained via crystallization. Single-crystals of KPy1, K2Py2·OC(CH3)2 and K3Py3·3THF·1.04 H2O were studied using X-ray diffraction (Fig. 1). Selected experimental bond distances of the three related anions Py1, Py2 and Py3 were compared to the calculated bond lengths in Table S3.†
Monosubstitution of partly fluorinated benzenes with K21
In addition to the reactions of K21 with hexafluorobenzene30 and pentafluoropyridine, K21 was found to undergo C–F/C–B exchange reactions with all of the partly fluorinated benzenes C6F6−nHn (n = 1–5) (Table 1 and Fig. 2). Unprecedentedly, the nucleophilic attack of 1 at almost all of the partially fluorinated benzenes was regio- and chemoselective. As expected for electron deficient partly fluorinated benzenes, deprotonation was observed for the strong base 1, yielding [BH(CN)3]− as a side product. The amount of [BH(CN)3]− formed reflects the Brønsted acidity of the hydrogen atoms of the respective fluorobenzene.56,57 1,2,4,5-tetrafluoro- and pentafluorobenzene gave the largest amounts of [BH(CN)3]− with up to 48% for C6F5H (Table 1). The addition of LiCl at the start of the reaction of K21 with C6F5H resulted in a reduction in the amount of [BH(CN)3]− to 10% and an increased yield of [1-{(NC)3B}-2,3,5,6-F4-C6H]− (B5) of 62%. Since the salts [(alkyl)3NH][BH(CN)3] (alkyl = Me, Et) are water soluble, purification was achieved via the precipitation or extraction of the respective trimethyl- or triethylammonium phenylborates from aqueous solutions. The ammonium salts were easily back-converted into K+ salts with K2CO3.
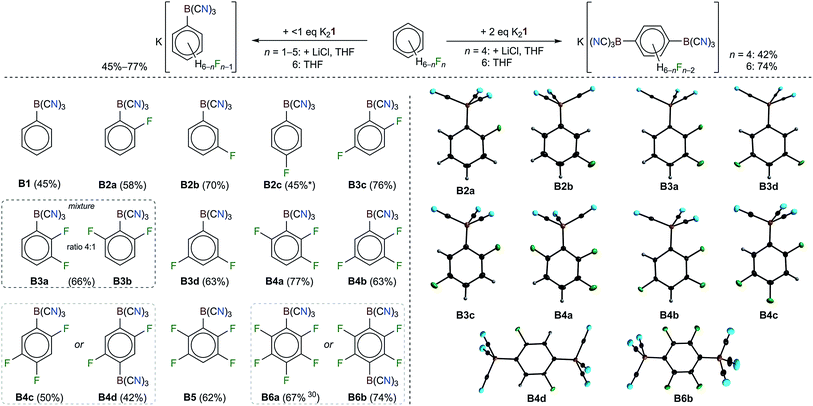 |
| Fig. 2 Reactions of K21 with fluorinated benzenes (top). The main products; dashed boxes show the anions that were derived from the same arene. In the cases of C6F6 and 1,2,4,5-C6F4H2, the different anions were obtained from different entries (bottom left). The tricyanophenylborate anions in the crystal structures of their K+ (B2a, B2b, B3a, B3c, B3d, B4a, and B4b), [Et4N]+ (B6b), [Et3NH]+ (B4d) and [Me3NH]+ (B4c) salts (the displacement ellipsoids are at the 50% probability level, except for B3a where they are at 25%, and the H atoms are depicted with arbitrary radii) (bottom right). | |
The regioselectivities of the C–F/C–B exchange reactions were found to be high, and in most cases one major isomer had formed (Table 1). Most of the new tricyanoborates were characterized using single-crystal X-ray diffraction (Fig. 2) and the details of the experimental and calculated bond parameters are summarized in Table S3.† The exchange of a fluorine with a tricyanoboryl group in the para position to a fluorine substituent is unfavoured. Replacement was found to be preferred for fluorine substituents in the meta position to one or two further fluorine substituents, which is typical for the SNAr reactions of fluorobenzenes.52,53 1,4-difluorobenzene gave a mixture of [1-{(NC)3B}-4-F-C6H4]− (B2c) and [1-{(NC)3B}-3-F-C6H4]− (B2b) in conjunction with 25% of [BH(CN)3]− (Scheme 2). The formation of anion B2b is rationalized by an aryne mechanism that is similar to related reactions.58 In summary, the high regioselectivities that were observed show that an SNAr mechanism dominates for the C–F/C–B exchange presented herein.
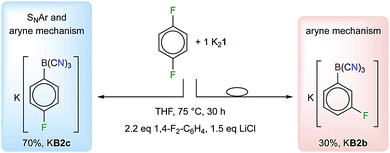 |
| Scheme 2 Reaction of 1,4-F2-C6H4 with K21 in the presence of LiCl to give a mixture of KB2 and KB3 (ratio 7 : 3). The [BH(CN)3]− that formed (25%) is not shown. | |
The reaction rate of the C–F/C–B exchange strongly depended on the degree of fluorination and therefore on the electron density of the aromatic ring system. C6F6 reacted within minutes at room temperature, whereas the conversion of C6F5H required two days. In the cases of mono-, di- and trifluorobenzenes, no reaction was observed with K21 in THF even at 80 °C. Tetrafluorobenzenes showed some reactivity towards K21 in THF depending on the substitution scheme. 1,2,3,5-tetrafluorobenzene reacted at 75 °C within 3 days. The reaction of the 1,2,4,5-isomer required 120 °C, yielding an inseparable brownish mixture. The addition of anhydrous LiCl was found to result in a tremendous increase in the reaction rate. For example, upon the addition of LiCl, a mixture of 1,2,4,5-tetrafluorobenzene and K21 immediately became warm and the reaction was complete within minutes. Similarly, the reaction time of the conversion of C6F5H into B5 was reduced from 2 days to 10 minutes. The shorter reaction time was accompanied by enhanced chemo- and regioselectivity (Table 1). The LiCl-induced reaction was successfully applied for all of the di- and trifluorobenzenes and C6FH5 (Fig. 1). The reactions of all three trifluorobenzene isomers and of 1,2- and 1,3-difluorobenzene were conducted at room temperature. Only for 1,4-F2-C6H4 and C6FH5 were higher temperatures necessary. Three different effects may be responsible for the faster reactions and the higher chemo- and regioselectivities in the presence of LiCl: (i) Li21 is more soluble in THF than K21,31 which results in an enhanced availability of the dianion 1; (ii) the high fluoride ion affinity of Li+ may lead to a Li⋯F interaction, a weakening of the C–F bond and a lowering of the activation barrier for the nucleophilic replacement; and (iii) a weak Li⋯B or Li⋯N interaction between Li+ and the boryl dianion 1 may influence the reactivity of 1.
Disubstitution of fluorinated benzenes with K21
C6F6 and 1,2,4,5-F4-C6H2 were reacted with an excess of K21 to give salts of the dianions [1,4-{(NC)3B}2-C6F4]2− (B6b) and [1,4-{(NC)3B}2-2,6-F2-C6H2]2− (B4d) (Table 1 and Fig. 2). In the case of the reaction of 1,2,4,5-F4-C6H2, anhydrous LiCl was added to enhance the rate of the reaction. Both of the reactions were found to be highly regioselective and in accordance with an SNAr mechanism. Therefore, the second C–F/C–B exchange gave the para-{B(CN)3}2 derivatives as the sole isomers.
In addition to the multiple C–F/C–B exchange reactions of C5F5N (Fig. 1), C6F6 and 1,2,4,5-F4-C6H2 (Fig. 2), perfluorobiphenyl and perfluoronaphthalene were successfully applied in related reactions with K21. Stoichiometric amounts or a slight excess of K21 yielded K2[4,4′-{(NC)3B}2-C12F8] (K2BP2) and K2[2,6-{(NC)3B}2-C10F6] (K2N2), respectively. In contrast, an excess of the perfluorinated arene predominantly gave the corresponding monoanions [4-{(NC)3B}-C12F9]− (BP1) and [2-{(NC)3B}-C10F7]− (N1) (Table 1). The successive reactions of perfluorobiphenyl that gave BP1 and BP2 were fully regioselective. K2BP2 was isolated in an excellent yield of 90% and a single crystal was investigated using X-ray diffraction (Fig. 3 and Table S3†). A slightly lower but still high regioselectivity was found for the first replacement of fluorine with 1 in perfluoronaphthalene to give N1. The introduction of a second {B(CN)3} moiety resulted in a mixture of N1, N2 and [2,7-{(NC)3B}2-C10F6]2− (N3) with the ratio 0.4
:
1.0
:
0.7. The formation of N2 as the major isomer agrees with the typical substitution scheme for the SNAr reactions of perfluoronaphthalene.53
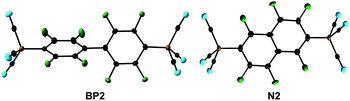 |
| Fig. 3 The bis(tricyanoborate) dianions BP2 and N2 in the crystal structures of their K+ salts (the displacement ellipsoids are at the 50% probability level). | |
Reactions of K21 with fluorinated benzenes with functional groups
The reactions described so far (vide supra) demonstrate the regioselectivity of the SNAr reaction of fluorinated (hetero)arenes with K21 and show the possibility of synthesising multiple charged tricyanoborate anions. The multiple C–F/C–B exchange reactions are the first examples of the transformations of functionalized fluorinated (hetero)arenes with 1. A series of further selected reactions of polyfluorinated benzenes with a functional group bonded to the benzene ring have been studied (Fig. 4 and Table 1, entries 24–31).
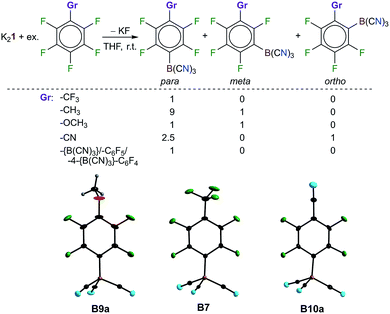 |
| Fig. 4 Reactions of K21 with functionalized fluorinated benzenes. Borate anions B9a, B7 and B10a in the crystal structures of their K+ salts (the displacement ellipsoids are at the 25% probability level, except for B10a where they are at 50%, and the H atoms are depicted with arbitrary radii). | |
Perfluorotoluene was found to give [1-F3C-4-{(NC)3B}-C6F4]− (B7) as the sole isomer and KB7 was isolated in 68% yield. 4-Me-C6F5 resulted in a mixture of the isomers [1-Me-4-{(NC)3B}-C6F4]− (B8a) and [1-Me-3-{(NC)3B}-C6F4]− (B8b) in a 9
:
1 ratio and pentafluoroanisole gave [1-MeO-4-{(NC)3B}-C6F4]− (B10a) and [1-MeO-3-{(NC)3B}-C6F4]− (B10b) in equal amounts. The decrease in the regioselectivity in the order F3C–C6F5, Me–C6F5 and MeO–C6F5 reflects the influence of the electronic properties of the –CF3, –Me and –OMe substituents on the reactivity of the corresponding fluoroarenes. The trifluoromethyl group is a strong electron withdrawing group, while the methoxy group is an electron donating group. Strong electron withdrawing groups are usually para and ortho directing. It is most likely that steric effects are the reason that the ortho-substituted product [1-F3C-2-{(NC)3B}-C6F4]− was not observed. The reaction of K21 with NC-C6F5, which contains the strong electron withdrawing cyano group that is sterically less demanding than the CF3 group, gave the ortho-substituted anion [1-NC-2-{(NC)3B}-C6F4]− (B11b) together with [1-NC-4-{(NC)3B}-C6F4]− (B11a) and [B(CN)4]− in a ratio of 1.5
:
2.5
:
1. The formation of the tetracyanoborate anion is due to the nucleophilic attack at the carbon atom of the cyano group. The formation of [B(CN)4]− starting from K21 was reported previously, e.g. from reactions with (CN)2 and PhOCN.31 Single crystals of the potassium salts of [1-F3C-4-{(NC)3B}-C6F4]− (B7), [1-MeO-4-{(NC)3B}-C6F4]− (B10a) and [1-NC-4-{(NC)3B}-C6F4]− (B11a) were characterized using diffraction experiments (Fig. 4 and Table S3†).
The deprotonation of 1-F3C-4-H-C6F4 to give [BH(CN)3]− was found to be the most relevant reaction with K21, and other unidentified borate anions had formed. In contrast, 1-F3C-2-H-C6F4 and K21 gave the [1-F3C-4-{(NC)3B}-2,3,5-F3-C6H]− anion (B9) in 78% yield and only 2% of [BH(CN)3]− was observed (Table 1).
The reactions of K21 with Cl–C6F5 and O2N–C6F5 gave complex product mixtures, and the hexacyanoborate dianion [B2(CN)6]2− (ref. 59) was identified. Additionally, the tricyanohaloborate anion [BCl(CN)3]− (ref. 60) formed from Cl–C6F5 (Table 1).
Conclusions
Boron-centred nucleophiles are an emerging class of compounds and are of growing importance for synthetic chemistry because they provide a very convenient entry to boron-functionalized compounds and materials.1–28 The unprecedented, straightforward, regioselective and transition-metal-free introduction of one, two or even three {B(CN)3}− units into fluoro(hetero)arenes starting from readily accessible K2130–32 opens unique and convenient access to single and multiple charged anions with tricyanoborate moieties. The observed regioselectivities for the C–F/C–B exchange reactions are almost all in accordance with an SNAr mechanism, which showed the boron-centred nucleophilic character of the dianion 1, as previously observed for the formation of [B2(CN)6]2− starting from K21 and K[BF(CN)3].59 So, an alternative radical reaction pathway is highly unlikely, although this type of reactivity was observed for other boron-centred nucleophiles.61,62 Furthermore, the syntheses highlight the value of K21 for the general preparation of a wealth of tricyanoborate anions. Since the salts of the tricyanoborate anions such as the ones described herein are promising building blocks for materials science, for example as components of ionic liquids that are used in electrochemical devices,63 convenient and high yield syntheses are necessary to facilitate applications.
Acknowledgements
The authors are grateful to Merck KGaA (Darmstadt, Germany) for their generous support.
Notes and references
-
M. Yamashita and K. Nozaki, in Topics in Organometallic Chemistry: Synthesis and Application of Organoboron Compounds, ed. E. Fernández and A. Whiting, Springer, Cham, Switzerland, 2015, pp. 1–37 Search PubMed.
- J. Cid, H. Gulyás, J. J. Carbó and E. Fernández, Chem. Soc. Rev., 2012, 41, 3558–3570 RSC.
- M. Yamashita, Bull. Chem. Soc. Jpn., 2011, 84, 983–999 CrossRef CAS.
- L. Weber, Eur. J. Inorg. Chem., 2012, 5595–5609 CrossRef CAS.
- Y. Segawa, M. Yamashita and K. Nozaki, Science, 2006, 314, 113–115 CrossRef CAS PubMed.
- Y. Segawa, Y. Suzuki, M. Yamashita and K. Nozaki, J. Am. Chem. Soc., 2008, 130, 16069–16079 CrossRef CAS PubMed.
- M. Yamashita, Y. Suzuki, Y. Segawa and K. Nozaki, J. Am. Chem. Soc., 2007, 129, 9570–9571 CrossRef CAS PubMed.
- Y. Okuno, M. Yamashita and K. Nozaki, Angew. Chem., Int. Ed., 2011, 50, 920–923 CrossRef CAS PubMed.
- N. Dettenrieder, Y. Aramaki, B. M. Wolf, C. Maichle-Mössmer, X. Zhao, M. Yamashita, K. Nozaki and R. Anwander, Angew. Chem., Int. Ed., 2014, 53, 6259–6262 CrossRef CAS PubMed.
- H. Hayashi, Y. Segawa, M. Yamashita and K. Nozaki, Chem. Commun., 2011, 47, 5888–5890 RSC.
- K. Nozaki, Y. Aramaki, M. Yamashita, S.-H. Ueng, M. Malacria, E. Lacôte and D. P. Curran, J. Am. Chem. Soc., 2010, 132, 11449–11451 CrossRef CAS PubMed.
- A. Rit, J. Campos, H. Niu and S. Aldridge, Nat. Chem., 2016, 8, 1022–1026 CrossRef CAS PubMed.
- A. V. Protchenko, D. Dange, A. D. Schwarz, C. Y. Tang, N. Phillips, P. Mountford, C. Jones and S. Aldridge, Chem. Commun., 2014, 50, 3841–3844 RSC.
- A. V. Protchenko, D. Dange, J. R. Harmer, C. Y. Tang, A. D. Schwarz, M. J. Kelly, N. Phillips, R. Tirfoin, K. H. Birjkumar, C. Jones, N. Kalsoyannis, P. Mountford and S. Aldridge, Nat. Chem., 2014, 6, 315–319 CrossRef CAS PubMed.
- B. Wang, Y. Li, R. Ganguly, H. Hirao and R. Kinjo, Nat. Commun., 2016, 7, 11871 CrossRef CAS PubMed.
- W. Lu, H. Hu, Y. Li, R. Ganguly and R. Kinjo, J. Am. Chem. Soc., 2016, 138, 6650–6661 CrossRef CAS PubMed.
- H. Braunschweig, C.-W. Chiu, K. Radacki and T. Kupfer, Angew. Chem., Int. Ed., 2010, 49, 2041–2044 CrossRef CAS PubMed.
- H. Braunschweig, M. Burzler, R. D. Dewhurst and K. Radacki, Angew. Chem., Int. Ed., 2008, 47, 5650–5653 CrossRef CAS PubMed.
- H. Braunschweig, R. D. Dewhurst, L. Pentecost, K. Radacki, A. Vargas and Q. Ye, Angew. Chem., Int. Ed., 2016, 55, 436–440 CrossRef CAS PubMed.
- R. Kinjo, B. Donnadieu, M. A. Celik, G. Frenking and G. Bertrand, Science, 2011, 333, 610–613 CrossRef CAS PubMed.
- D. A. Ruiz, M. Melaimi and G. Bertrand, Chem. Commun., 2014, 50, 7837–7839 RSC.
- L. Kong, Y. Li, R. Ganguly, D. Vidovic and R. Kinjo, Angew. Chem., Int. Ed., 2014, 53, 9280–9283 CrossRef CAS PubMed.
- L. Kong, R. Ganguly, Y. Li and R. Kinjo, Chem. Sci., 2015, 6, 2893–2902 RSC.
- D. Wu, L. Kong, Y. Li, R. Ganguly and R. Kinjo, Nat. Commun., 2015, 6, 7340 CrossRef CAS PubMed.
- T. Imamoto and T. Hikosaka, J. Org. Chem., 1994, 59, 6753–6759 CrossRef CAS.
- L. Kong, W. Lu, L. Yongxin, R. Ganguly and R. Kinjo, Inorg. Chem., 2017, 56, 5586–5593 CrossRef CAS PubMed.
- J. Monot, A. Solovyev, H. Bonin-Dubarle, É. Derat, D. P. Curran, M. Robert, L. Fensterbank, M. Malacria and E. Lacôte, Angew. Chem., Int. Ed., 2010, 49, 9166–9169 CrossRef CAS PubMed.
- D. A. Ruiz, G. Ung, M. Melaimi and G. Bertrand, Angew. Chem., Int. Ed., 2013, 52, 7590–7592 CrossRef CAS PubMed.
- E. Bernhardt, V. Bernhardt-Pitchougina, H. Willner and N. V. Ignatiev, Angew. Chem., Int. Ed., 2011, 50, 12085–12088 CrossRef CAS PubMed.
- J. Landmann, J. A. P. Sprenger, R. Bertermann, N. Ignat’ev, V. Bernhardt-Pitchougina, E. Bernhardt, H. Willner and M. Finze, Chem. Commun., 2015, 51, 4989–4992 RSC.
- J. Landmann, F. Keppner, D. B. Hofmann, J. A. P. Sprenger, M. Häring, S. H. Zottnick, K. Müller-Buschbaum, N. V. Ignat’ev and M. Finze, Angew. Chem., Int. Ed., 2017, 56, 2795–2799 CrossRef CAS PubMed.
-
J. Landmann, F. Keppner, J. A. P. Sprenger, M. Finze and N. Ignat’ev, DE102014018103A1, Julius-Maximilians-Universität Würzburg, Merck Patent GmbH, 2016.
- J. A. P. Sprenger, J. Landmann, M. Drisch, N. Ignat’ev and M. Finze, Inorg. Chem., 2015, 54, 3403–3412 CrossRef CAS PubMed.
-
N. Ignatyev, J. A. P. Sprenger, J. Landmann and M. Finze, WO2014198401, Merck Patent GmbH, 2014.
-
L. A. Bischoff, M. Drisch, C. Kerpen, P. T. Hennig, J. Landmann, J. A. P. Sprenger, N. Ignat’ev and M. Finze, in preparation.
-
M. Drisch, L. A. Bischoff, L. Herkert, J. A. P. Sprenger, M. Finze, N. Ignatyev and R. van Hal, WO2016074760, Merck Patent GmbH, 2016.
- W.-H. Guo, Q.-Q. Min, J.-W. Gu and X. Zhang, Angew. Chem., Int. Ed., 2015, 54, 9075–9078 CrossRef CAS PubMed.
- J. Zhou, M. W. Kuntze-Fechner, R. Bertermann, U. S. D. Paul, J. H. J. Berthel, A. Friedrich, Z. Du, T. B. Marder and U. Radius, J. Am. Chem. Soc., 2016, 138, 5250–5253 CrossRef CAS PubMed.
- T. Niwa, H. Ochiai, Y. Watanabe and T. Hosoya, J. Am. Chem. Soc., 2015, 137, 14313–14318 CrossRef CAS PubMed.
- X.-W. Liu, J. Echavarren, C. Zarate and R. Martin, J. Am. Chem. Soc., 2015, 137, 12470–12473 CrossRef CAS PubMed.
- S. I. Kalläne, M. Teltewskoi, T. Braun and B. Braun, Organometallics, 2015, 34, 1156–1169 CrossRef.
- M. Teltewskoi, J. A. Panetier, S. A. Macgregor and T. Braun, Angew. Chem., Int. Ed., 2010, 49, 3947–3951 CrossRef CAS PubMed.
- R. J. Lindup, T. B. Marder, R. N. Perutz and A. C. Whitwood, Chem. Commun., 2007, 3664–3666 RSC.
- A. M. Mfuh, J. D. Doyle, B. Chhetri, H. D. Arman and O. V. Larionov, J. Am. Chem. Soc., 2016, 138, 2985–2988 CrossRef CAS PubMed.
- K. Chen, M. S. Cheung, Z. Lin and P. Li, Org. Chem. Front., 2016, 3, 875–879 RSC.
- T. Ahrens, J. Kohlmann, M. Ahrens and T. Braun, Chem. Rev., 2015, 115, 931–972 CrossRef CAS PubMed.
- E. Clot, O. Eisenstein, N. Jasim, S. A. Macgregor, J. E. McGrady and R. N. Perutz, Acc. Chem. Res., 2011, 44, 333–348 CrossRef CAS PubMed.
- H. Amii and K. Uneyama, Chem. Rev., 2009, 109, 2119–2183 CrossRef CAS PubMed.
- D. O’Hagan, Chem. Soc. Rev., 2008, 37, 308–319 RSC.
- T. G. Richmond, Angew. Chem., Int. Ed., 2000, 39, 3241–3244 CrossRef CAS PubMed.
- W. Chen, C. Bakewell and M. R. Crimmin, Synthesis, 2017, 49, 810–821 CAS.
- G. M. Brooke, J. Fluorine Chem., 1997, 86, 1–76 CrossRef.
- R. D. Chambers, W. K. R. Musgrave, J. S. Waterhouse and D. L. H. Williams, J. Chem. Soc., Chem. Commun., 1974, 239–240 RSC.
- V. V. Aksenov, V. M. Vlasov and G. G. Yakobson, J. Fluorine Chem., 1982, 20, 439–458 CrossRef CAS.
- Y. Sun, H. Sun, J. Jia, A. Du and X. Li, Organometallics, 2014, 33, 1079–1081 CrossRef CAS.
- M. Schlosser and E. Marzi, Chem.–Eur. J., 2005, 11, 3449–3454 CrossRef CAS PubMed.
- H. H. Büker, N. M. M. Nibbering, D. Espinosa, F. Mongin and M. Schlosser, Tetrahedron Lett., 1997, 38, 8519–8522 CrossRef.
- C. Wu and F. Shi, Asian J. Org. Chem., 2013, 2, 116–125 CrossRef CAS.
- J. Landmann, J. A. P. Sprenger, M. Hailmann, V. Bernhardt-Pitchougina, H. Willner, N. Ignat’ev, E. Bernhardt and M. Finze, Angew. Chem., Int. Ed., 2015, 54, 11259–11264 CrossRef CAS PubMed.
-
J. A. P. Sprenger, L. A. Bischoff, M. Drisch, L. Herkert, M. Finze, H. Willner, E. Bernhardt, N. Ignatyev and M. Schulte, WO2016058665, Merck Patent GmbH, 2016.
- R. Bertermann, H. Braunschweig, R. D. Dewhurst, C. Hörl, T. Kramer and I. Krummenacher, Angew. Chem., Int. Ed., 2014, 53, 5453–5457 CrossRef CAS PubMed.
- D. P. Curran, A. Boussonnière, S. J. Geib and E. Lacôte, Angew. Chem., Int. Ed., 2012, 51, 1602–1605 CrossRef CAS PubMed.
- N. V. Ignat’ev, M. Finze, J. A. P. Sprenger, C. Kerpen, E. Bernhardt and H. Willner, J. Fluorine Chem., 2015, 177, 46–54 CrossRef.
Footnote |
† Electronic supplementary information (ESI) available: Additional tables, experimental information, analytical data and details of the DFT calculations. CCDC 1548893–1548910. For ESI and crystallographic data in CIF or other electronic format see DOI: 10.1039/c7sc02249b |
|
This journal is © The Royal Society of Chemistry 2017 |
Click here to see how this site uses Cookies. View our privacy policy here.