DOI:
10.1039/C7FO00983F
(Paper)
Food Funct., 2018,
9, 426-439
Hypoglycemic and hypolipidemic effects of anthocyanins extract from black soybean seed coat in high fat diet and streptozotocin-induced diabetic mice
Received
3rd July 2017
, Accepted 23rd November 2017
First published on 24th November 2017
Abstract
Black soybean seed coat extract (BSSCE) is a rich source of anthocyanins with multiple health effects. This study was aimed at investigating the composition and hypoglycemic and hypolipidemic effects of BSSCE in vitro and in a high-fat diet and streptozotocin (STZ)-induced diabetic mice. The anthocyanins of BSSCE were identified as cyanidin-3-O-glucoside, delphinidin-3-O-glucoside, and peonidin-3-O-glucoside by HPLC-MS. Results demonstrated that BSSCE exhibited strong inhibitory activities for α-amylase, potent inhibition activity against lipid accumulation in HepG2 cells and protection effect on H2O2-induced oxidative stress-damaged HepG2 cells. The food and water intake, body weight loss, blood glucose and insulin level of BSSCE treatment group were found to be significantly reduced when compared with those of diabetic mice group (p < 0.05). The fasting blood glucose level and insulin level of the BSSCE 400 mg kg−1 group mice significantly decreased by 47.97% and 46.49%, respectively. The oral glucose tolerance and activities of antioxidant enzymes including superoxide dismutase (SOD), glutathione peroxidase (GPX) and catalase (CAT) notably improved (p < 0.05). BSSCE could also ameliorate the atherogenic dyslipidaemia of diabetic mice by remarkably decreasing the content of total cholesterol (T-CHO), total triglyceride (TG), and non-esterified fatty acid (NEFA) and increasing the content of high-density lipoprotein cholesterol (HDL-c) (p < 0.05). BSSCE could protect against liver, kidney and pancreas damages in diabetic mice. This study suggested that cyanidin-3-O-glucoside contributed to BSSCE-induced hypoglycemia and hypolipidemia effects in type 2 diabetes mellitus (T2DM), and BSSCE might be a promising functional food or medicine for T2DM treatment.
1. Introduction
Type 2 diabetes mellitus (T2DM), a non-insulin-dependent DM, is a complex metabolic disease that affects more than 90% of the diabetic population and is mainly characterized by hyperglycemia and insulin resistance caused by the impairment of insulin secretion.1 Furthermore, hyperglycemia in diabetes further results in long-term damage and complications, including heart and blood vessel disease; nephropathy; and tissue impairments of the liver, kidney, and pancreas.2 Although the etiology of diabetes has not been fully elucidated, many experimental and clinical studies have revealed that the overweight and obese population is more vulnerable to T2DM, and obesity can even lead to a dramatic rise in probability of T2DM.3 There have been various drugs for treatment of diabetes for many years, including biguanide, thiazolidinedione, sulfonylurea, α-glycosidase inhibitors, and insulin; however, these agents are restricted and may have several significant side effects.4 However, mounting evidence indicates that dietary modifications can prevent and alleviate a range of metabolic abnormalities characterized by central obesity, dyslipidemias and high fasting glucose.5 Therefore, a large number of dietary plants and their bioactive constituents have been used to treat diabetes and its complications due to their efficacy and safety.
Anthocyanins are water-soluble natural pigments widely distributed in plants and are found in grains, vegetables, beans, fruits, and some colorful flowers; anthocyanins are often consumed by humans as part of their daily diet.6 They not only impart color to plants but also exhibit multiple health effects, such as antioxidant, anticancer, antidiabetic, and antimicrobial activities.7 A study by Hertog et al. showed that the average human intake of anthocyanins in the USA is about 180 to 215 mg d−1; however, the daily intake of most other dietary flavonoids, including genistein and quercetin is estimated to be only 20 to 25 mg d−1.8 Other publications have reported that the daily consumption of anthocyanins through one's diet ranges from 3 to 215 mg d−1.9,10
Black soybean (Glycine max (L.) Merr.) has been widely used as a nutritionally rich food and traditional medicine in Asia (mainly in Korea, China, and Japan) for hundreds of years,11 and its seed coat contains abundant levels of anthocyanins (cyanidin-3-O-glucoside; C3G)12 that exhibit various beneficial bioactivities, such as antioxidant,13 anti-diabetic and anti-obesity effects.14 Some previous studies have demonstrated that black soybean seed coat extracts could act as a functional food in the prevention of obesity, hyperglycemia and inflammation-induced diseases.12,14 High fat diet and streptozotocin (STZ)-induced type 2 diabetes in mice is similar to the development of human noninsulin-dependent T2DM, and this mice model is widely employed for screening potential therapies for the treatment of T2DM.15,16 However, little is known about the hypoglycemic and hypolipidemic effects of anthocyanin extracts from black soybean seed coat in vitro and in high fat diet and STZ-induced type 2 diabetic mice.
In this study, the anthocyanins of BSSCE were extracted and the composition was analyzed by HPLC-MS. The hypoglycemic and hypolipidemic effects of BSSCE in high fat diet and streptozotocin-induced insulin-resistant diabetic mice were evaluated. The hypoglycemic and hypolipidemic activities and protection against oxidative damage in HepG2 cells of BSSCE in vitro were also investigated.
2. Materials and methods
2.1. Materials and chemicals
Black soybean seed coat was purchased from a local market in Zhaozhuang (Shandong province, China). STZ and all fine chemicals were obtained from Sigma-Aldrich (St Louis, MO, USA). Rosiglitazone was purchased from Hengrui Pharmaceutical Co., Ltd (Chengdu, China). Commercial kits for the determination of glycogen, TG (triglyceride), T-CHO (total cholesterol), LDL-c (low-density lipoprotein cholesterol), HDL-c (high-density lipoprotein cholesterol), GSP (glycated serum protein), NEFA (non-esterified fatty acid), SOD (superoxide dismutase), CAT (catalase), GPX (glutathione peroxidase) and MDA (methylene dioxyamphetamine) were purchased from Nanjingjiancheng Technology Co. (Nanjing, China). An insulin ELISA assay kit was acquired from Wuhan Huamei Biotech Co., Ltd (Wuhan, China). All other chemicals were obtained from local firms and were of analytical grade.
2.2. Determination of the content of total polyphenols and anthocyanins
Preparation of black soybean seed coat anthocyanin extracts was according to a previous method.17 Then the crude extract was sequentially extracted using ethyl acetate and n-butanol. Anthocyanin quantification was performed by the pH differential method using cyanidin-3-O-glucoside as the standard.18 The content of total polyphenols was determined using the Folin–Ciocalteu method with gallic acid as the standard.19 The n-butanol extract with the high anthocyanin content was named as BSSCE and used for further study.
2.3. HPLC-ESI-QqQ-MS analysis
Chromatographic analysis of BSSCE was performed on an Agilent 1200 series HPLC system equipped with a quaternary SL pump, diode-array detection (DAD) and an online degasser (Agilent Technologies, Santa Clara, CA, USA). Chromatographic separation was carried out on a C18 column (250 mm × 4.6 mm i.d., 5 mm, Kromasil C18). The mobile phase consisted of 0.1% formic acid in water (A) and acetonitrile (B). The elution process was performed at 30 °C with a linear gradient of 10% B at 0–2 min, 10–40% B at 2–15 min and 40% B at 15–30 min and at a flow rate of 0.5 mL min−1. The sample injection volume was 10 μL and detected at 530 nm.
MS detection was performed on an Agilent G6400 Triple Quadrupole MS (Agilent Technologies, Santa Clara, CA, USA) equipped with an electrospray ionization (ESI) source. The sample ionization was achieved in the positive ESI mode within the mass/charge (m/z) range of 50–1500. The other conditions were as follows: drying gas flow (N2), 12.0 L min−1; nebulizer pressure, 50 psi; gas drying temperature, 370 °C; and capillary voltage, 3500 V.
2.4. α-Amylase inhibitory activity of BSSCE
The α-amylase inhibitory activity of BSSCE was measured according to our previous study20 with a slight modification. Briefly, 200 μL of sodium phosphate buffer containing NaCl (6 mM, pH 6.9) was mixed with 100 μL of soluble starch (0.05%) as a substrate and 100 μL of our extracts (0.25–1.25 mg mL−1). 100 μL of the buffer was used as the blank sample and acarbose as the positive control. After thoroughly mixing, all test tubes were preincubated at 37 °C for 10 min. Then 100 μL of α-amylase (10 U) was added into the mixture to start the reaction and the mixture system was incubated at 37 °C for 5 min. Subsequently, 2.0 mL of DNS reagent (1% dinitrosalicylic acid, 12% potassium sodium tartrate) was added and the mixture was incubated at 100 °C for 5 min. After cooling to room temperature, the reaction mixture was diluted by adding 3 mL of distilled water and the absorbance was measured at 540 nm.
2.5. Inhibitory effect of BSSCE on lipid accumulation in HepG2 cell
2.5.1 Cell culture and MTT assay.
The hepatoma cell line HepG2 was obtained from Chinese Academy of Sciences Committee on Type Culture Collection Cell Bank (Shanghai, China). Cells were cultured in Dulbecco modified Eagle medium (DMEM) with 10% FBS, 100 U per mL penicillin, and 100 g mL−1 streptomycin and incubated at 37 °C under 5% CO2 atmosphere. In this study, we aimed to study the inhibitory effect of BSSCE on lipid accumulation in HepG2 cells. However, the cytotoxicity effect of BSSCE needed to be avoided, which could influence the results of lipid accumulation analysis. The HepG2 cell viability activity was evaluated by using the MTT assay according to our previous study.21 Briefly, HepG2 cells were seeded into 96-well plates at a density of 6 × 103 cells per well and then treated with 100 μL samples in DMEM of different concentration (20–200 μg mL−1). After incubation for 48 h, 50 μL of the MTT solution (2 mg mL−1) was added and incubated for 4 h at 37 °C under 5% CO2 atmosphere. Then, the MTT solution was discarded and 150 μL of dimethyl sulfoxide (DMSO) was added. The optical density of each well was measured at 492 nm by Multiskan Spectrum (DNM-9602, Perlong Scientific, China).
2.5.2 Oil red O staining.
The inhibitory effect of BSSCE on lipid accumulation in HepG2 cells was evaluated by oil red O staining. HepG2 cells were seeded at a density of 1.0 × 104 on 96-well plates. After 24 h, the cells were grouped into normal control, model control and sample control groups. The cell culture media of the model control and sample control groups were supplemented with palmitate (lipid-containing media) to develop the lipid disorder model according to the previous method22 with some modifications. Briefly, the cell culture media of the model control and sample control groups were supplemented with 100 μL palmitate
:
BSA (fatty acid free) solution (1
:
9 volume ratio) and the cells were further incubated for 24 h. The final concentration of palmitate in the medium was 0.04 mM. (For preparation of palmitate–BSA solution, 0.4 mM sodium palmitate was prepared in PBS and heated at 60 °C for 5 min. Then 4% fatty acid free BSA (dissolved in DMEM) was slowly added to palmitate solution. Finally, the palmitate–BSA solution was gently rotated for 1 h at 37 °C.) Then cells were treated with BSSCE (100 μL) at different concentrations, which depended on the MTT assay. After 24 h incubation, the cells were rinsed with cold phosphate buffered saline (PBS) and fixed in 4% v/v paraformaldehyde for 30 min. Then the lipid droplets were stained with oil red O (0.5% v/v).23 Images of cells were captured using an optical inverted microscope (TS100, Nikon eclipse, Japan; 10× magnification) and lipid accumulation was calculated and quantified by Image J (National Institutes of Health).
2.6. Determination of CAT, SOD, and GPX activities and MDA levels in H2O2-induced HepG2 cells
In this assay, H2O2-induced oxidative damage in HepG2 cells was induced to evaluate the protection effect of BSSCE according to the previous study.24,25 Briefly, the cytotoxicity of H2O2 and BSSCE with different concentrations treatment were measured using the MTT assay and the optimal concentration of H2O2 was 100 μM for the oxidative stress damage of HepG2 cells. Then, HepG2 cells were cultured in 6-well plates (2.0 × 106 cells per well). After 24 h incubation, the assays were performed as follows: (1) BSSCE (final concentration of 40, 80 and 120 μg mL−1) were added into the protection groups; (2) the damage group was treated with DMEM alone; (3) the control group was also treated with DMEM alone. After 24 h protection, the BSSCE group and damage group were charged with 100 μM H2O2 for 4 h to induce oxidative stress, and the control group was treated with DMEM alone in the same manner. Subsequently, cells were lysed using the lysis buffer and centrifuged for 10 min at 14
000g and 4 °C. Then the liquid supernatant was collected for enzyme activity assay. SOD, CAT, and GPX activities and the content of MDA were measured using assay kits according to the manufacturer's protocols. Protein concentrations in cell lysates were quantified with a bicinchoninic acid (BCA) assay kit (KeyGEN Biotech, China). Enzymatic activities were normalized by protein content (U per mg prot).
2.7. Animals
Kunming mice (20 ± 2 g, certificate number: SXCK (Jing) 2014-0008) were purchased from Beijing HFK Bioscience Co., Ltd. The mice were housed in standard cages at a constant temperature (22 ± 1 °C) and humidity (a relative humidity of 60 ± 5%), under a 12 h light/dark cycle, with free access to food and water. All experimental procedures were carried out according to National Institutes of Health (NIH) guidelines and approved by The Ethics Committee on animal experiments of Institute of Radiation Medicine Chinese Academy of Medical Science (the approval number is (2016) 6-018).
2.8. Experimental design
2.8.1 Development of T2DM.
The T2DM animal model was established by high fat diet (HFD) and streptozotocin (STZ) according to our previous method.16 Briefly, after 7 days of accommodation, excluding the normal control (NC) group with normal diet, other mice were fed with a high fat diet (HFD): 2% cholesterol, 0.2% sodium cholate, 5% sugar, 15% coconut oil, 0.6–0.8% salt, 0.8% trace element additive, and 76.2% commercial standard pellet diet (composition and energy of diets are presented in Table 1). After 4 weeks, the mice were injected intraperitoneally with 90 mg kg−1 streptozotocin dissolved in citrate buffer solution (0.1 M, pH 4.5). Citrate buffer alone was injected in the mice of the NC group. Blood samples were withdrawn from the tail vein of the overnight fasted mice 3 days after STZ induction. Mice with fasting blood glucose (FBG) level >11.1 mmol L−1 were recognized as T2DM and used for future study.
Table 1 Normal and HFD diets fed to mice
|
Normal pellet diets (g per 100 g diet) |
HFDs (g per 100 g diet) |
Ingredient
|
Casein |
20.0 (80.0 kcal) |
20.0 (80.0 kcal) |
L-Cysteine |
0.3 (0 kcal) |
0.3 (0 kcal) |
Corn starch |
36.5 (146.0 kcal) |
22 (88.0 kcal) |
Sucrose |
35.0 (136.2 kcal) |
12.5 (48.63 kcal) |
Soybean oil |
5.0 (45.0 kcal) |
20.0 (180.0 kcal) |
Cellulose |
1.4 (0 kcal) |
1.4 (0 kcal) |
Sodium cholate |
0.2 (0 kcal) |
0.2 (0 kcal) |
Salt |
0.8 (0 kcal) |
0.8 (0 kcal) |
Trace element additive |
0.8 (0 kcal) |
0.8 (0 kcal) |
Coconut oil |
— |
15 (134.9 kcal) |
Maltodextrin 10 |
— |
5 (20.00 kcal) |
Cholesterol |
— |
2 (14.3 kcal) |
Protein (% of energy) |
20 |
14 |
Carbohydrate (% of energy) |
69 |
28 |
Fat (% of energy) |
11 |
58 |
Total energy (kcal per 100 g) |
407.2 |
565.8 |
2.8.2 Experimental design.
After T2DM model development, the diabetic mice were randomly divided into 5 groups of 8 mice each. Positive control (PC) with rosiglitazone 2 mg per kg b.w. dissolved in normal saline (NS) per day; diabetic control (DC) with high fat diet; and other three groups were treated with BSSCE dissolved in NS at the doses of 100 (LD), 200 (MD) and 400 (HD) mg kg−1 day−1. In addition, the NC group consisted of 8 normal mice with free access to a normal diet and treated with saline. All the mice were given free access to water and administered continuously for 28 days. Fasting blood glucose (FBG) of mice was measured once a week using a glucometer. Food intake, water consumption, and body weight of experimental mice were recorded every day during the experimental period. On the 28th day of the experiment, the mice were fasted overnight and blood samples were collected in dry tubes without anticoagulants and separated from serum through centrifugation and were immediately stored at −20 °C for biochemical assays. After blood sample collection, all the mice were sacrificed. The pancreas, liver and kidney were removed and washed in NS, and portions of each were fixed in 10% formalin (pH 7.4) for biochemical estimations and histological examinations.
2.9. Oral glucose tolerance test (OGTT)
Oral glucose tolerance test (OGTT) test was performed on the 25th day after sample administration. After a 12 h fasting, the mice were orally administered with glucose (1.5 g per kg b.w.) and blood samples were collected from the caudal vein at 0, 30, 60 and 120 min after glucose administration to measure blood glucose levels. The results of OGTT were expressed as an integrated area under the curve (AUC) over 120 min and the AUC was calculated by GraphPad Prism version 5.0.
2.10. Biochemical analysis
GSP (minimum detectable concentration (MDC): 0.05 mmol L−1), NEFA (MDC: 0.01 mmol L−1), TG (linear range: 0–9.04 mmol L−1; MDC: 0.1 mmol L−1), T-CHO (linear range: 0–10.34 mmol L−1; MDC: 0.1 mmol L−1), LDL-c (MDC: 0.1 mmol L−1), HDL-c (MDC: 0.01 mmol L−1), MDA (linear range: 1.5–24 nmol mg−1 protein; MDC: 0.1 nmol mg−1 protein) and glycogen contents (MDC: 10 μg g−1 protein), SOD (linear range: 93.79–1077.10 U per mg protein, MDC: 23.46 U per mg protein), CAT (linear range: 0–80 U per mg protein; MDC: 1.0 U per mg protein) and GPX (MDC: 1.0 U per mg protein) activities were all determined by colorimetric methods following the instructions of commercial kits (Nanjingjiancheng Technology Co., Nanjing, China). The serum insulin level was determined according to the enzyme-linked immunosorbent (ELISA) method using a commercial kit (Wuhan Huamei Biotech Co., Ltd. Wuhan, China) (linear range: 6.638–424 mIU L−1; MDC: 3.986 mIU L−1).
The HOMA method developed by Matthews et al.26 was used to estimate insulin resistance (HOMA-IR) and dysfunction of β-cells (HOMA β-cell). The results were calculated according to the following formulae: HOMA-IR = [fasting insulin (mIU L−1) × fasting glucose (mmol L−1)/22.5] and HOMA-β = [(20 × fasting insulin mIU L−1)/(fasting glucose mmol L−1 − 3.5)]. The insulin sensitivity index (ISI) was calculated by relative formula27 ISI = Ln (1/(FBG × FSI)).
2.11. Histological evaluation
For the histopathological examination, the pancreas, liver and kidney tissues were fixed in 10% formalin and embedded in paraffin wax for pathological cut sheet. Sections (5 mm thicknesses) were stained with hematoxylin–eosin and examined using an electron microscope (TE2000-U, Nikon eclipse, Japan; 400× magnification).
2.12. Statistical analyses
The data was expressed as mean ± S.D. Statistical analysis of the data was done using the SPSS version 20.0. One-way ANOVA test (Dunnett's test) was used for the statistical analyses. P value of 0.05 or 0.01 was considered as statistically significant.
3. Results
3.1. Total phenolic and anthocyanin content
The extraction yield of crude anthocyanin extract from black soybean seed coat can reach approximately 8.0% (w/w) of the fresh dried weight. Total phenolic content (TPC) and total anthocyanin content (TAC) of the extract were expressed as gallic acid equivalents and cyanidin-3-O-glucoside equivalents, respectively. The TPC was 342.73 mg gallic acid equivalent (GAE) per gram of extract. The TAC was 88.11 mg cyanidin-3-O-glucoside equivalent (Cy3GE) per gram of extract. Then, the extract was further extracted by ethyl acetate (to remove the non-anthocyanin polyphenols) and n-butanol. The significantly highest anthocyanin content (73.93%) was obtained in the n-butanol fraction, indicating BSSCE is a cyanidin-3-O-glucoside-enriched extract. The TAC of BSSCE was 119.18 mg Cy3GE/1 g BSSCE.
3.2. Anthocyanin composition of BSSCE
The anthocyanin compositions of BSSCE were identified by HPLC-ESI-QqQ-MS. Fig. 1 shows the HPLC chromatogram of BSSCE at 530 nm. One major peak was observed, which was attributed to cyanidin-3-O-glucoside. In addition, delphinidin-3-O-glucoside and peonidin-3-O-glucoside were also observed and identified in BSSCE (Table 2). The data confirmed that anthocyanins were indeed present in BSSCE and cyanidin-3-O-glucoside was the major anthocyanin, with a relative peak area composition of 90.16%.
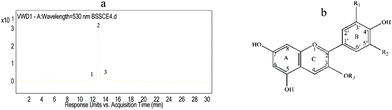 |
| Fig. 1 Anthocyanin analysis of BSSCE. (a) HPLC chromatogram of BSSCE at 530 nm; (b) structures of principal anthocyanins identified in BSSCE. Peak 1: Delphinidin-3-O-glucoside: R1 = OH, R2 = OH, R3 = glucose; Peak 2: cyanidin-3-O-glucoside: R1 = OH, R2 = H, R3 = glucose; Peak 3: peonidin-3-O-glucoside: R1 = OCH3, R2 = OH, R3 = glucose. All measurements were run in triplicate (n = 3). | |
Table 2 Chromatographic and spectroscopic characteristics of principal anthocyanins identified from BSSCE
No. |
T
R (min) |
[M + H]+ (m/z) |
MS2 (m/z) |
Identity |
1 |
12.06 |
465 |
303 |
Delphinidin-3-O-glucoside |
2 |
12.89 |
449 |
287 |
Cyanidin-3-O-glucoside |
3 |
13.90 |
463 |
301 |
Peonidin-3-O-glucoside |
3.3. Hypoglycemic and hypolipidemic effect of BSSCE in vitro
Fig. 2a indicates that BSSCE exhibited a significantly stronger does-dependent inhibitory activity for α-amylase than acarbose (p < 0.05). The MTT results showed that the cell viability of BSSCE treatment (20–200 μg ml−1) was higher than 80% (Fig. 2b), which indicated that BSSCE with the concentration ranging from 20 to 200 μg ml−1 had no significant cytotoxicity against HepG2 cell lines during the period of incubation.28 An intermediate concentration of BSSCE from 40 to 120 μg ml−1 was selected for further inhibitory effect investigation on lipid accumulation in the HepG2 model, as the HepG2 cell line reserves the morphology of hepatocytes and most of their functions during culturing. HepG2 cells were induced with palmitate to mimic the lipid disorders of type 2 diabetes.22 The lipid droplet in the cell was stained with an oil red O kit. There were abundant lipid droplets observed in the model control (palmitate administration) compared with those in the NC (Fig. 2c). After BSSCE treatment, the lipid accumulation area notably decreased, and the quantitative calculation by Image J showed that lipid accumulation could be alleviated by BSSCE in a dose-dependent manner (Fig. 2d), which proved that BSSCE has the potential to ameliorate lipid disorders in diabetes. The above results suggested that BSSCE exhibited strong hyperglycemic and hypolipidemic effects in vitro.
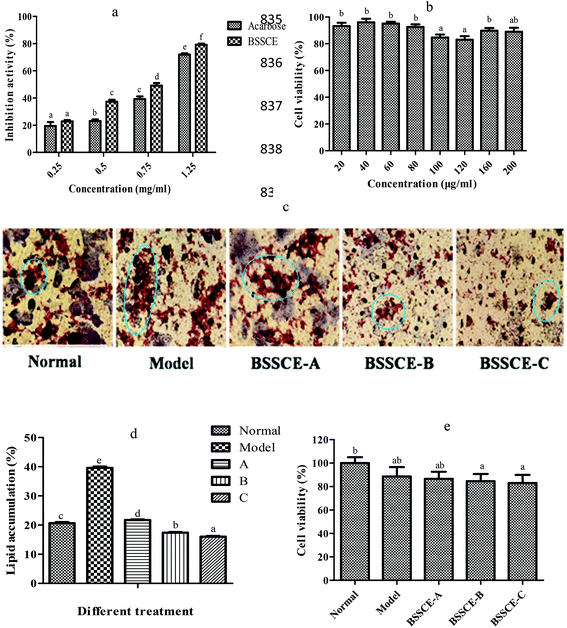 |
| Fig. 2 Hyperglycemic and hypolipidemic activities of BSSCE in vitro. (a) α-Amylase inhibitory activity of BSSCE; (b) cell viability of HepG2 treated with different concentrations of BSSCE; (c) inhibitory activity of BSSCE on lipid accumulation in HepG2 (oil red O staining images under microscope), Normal: without palmitate and BSSCE; Model: palmitate (40 μM) treated group; BSSCE-A: 40 μg ml−1; BSSCE-B: 80 μg ml−1; BSSCE-C: 120 μg ml−1; (d) inhibitory activity of BSSCE on lipid accumulation in HepG2 cells, qualitative calculation by Image J. A: BSSCE, 40 μg ml−1; B: BSSCE, 80 μg ml−1; C: BSSCE, 120 μg ml−1; (e) cell viability of HepG2 treated with different concentrations of H2O2 and BSSCE. Normal: without H2O2 and BSSCE, Model: H2O2 (100 μM) treated group; BSSCE-A: 40 μg ml−1; BSSCE-B: 80 μg ml−1; BSSCE-C: 120 μg ml−1. All data are expressed as mean ± S.D. Different letters indicate significant differences (p < 0.05). All measurements were run in triplicate (n = 3). | |
3.4. Protection effect of BSSCE on H2O2-induced oxidative damage in HepG2 cells
Studies have shown that oxidative stress plays a major role in the development of chronic and degenerative ailments such as cancer, aging, cardiovascular disease and diabetes.29,30 In this study, the effects of BSSCE on the intracellular antioxidant enzyme activities of CAT, SOD, and GPX and MDA levels in H2O2 induced HepG2 cells were evaluated in order to elucidate whether BSSCE could exhibit oxidative stress inhibition effect and whether the hypoglycemic and hypolipidemic effects of BSSCE were associated with the protection of the antioxidant defense systems.
The MTT assay results showed that the cell viability of H2O2 group and BSSCE treatment (40, 80 and 120 μg ml−1) was over 80% (Fig. 2e), which indicated that 100 μM H2O2 and BSSCE treatment group showed no significant cytotoxicity against HepG2 cell lines during the period of incubation and could be used for further experiments. Enzyme activity assay showed that a significant (p < 0.05) decrease in the activities of CAT, SOD and GPX was observed in the HepG2 cells exposed to H2O2 compared those of the NC group (Table 3), which suggested that the oxidative stress damaged HepG2 cell model was successfully established. However, the pretreatment of cells with different concentrations of BSSCE (40, 80 and 120 μg ml−1) significantly increased CAT by 36.17%, 42.87% and 48.85%; SOD by 46.80%, 58.89% and 77.13%; and GPX by 30.21%, 58.01% and 95.15%, respectively. In addition, the MDA level of BSSCE treatment (40, 80 and 120 μg ml−1) also significantly decreased by 37.13%, 48.53% and 55.70%, respectively.
Table 3 Effects of BSSCE on CAT, GPX, SOD and MDA in H2O2-induced HepG2 cells
Group |
CAT (U per mg protein) |
SOD (U per mg protein) |
GPX (U per mg protein) |
MDA (nmol mg−1 protein) |
NC: normal control; H2O2: H2O2 (100 μM) treated groups; BSSCE-A: 40 μg ml−1; BSSCE-B: 80 μg ml−1; BSSCE-C: 120 μg ml−1. All data are expressed as mean ± S.D. Different letters indicate significant differences (p < 0.05). All measurements were run in triplicate (n = 3). |
NC |
27.52 ± 0.45e |
211.91 ± 14.65d |
246.55 ± 12.73d |
1.13 ± 0.11a |
H2O2 |
16.89 ± 0.70a |
107.83 ± 5.08a |
90.03 ± 10.12a |
3.07 ± 0.23d |
BSSCE-A |
23.00 ± 0.11b |
158.29 ± 5.07b |
117.23 ± 5.64ab |
1.93 ± 0.09c |
BSSCE-B |
24.03 ± 0.35c |
171.33 ± 4.58b |
142.26 ± 8.24b |
1.58 ± 0.07b |
BSSCE-C |
25.04 ± 0.28d |
191.00 ± 5.80c |
175.69 ± 13.65c |
1.36 ± 0.11b |
3.5. Effect of BSSCE on body weight, organ index, food and water intake
In this study, the dose of the positive control drug rosiglitazone used for mice was 2 mg kg−1, which was calculated and extrapolated according to the dosage instructions (for humans). The selected concentrations of the extract (100, 200, 400 mg kg−1) were according to those in previous studies,31,32 where the extracts studied have similar composition as our extract and show a reduced hepatic lipid accumulation effect and hypoglycemic effect. The body weight gain rate in the diabetic control group after 4 weeks was only 1.89%, which was significantly less than that of the NC group (7.68%) (p < 0.01) (Table 4). The administration of BSSCE notably resulted in a remarkable elevation, in a dose dependent manner, in body weight after 4 weeks as compared with mice in the diabetic control group (4.04, 5.79% and 6.20% vs. 1.89%, p < 0.01), and there was no significant effect of BSSCE (400 mg kg−1) and the positive control drug rosiglitazone on body weight gain (6.20% vs. 6.08%) (Table 4). The weight of the liver, spleen and thymus index of the diabetic control group exhibited a notable increase compared to that of the NC group (p < 0.05) (Table 5), while the pancreas weight decreased. However, after administration of BSSCE for four weeks, BSSCE suppressed these trends and the related tissue index was restored to the normal level. There was no obvious change found in the heart tissue of the different experimental groups.
Table 4 Effects of BSSCE on body weights (g) of STZ/HFD induced diabetic mice
Week |
NC |
DC |
PC |
LD |
MD |
HD |
WG%: weight gain rate (%). NC: normal control; DC: diabetic group; PC: positive control, rosiglitazone; LD: BSSCE (100 mg kg−1); MD: BSSCE (200 mg kg−1); HD: BSSCE (400 mg kg−1). Values are expressed as mean ± S.D. of 8 mice per group. 0*: The body weights of mice in the 0* row refer to the starting body weights of mice after T2DM development and do not refer to the initial body weight. The initial body weight of mice was 20 ± 2 g and was equal for all groups. Different lowercase letters in the same column indicate significant differences among the four-week treatments for a single group (p < 0.05). Different capital letters in the same row indicate significant differences between the six groups (p < 0.05). |
0* |
44.82 ± 0.80Ca |
40.99 ± 0.78Aa |
41.42 ± 0.65ABa |
42.11 ± 0.50Ba |
42.28 ± 0.39Ba |
42.45 ± 0.25Ba |
1 |
45.40 ± 0.37Ca |
41.47 ± 0.40Aab |
42.33 ± 0.33Bb |
42.55 ± 0.41Bab |
42.76 ± 0.32Bab |
42.73 ± 0.30Ba |
2 |
46.19 ± 0.42Db |
41.22 ± 0.33Aab |
42.74 ± 0.32Bb |
42.96 ± 0.41BCb |
43.25 ± 0.35BCb |
43.27 ± 0.16Cb |
3 |
47.22 ± 0.41Db |
41.68 ± 0.32Ab |
43.75 ± 0.50BCc |
43.30 ± 0.35Bbc |
44.05 ± 0.35Cc |
44.06 ± 0.23Cc |
4 |
48.26 ± 0.42Db |
41.73 ± 0.39Ab |
43.94 ± 0.46Bc |
43.81 ± 0.39Bc |
44.73 ± 0.38Cd |
45.08 ± 0.30Cd |
WG% |
7.68 ± 0.49D |
1.81 ± 0.11A |
6.08 ± 0.40C |
4.04 ± 0.23B |
5.79 ± 0.0.28C |
6.20 ± 0.39C |
Table 5 Effects of BSSCE on organ index in STZ/HFD induced diabetic mice
Group |
Liver (mg g−1) |
Kidney (mg g−1) |
Spleen (mg g−1) |
Thymus (mg g−1) |
Pancreas (mg g−1) |
Heart (mg g−1) |
NC: normal control; DC: diabetic control; PC: positive control, rosiglitazone; LD: BSSCE (100 mg kg−1); MD: BSSCE (200 mg kg−1); HD: BSSCE (400 mg kg−1). Values are expressed as mean ± S.D. of 8 mice per group. Different letters in the same column indicate significant differences (p < 0.05). |
NC |
61.57 ± 3.18a |
14.76 ± 0.53ab |
3.47 ± 0.58ab |
2.26 ± 0.29a |
5.64 ± 0.62c |
6.22 ± 0.80b |
DC |
78.15 ± 9.20b |
16.45 ± 1.31b |
5.33 ± 0.60cd |
3.18 ± 0.64b |
3.78 ± 0.62a |
6.25 ± 0.54b |
PC |
61.05 ± 11.85a |
13.64 ± 1.81a |
3.80 ± 0.28bc |
2.34 ± 0.39a |
5.28 ± 0.03bc |
5.63 ± 0.77b |
LD |
64.17 ± 8.16a |
14.35 ± 1.00a |
2.89 ± 0.36ab |
2.09 ± 0.35a |
5.12 ± 0.50bc |
4.60 ± 0.70a |
MD |
66.65 ± 5.51ab |
14.29 ± 1.30a |
3.53 ± 0.38b |
2.21 ± 0.50a |
4.60 ± 0.61b |
5.52 ± 0.53ab |
HD |
66.13 ± 6.81a |
14.71 ± 0.41a |
2.85 ± 0.30a |
2.09 ± 0.34a |
5.63 ± 0.56c |
5.63 ± 0.56b |
The food and water intake changes of the NC group were negligible over the 4 weeks (Fig. 3). However, the food and water intake of the diabetic control group sequentially increased over the 4 weeks. After BSSCE treatment for 4 weeks, food and water consumption was significantly (p < 0.01) lower compared with that of the diabetic control group. Especially, the food intake of the three BSSCE groups had no significant difference from that of the PC group.
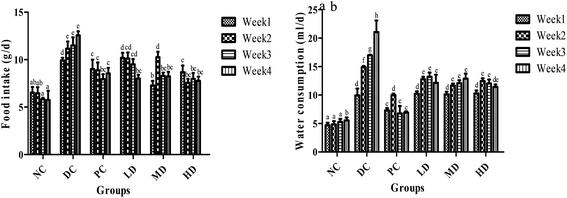 |
| Fig. 3 Effects of BSSCE on food intake and water consumption of STZ/HFD induced diabetic mice. (a) Food intake (g d−1); (b) water consumption (ml d−1). NC: normal control; DC: diabetic control; PC: positive control, rosiglitazone; LD: BSSCE (100 mg kg−1); MD: BSSCE (200 mg kg−1); HD: BSSCE (400 mg kg−1). Different letters indicate significant differences (p < 0.05). Data are expressed as mean ± SD (n = 8). | |
3.6. Fasting blood glucose (FBG), oral glucose tolerance test (OGTT) and insulin levels
As shown in the FBG test, the blood glucose of the diabetic control group increased significantly (p < 0.01) after the injection of STZ when compared with the NC group and gradually increased over 4 weeks (Fig. 4a), which might be due to the impairment of insulin secretion induced by STZ.33,34 Before the positive drug and BSSCE treatment, the glucose levels of the four diabetic groups (PC, LD, MD, and HD group) were almost identical. Compared with the diabetic control group, BSSCE-intake group FBG significantly decreased at week 3 and 4 (LD decreased by 31.44%, MD decreased by 39.05%, HD decreased by 47.97% at the 4th week, p < 0.01), and especially BSSCE 400 mg kg−1 could be as effective as the positive drug rosiglitazone, which indicated that BSSCE could observably alleviate the FBG of diabetic mice.
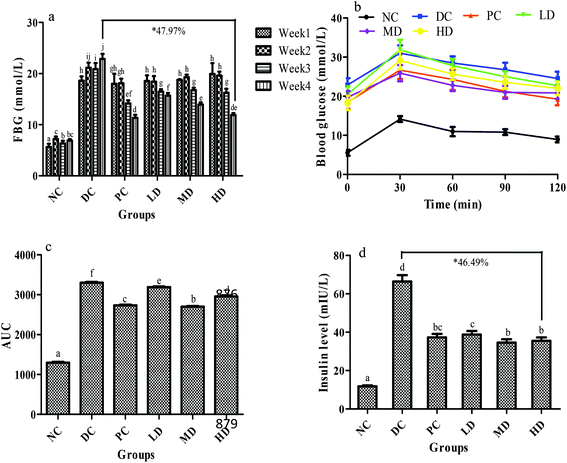 |
| Fig. 4 Effects of BSSCE on serum glucose and insulin of STZ/HFD induced diabetic mice. (a) FBG; (b) OGTT; (c) AUC; (d) insulin levels (mIU L−1). NC: normal control; DC: diabetic control; PC: positive control, rosiglitazone; LD: BSSCE (100 mg kg−1); MD: BSSCE (200 mg kg−1); HD: BSSCE (400 mg kg−1). * The decrease percentage of FBG (a) or insulin level (d) for the HD group compared with that for the DC group at the 4th week. Different letters indicate significant differences (p < 0.05). Data are expressed as mean ± SD (n = 8). | |
Fig. 4b shows the OGTT test results. The blood glucose in all groups reached its peak value in 30 min, and that in the NC group then gradually decreased to almost its initial level. Compared with the DC group, the MD and HD groups exhibited a significant decrease (p < 0.01) in fasting blood glucose at 30 min, which indicated that BSSCE could effectively suppress the glucose peak value. The AUC could indicate the potent of blood glucose tolerance and the BSSCE treated groups showed a significant lower AUC value than the DC group (p < 0.01) (Fig. 4c). BSSCE 200 mg kg−1 exhibited a lower AUC value than the positive control group (p < 0.05). The results demonstrated that BSSCE possessed the potency for diabetic treatment.
The serum insulin level of the DC group was abnormally elevated compared with that of the NC group (p < 0.01) (Fig. 4d), which could trigger insulin resistance. However, BSSCE significantly suppressed this trend and lowered the insulin level (LD decreased by 41.57%, MD decreased by 47.89%, HD decreased by 46.49%, p < 0.01). In addition, the insulin resistance (HOMA-IR), dysfunction of β-cells (HOMA-β) and insulin sensitivity index (ISI) in mice were also calculated to assess the insulin metabolism. The HOMA-IR of the DC group was significantly higher than that of the NC group (p < 0.01), which in turn markedly decreased ISI (p < 0.01). Compared with those in the DC group, the serum levels of HOMA-IR in the positive control group and BSSCE treated groups were notably lowered, which in turn progressively increased ISI (p < 0.01) (Table 6). The HOMA-β of the DC group had no significant difference when compared with that of the NC group (p > 0.05), while the HOMA-β of the positive control and HD groups remarkably increased compared to that of the DC group (p < 0.01).
Table 6 Effects of BSSCE on HOMA-IR, HOMA-β and ISI in STZ/HFD induced diabetic mice
Group |
FBG (mmol L−1) |
FSI (mIU L−1) |
HOMA-IR |
HOMA-β |
ISI |
NC: normal control; DC: diabetic control; PC: positive control, rosiglitazone; LD: BSSCE (100 mg kg−1); MD: BSSCE (200 mg kg−1); HD: BSSCE (400 mg kg−1). FBG: fasting blood glucose of mice (4-week treatment by extracts); FSI: fasting serum insulin of mice (immediately determined after mice sacrifice after 4-week treatment by extracts); HOMA-IR: insulin resistance index; HOMA-β: dysfunction index of β-cells; ISI: insulin sensitivity index. Values are expressed as mean ± S.D. of 8 mice per group. Different letters in the same column indicate significant differences (p < 0.05). |
NC |
6.88 ± 0.27a |
11.73 ± 0.57a |
3.59 ± 0.69a |
69.43 ± 4.26a |
−4.39 ± 0.19c |
DC |
22.87 ± 1.00e |
66.40 ± 3.31d |
67.50 ± 4.70d |
68.55 ± 6.63a |
−7.32 ± 0.12a |
PC |
11.33 ± 0.60b |
37.33 ± 1.79bc |
18.80 ± 4.80b |
95.32 ± 5.97c |
−6.05 ± 0.07b |
LD |
15.68 ± 0.53d |
38.80 ± 1.82c |
27.04 ± 4.30c |
63.71 ± 6.88a |
−6.41 ± 0.34b |
MD |
13.94 ± 0.42c |
34.60 ± 1.73b |
21.44 ± 3.23b |
66.28 ± 8.23a |
−6.17 ± 0.32b |
HD |
11.90 ± 0.37b |
35.53 ± 1.74b |
18.79 ± 2.86b |
84.60 ± 9.41b |
−6.05 ± 0.44b |
3.7. Effects of BSSCE on glycogen content in liver and muscle
The glycogen content in liver and muscle are shown in Fig. 5. Compared with that of the NC group, the glycogen content in liver and muscle of the DC group decreased significantly (p < 0.01). It was found that BSSCE treatment for 4 weeks dose-dependently caused a significant increase (p < 0.01) in the glycogen content both in the liver and muscle when compared with that of the DC group; especially, BSSCE treatment at a dose of 400 mg kg−1 could result in the increase of the glycogen content by 50.10% in the liver and 62.71% in the muscle.
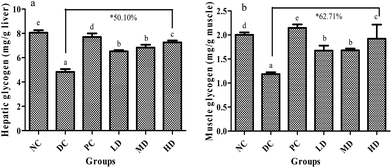 |
| Fig. 5 Effects of BSSCE on glycogen content of STZ/HFD induced diabetic mice. (a) Hepatic glycogen content (mg g−1 liver); (b) muscle glycogen content (mg g−1 muscle). NC: normal control; DC: diabetic control; PC: positive control, rosiglitazone; LD: BSSCE (100 mg kg−1); MD: BSSCE (200 mg kg−1); HD: BSSCE (400 mg kg−1). * The increase percentage of glycogen content of the HD group compared with that of the DC group at the 4th week. Different letters indicate significant differences (p < 0.05). Data are expressed as mean ± SD (n = 8). | |
3.8. Effects of BSSCE on serum lipid profiles of diabetic mice
The effects of BSSCE on lipid abnormalities in diabetic mice are summarized in Table 7. Compared with the NC group, the DC group exhibited notably higher levels (p < 0.01) of serum NEFA, TG, TC and LDL-c and lower levels of HDL-c (p < 0.01); however, BSSCE intake for 4 weeks resulted in a significant decrease (p < 0.01) in the levels of serum NEFA, TG, and TC and a marked increase (p < 0.01) in the HDL-c level. While LDL-c level of the BSSCE treated groups showed no significant difference compared with that of the DC group, but the ratio of LDL/HDL in the MD and HD groups obviously decreased (9.1% for MD and 8.3% for HD vs. 14.6% for DC, p < 0.01). The results also showed that BSSCE could cause a significant decrease (p < 0.01) in the GSP level compared with diabetic control, especially, the GSP content of the HD group was restored to the normal level (2.87 vs. 2.80 mmol L−1, p > 0.05).
Table 7 Effects of BSSCE on serum NEFA, GSP and lipid profiles in STZ/HFD induced diabetic mice
Group |
GSP (mmol L−1) |
NEFA (mmol L−1) |
T-CHO (mmol L−1) |
TG (mmol L−1) |
HDL-C (mmol L−1) |
LDL-C (mmol L−1) |
LDL-C/HDL-C (%) |
NC: normal control; DC: diabetic control; PC: positive control, rosiglitazone; LD: BSSCE (100 mg kg−1); MD: BSSCE (200 mg kg−1); HD: BSSCE (400 mg kg−1). Values are expressed as mean ± S.D. of 8 mice per group. Different letters in the same column indicate significant differences (p < 0.05). |
NC |
2.80 ± 0.11a |
1.90 ± 0.12a |
6.00 ± 0.52b |
1.08 ± 0.13a |
7.37 ± 0.35d |
0.54 ± 0.17a |
7.33 ± 0.49a |
DC |
3.40 ± 0.11b |
3.36 ± 0.80b |
9.19 ± 0.37c |
3.58 ± 0.23c |
5.75 ± 0.07b |
0.84 ± 0.15b |
14.61 ± 2.14b |
PC |
2.91 ± 0.30a |
2.15 ± 0.15a |
5.14 ± 0.57ab |
1.15 ± 0.48ab |
7.06 ± 0.12c |
0.61 ± 0.23ab |
8.64 ± 1.92a |
LD |
3.08 ± 0.19a |
2.43 ± 0.18a |
6.52 ± 0.66b |
1.75 ± 0.77b |
5.33 ± 0.06a |
0.75 ± 0.31ab |
14.07 ± 5.17b |
MD |
2.97 ± 0.20a |
2.17 ± 0.17a |
6.34 ± 0.73b |
1.60 ± 0.31ab |
7.00 ± 0.14c |
0.64 ± 0.07ab |
9.14 ± 0.50a |
HD |
2.87 ± 0.18a |
2.13 ± 0.16a |
5.12 ± 0.58a |
1.36 ± 0.38ab |
7.34 ± 0.17d |
0.61 ± 0.08ab |
8.31 ± 0.47a |
3.9. Effects of BSSCE on antioxidant indices and MDA level in liver
As shown in Table 8, the diabetic control group caused a significant decrease in activities of SOD, CAT, and GPX and increase in MDA level compared with those of the NC group (p < 0.01). After BSSCE treatment, the activities of CAT and GPX obviously increased and MDA content decreased (p < 0.01) compared to those of the DC group. However, there was no significant difference in the SOD activity of the positive control, MD and HD groups when compared with that of the DC group (p > 0.05). The SOD activity of the LD group (100 mg kg−1 BSSCE) was significantly higher than that of the DC group (p < 0.05).
Table 8 Effects of BSSCE on antioxidant enzymes and MDA level in STZ/HFD induced diabetic mice
Group |
CAT (U per mg protein) |
SOD (U per mg protein) |
GPX (U per mg protein) |
MDA (nmol mg−1 protein) |
NC: normal control; DC: diabetic control; PC: positive control, rosiglitazone; LD: BSSCE (100 mg kg−1); MD: BSSCE (200 mg kg−1); HD: BSSCE (400 mg kg−1). Values are expressed as mean ± S.D. of 8 mice per group. Different letters in the same column indicate significant differences (p < 0.05). |
NC |
43.28 ± 5.37cd |
329.35 ± 9.89d |
672.01 ± 5.86d |
3.25 ± 0.13a |
DC |
21.38 ± 3.02a |
250.30 ± 12.21a |
426.73 ± 12.84a |
4.88 ± 0.63bc |
PC |
30.93 ± 4.23b |
278.91 ± 11.93bc |
602.64 ± 14.49c |
4.12 ± 0.83b |
LD |
32.86 ± 3.40bc |
290.72 ± 16.17c |
510.01 ± 12.31b |
4.76 ± 0.28bc |
MD |
40.93 ± 4.78cd |
268.57 ± 16.46ab |
506.73 ± 44.45b |
3.57 ± 0.54ab |
HD |
38.43 ± 4.02c |
271.95 ± 4.57b |
623.53 ± 14.27c |
3.37 ± 0.35ab |
3.10. Histopathological assay on the tissues of the BSSCE treated diabetic mice
The liver histology results showed that the NC group exhibited usual hepatic cell morphology and architecture (Fig. 6A1). Compared with the NC group, severe liver damage such as focal necrosis, infiltration of lymphocytes, congestion in the central vein and mussy hepatic cords were observed in the diabetic control group (Fig. 6A2). The rosiglitazone exhibited a positive effect on the STZ-induced pathological changes (Fig. 6A3). BSSCE significantly attenuated these histopathological changes (Fig. 6A4–6) and no clear histopathological abnormalities were observed in the HD group (Fig. 6A6).
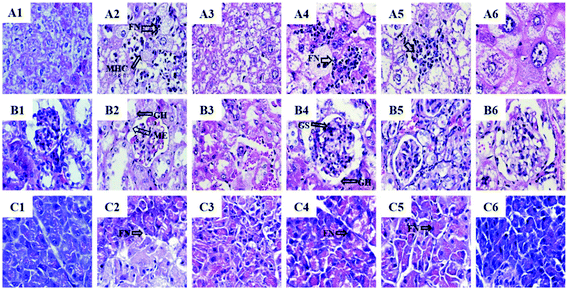 |
| Fig. 6 Histopathological studies of BSSCE on the liver, kidney and pancreas of STZ/HFD-induced diabetic mice. (A) Liver (H&E stain, 400×): A1 (normal control); A2 (diabetic control); A3 (positive control, rosiglitazone); A4 (BSSCE 100 mg kg−1); A5 (BSSCE 200 mg kg−1); A6 (BSSCE 400 mg kg−1). (B) Kidney (H&E stain, 400×): B1 (normal control); B2 (diabetic control); B3 (positive control, rosiglitazone); B4 (BSSCE 100 mg kg−1); B5 (BSSCE 200 mg kg−1); B6 (BSSCE 400 mg kg−1). (C) Pancreas (H&E stain, 400×): C1 (normal control), C2 (diabetic control); C3 (positive control, rosiglitazone); C4 (BSSCE 100 mg kg−1); C5 (BSSCE 200 mg kg−1); C6 (BSSCE 400 mg kg−1). FN: focal necrosis; MHC: mussy hepatic cords; GS: glomerular sclerosis; ME: mesangial expansion; GH: glomerular hypertrophy. | |
Histopathology of the NC group showed a normal kidney histology (Fig. 6B1), while severe kidney damage with glomerular sclerosis, hypertrophy and mesangial expansion appeared in the diabetic mice (Fig. 6B2). The BSSCE groups obviously alleviated the histological damage (Fig. 6B4–6) and the kidney histology of the HD group was almost restored to the normal level.
Complete pancreatic structure and uniform arrangement of numerous pancreatic β-cells were observed in the NC group (Fig. 6C1). In contrast, the DC group showed obvious islet cell damage with a loose arrangement and focal necrosis (Fig. 6C2). Each BSSCE group exhibited damage alleviation effects to different degrees (Fig. 6C4–6), and the number of pancreatic β-cells and islet morphology were almost recovered to normal. All the above histopathological results demonstrated that BSSCE could postpone and treat liver, kidney and pancreas damage, and exert a protection effect on STZ-induced diabetic mice; thus, BSSCE may serve as a potential resource for prevention and treatment of T2DM.
4. Discussion
In this study, the results indicated that Cy3G was the major anthocyanin of the black soybean seed coat, which is in accordance with a previous report.17 The Cy3G-enriched extract from the black soybean seed coat could ameliorate type 2 diabetes by differentiating preadipocytes into smaller and insulin-sensitive adipocytes.35 The present study showed that BSSCE has high content of total anthocyanins (119.18 mg g−1) and exhibited strong inhibitory activity for α-amylase in vitro and potential inhibition activity against lipid accumulation in HepG2 cells. Besides, H2O2-induced HepG2 cells were used to investigate the protection effect of BSSCE on oxidative stress damage and the results showed that BSSCE could protect H2O2-induced HepG2 cells from oxidative stress by enhancing endogenous antioxidant defense systems including increasing CAT, SOD and GPX activities and reducing MDA content; this suggested that the hypoglycemic and hypolipidemic effects of BSSCE in vitro might be associated with the protection of antioxidant defense systems.
BSSCE intake in vivo for 4 weeks significantly attenuated the hyperglycemia and hyperlipidemia syndromes and improved insulin resistance and antioxidant enzymes activities in diabetic mice. These findings suggested that BSSCE could exhibit great anti-diabetic effects in vitro and in vivo, and anthocyanins, especially cyanidin-3-O-glucoside, one of the major constituents of the BSSCE extract, played an important role in exerting hypoglycemic and hypolipidemic activities.
Black soybean seed coat extract and Cy3G had been shown prevent obesity and hyperglycemia in DIO mice or C57BL/6 mice in previous studies.14,36 However, this study is the first report showing the hypoglycemic and hypolipidemic activities of anthocyanin extracts from black soybean seed coat in vitro and in STZ/HFD induced diabetic mice. It was found that BSSCE could mitigate body weight loss and decrease the food and water intake of diabetic mice (Table 4 and Fig. 3), and some organ weights were restored to the normal level by alleviating hepatomegaly and renal swelling (Table 5). The body weight loss and the increase of organ index were considered to be the typical T2DM characteristics induced by STZ and HFD.37 The results indicated that BSSCE could ameliorate the decrease in body weight and improve the quality of life in diabetic mice.
Hyperglycemia is the most important symptom of T2DM.38 The fasting blood glucose (FBG) value and oral glucose tolerance test (OGTT) are important indicators of diabetes alleviation. In this research, administration of BSSCE to diabetic mice markedly reduced FBG at week 3 and 4 (Fig. 4a). OGTT indicated that glucose tolerance AUC of the BSSCE groups was significantly lower than that of the diabetic control group (p < 0.05) (Fig. 4b and c), indicating BSSCE has possible hypoglycemic effect in type 2 diabetes. The BSSCE 200 mg kg−1 group exhibited a lower AUC value than the BSSCE 400 mg kg−1 group (p < 0.05), which might be because the bioavailability of high-dose BSSCE was not linearly higher than that of low- and medium-dose BSSCE. Similar results were also reported by previous studies. The study of green rooibos extract (SB1) from Rooibos (a kind of herbal tea) showed that the medium-dose SB1 group showed lower AUC than the high-dose SB1 group.39 Polyphenol enriched extracts (RPEs) of Rosa rugosa Thunb. in medium doses showed higher oral glucose tolerance than RPEs in high doses.40 The hypoglycemic effect investigation of an acidic polysaccharide (SERP1) isolated from Sarcandra glabra (Thunb.) also showed that the extract in medium doses exhibited lower AUC than that in high doses.41
Previous studies and our research reveal that glycogen storage can be decreased in diabetes,42–44 which was attributed to glycolipid metabolism disorder caused by insulin resistance in diabetes. In the current study, BSSCE effectively increased the glycogen content both in the liver and muscle compared with that in the diabetic control group (Fig. 5), indicating that BSSCE might alleviate hyperglycemia by regulating glycogen synthesis and glucose metabolism.42,45
Insulin resistance is another major symptom of T2DM and is associated with insulin secretion impairment caused by STZ injection and hyperglycemia.16 In our study, the diabetic control group exhibited hyperinsulinemia symptoms, indicating that the mice developed insulin resistance (Fig. 4d). On the other hand, BSSCE treatment remarkedly decreased the serum insulin levels of diabetic mice by more than 41.77% compared with that of the diabetic control mice. The insulin resistance index (HOMA-IR), HOMA-β and insulin sensitivity index (ISI) were calculated to estimate insulin metabolism. The results exhibited that a higher level of HOMA-IR and lower level of ISI in the DC group mice showed disturbance in β-cell function based on the above serum insulin level assay (Table 6). However, the HOMA-IR level and ISI of the BSSCE treated mice was significantly decreased and increased, respectively, indicating that BSSCE could improve β-cell function and insulin resistance. Also, HOMA-IR and ISI values of the BSSCE treated groups showed no significant differences with those of the PC group (p > 0.05), which indicated that BSSCE treatment could be as effective as the positive drug rosiglitazone. In this study, the dose of the positive control drug rosiglitazone used for mice was 2 mg kg−1, which was calculated according to the dosage instructions (for humans: 8 mg day−1; for an adult weighing 50–70 kg) and extrapolated based on the dose conversion method46 (the dose for mice: mg per kg BW = 9.1 × the dose of humans (mg per kg BW)). Similar results were also reported in previous studies. Li et al.27 and Man et al.38 respectively revealed that cinnamon polyphenol and Litchi chinensis Sonn. seeds extract (LSE)-treated mice exhibited lower HOMA-IR level and higher ISI level than the diabetic control group.
The HOMA-β values of the positive control and HD groups remarkably increased compared to that of the DC group (p < 0.01). Though HOMA-β value of the DC group (68.55 ± 6.63) was lower than that of the NC group (69.43 ± 4.26), there was no significant difference (p > 0.05), which might be due to the severely higher fasting serum insulin (FSI) value of the former compared to that of the latter (about 5.7-fold, 66.4 mIU L−1 for DC vs. 11.73 mIU L−1 for NC, see Table 6). Similarly, in the study of Li et al.,27 the fasting serum insulin (FSI) value of the DC group (51.24 ± 7.67 mIU L−1) was also severely higher than that of the NC group mice (16.87 ± 4.59 mIU L−1) (about 3.0-fold). After calculation, the HOMA-β of the DC group (79.07 ± 2.89) also showed no significant difference when compared with that of the NC group (79.18 ± 8.23) (p > 0.05). In these cases, insulin could be accumulated and not be used more due to the insulin resistance of the diabetic mice. Thus, the abnormally high insulin level could lead to a high value of HOMA-β. Previous research also reported that monitoring HOMA indices was not helpful to assess β-cell function and insulin resistance when some of the patients use exogenous insulin injection (insulin is injected in to the body, leading to abnormally high insulin level).47 In this case, HOMA-β might not truly reflect the islet function. The insulin levels, HOMA-IR and ISI analysis suggested that BSSCE might mitigate insulin secretion impairment and further exert hypoglycemic effects.
In addition, long-term insulin resistance can disturb both glucose and lipid metabolism and further result in abnormal higher lipid and glucose levels.48 Hyperlipidemia and dyslipidemia are often closely associated with diabetes, which is attributed to the metabolic disorder caused by diabetes mellitus.42 As previously reported, anthocyanins could exert cardiovascular protection by improving lipid homeostasis by reducing TC, TG, and LDL-c levels and increasing HDL-c levels.49 In this study, BSSCE intake significantly decreased TC, TG levels, and LDL/HDL ratio and increased HDL levels in diabetic mice (Table 7). Increased levels of TC and TG are associated with the risk of diabetes, whereas increased HDL levels alleviate diabetes.48 Furthermore, the obvious decreased ratio of LDL/HDL (so-called atherosclerosis index) in the MD and HD group (p < 0.05) can reduce the risk of cardiovascular disease.50 The LDL/HDL ratio of BSSCE treated groups showed no significant differences with that of the PC group (p > 0.05), which indicated that BSSCE treatment could be as effective as positive drug rosiglitazone. GSP, formed by the reaction between glucose and N-terminal of other protein molecules, can indicate the serum glucose level.43 BSSCE treatment markedly decreased the GSP levels (p < 0.01) to show hypoglycemic effect (Table 7). The results demonstrated that supplementation of BSSCE rich in anthocyanins could ameliorate the dyslipidemia of diabetic mice and exert cardiovascular protection.
Studies have shown that excessive oxidative stress in body cells plays an important role in the development of diabetes, and the persistent hyperglycemia of diabetes can also lead to increased oxidative stress in the body;29,30 therefore, the inhibition or reduction of oxidative stress can be an additional therapy for preventing or delaying the incidence of diabetes. Kruger et al. revealed that grape and black rice anthocyanins could effectively limit the oxidant stress in vitro and in vivo.49 A review by Thompson et al. elucidated that anthocyanins can potentially exert antioxidant capacity under high oxidative stress such as obesity/hypercholesterolemia.13 In this study, significantly increased activities of SOD, CAT, and GPX and decreased MDA levels were found in the BSSCE groups (p < 0.05) (Table 8), which were consistent with the results of antioxidant enzyme activity assay in H2O2 induced HepG2 cells. The SOD, CAT and GPX activities of the BSSCE treated groups showed no significant differences when compared with those of the positive control group, which suggested that BSSCE treatment could be as effective as the positive drug rosiglitazone. The results suggested that BSSCE could suppress oxidant stress by improving the activities of SOD, CAT, and GPX antioxidant enzymes, which might be attributed to hyperglycemia and hyperlipidemia alleviation effect of BSSCE. In addition, long-term excessive oxidative stress in diabetes could cause tissue damage. Organ weights indicated that BSSCE could alleviate the hepatomegaly and renal swelling, and the pancreas weights of BSSCE group have been restored to normal level (Table 5). Histopathological examination indicated that the liver, kidney and pancreas suffered different levels of damage in the STZ/HFD-induced diabetic mice (Fig. 6), while BSSCE intake for 4 weeks alleviated these lesions and exerted protection.
The selected concentration of anthocyanin extract (BSSCE: 100, 200, 400 mg kg−1) from black soybean seed coat in this study can be obtained by humans through their diet. In humans, the dose conversion method based on the equivalent coefficient is as follows: the dose of mice (mg per kg BW) = 9.1 × the dose of humans (mg per kg BW);46 thus, the dose of mice (100, 200, 400 mg kg−1 BSSCE) can be converted to the dose of humans (mg per kg BW): 10.99, 21.98, and 43.96 mg kg−1 BSSCE, respectively. For an adult with an average body weight of 60 kg, about 0.66 to 2.64 g BSSCE (containing 78.66 to 314.64 mg anthocyanins, calculated based on the fact that the total anthocyanin content of BSSCE was 119.18 mg Cy3GE/1 g BSSCE) should be orally administered daily for similar effects as observed in the present study. According to the Hygienic Standard for Food Additives in China (GB 2760-2014) and previous literature,51 anthocyanins or black soybean seed coat extract can be used as additives, such as in red wines and beverages. Thus, in theory, 300 mL of red wine, beverages, porridge and other nutraceutical (containing 0.66 to 2.64 g BSSCE or 78.66 to 314.64 mg anthocyanins) can be consumed daily for similar effects as observed in the present study. Other publications have reported that the daily consumption of anthocyanins in the diet should range from 3 to 215 mg d−1.9,10 However, the consumption and bioavailability of BSSCE in humans remains to be further studied.
5. Conclusion
The hypoglycemic and hypolipidemic effects of anthocyanin extracts from black soybean seed coat (BSSCE) in vitro and in STZ/HFD induced diabetic mice were investigated for the first time in this study. The study demonstrated that the major anthocyanin composition of BSSCE was analyzed and identified as cyanidin-3-O-glucoside, with little delphinidin-3-O-glucoside and peonidin-3-O-glucoside. The results demonstrated that BSSCE exhibited strong inhibitory activity for α-amylase and potential inhibition activity against lipid accumulation in HepG2 cells and protection to HepG2 cells damaged by H2O2 induced oxidative stress. BSSCE could significantly ameliorate hyperglycemia and hyperlipidemia in diabetic mice via significantly decreasing the body weight loss, blood glucose level, and serum lipid levels and the glucose tolerance was improved. Insulin resistance of diabetic mice was notably mitigated by BSSCE and the antioxidant activities of SOD, GPX and CAT were remarkably improved in the liver tissue. In addition, the histological evaluation showed that BSSCE treatment could protect the pancreas, liver and kidney from damage and dysfunction. Results suggested that cyanidin-3-O-glucoside might play key role in the hypoglycemic and hypolipidemic effects of BSSCE for preventing and treating T2MD. BSSCE could be of great value in serving as a potential candidate for T2DM treatment or a related functional food for T2DM prevention.
Conflicts of interest
There are no conflicts of interest to declare.
Acknowledgements
This work was supported by the grant from National High Technology Research and Development Program (“863”Program) of China (Grant No. SS2013AA100207) and the National Natural Science Foundation of China (NSFC 31371879).
References
- W. Liu, Y. Zheng, Z. Zhang, W. Yao and X. Gao, Hypoglycemic, hypolipidemic and antioxidant effects of Sarcandra glabra polysaccharide in type 2 diabetic mice, Food Funct., 2014, 5, 2850–2860 Search PubMed.
- A. J. Akindele, E. Otuguor, D. Singh, D. Ota and A. S. Benebo, Hypoglycemic, antilipidemic and antioxidant effects of valproic acid in alloxan-induced diabetic rats, Eur. J. Pharmacol., 2015, 762, 174–183 CrossRef PubMed.
- H. Guo and W. Ling, The update of anthocyanins on obesity and type 2 diabetes: experimental evidence and clinical perspectives, Rev. Endocr. Metab. Disord., 2015, 16, 1–13 CrossRef PubMed.
- P. K. Prabhakar, R. Prasad, S. Ali and M. Doble, Synergistic interaction of ferulic acid with commercial hypoglycemic drugs in streptozotocin induced diabetic rats, Phytomedicine, 2013, 20, 488–494 CrossRef PubMed.
- C. J. Andersen and M. L. Fernandez, Dietary strategies to reduce metabolic syndrome, Rev. Endocr. Metab. Disord., 2013, 14, 241–254 CrossRef PubMed.
- C. Zhang, H. Jia, W. Wu, X. Wang, J. Fang and C. Wang, Functional conservation analysis and expression modes of grape anthocyanin synthesis genes responsive to low temperature stress, Gene, 2015, 574, 168–177 CrossRef PubMed.
- T. L. Jeng, J. S. Yi, M. T. Wu and J. M. Sung, Comparisons of flavonoids and anti-oxidative activities in seed coat, embryonic axis and cotyledon of black soybeans, Food Chem., 2010, 123, 1112–1116 CrossRef.
- M. G. Hertog, P. C. Hollman, M. B. Katan and D. Kromhout, Intake of potentially anticarcinogenic flavonoids and their determinants in adults in the Netherlands, Nutr. Cancer, 1993, 20, 21–29 CrossRef PubMed.
- O. K. Chun, S. J. Chung and W. O. Song, Estimated dietary flavonoid intake and major food sources of U.S. adults, J. Nutr., 2007, 137, 1244–1252 Search PubMed.
- X. L. Wu, G. R. Beecher, J. M. Holden, D. B. Haytowitz, S. E. Gebhardt and R. L. Prior, Concentrations of anthocyanins in common foods in the United States and estimation of normal consumption, J. Agric. Food Chem., 2006, 54, 4069–4075 CrossRef PubMed.
- B. Xu and S. K. C. Chang, Antioxidant capacity of seed coat, dehulled bean, and whole black soybeans in relation to their distributions of total phenolics, phenolic acids, anthocyanins, and isoflavones, J. Agric. Food Chem., 2008, 56, 8365–8373 CrossRef PubMed.
- Y. Kurimoto, Y. Shibayama, S. Inoue, M. Soga, M. Takikawa, C. Ito, F. Nanba, T. Yoshida, Y. Yamashita, H. Ashida and T. Tsuda, Black soybean seed coat extract ameliorates hyperglycemia and insulin sensitivity via the activation of amp-activated protein kinase in diabetic mice, J. Agric. Food Chem., 2013, 61, 5558–5564 CrossRef PubMed.
- K. Thompson, W. Pederick and A. B. Santhakumar, Anthocyanins in obesity-associated thrombogenesis: a review of the potential mechanism of action, Food Funct., 2016, 7, 2169–2178 Search PubMed.
- K. Soyoung, W. Haeri, C. Seojin, H. Taejoung, L. Byongwon and L. Myoungsook, Inhibitory effect of anthocyanin-rich black soybean testa (glycine max (l.) merr.) on the inflammation-induced adipogenesis in a DIO mouse model, J. Funct. Foods, 2015, 14, 623–633 CrossRef.
- K. Wang, Z. Tang, J. Wang, P. Cao, Q. Li, W. Shui, H. Wang, Z. Zheng and Y. Zhang, Polysaccharide from angelica sinensis ameliorates high-fat diet and STZ-induced hepatic oxidative stress and inflammation in diabetic mice by activating the sirt1-AMPK pathway, J. Nutr. Biochem., 2017, 43, 88–97 CrossRef PubMed.
- C. Wang, Z. Chen, Y. Pan, X. Gao and H. Chen, Anti-diabetic effects of inonotus obliquus, polysaccharides-chromium(III) complex in type 2 diabetic mice and its sub-acute toxicity evaluation in normal mice, Food Chem. Toxicol., 2017, 108, 498–509 CrossRef PubMed.
- H. L. Jin, N. S. Kang, S. O. Shin, S. H. Shin, S. G. Lim, D. Y. Suh, I. Y. Baek, K. Y. Park and T. J. Ha, Characterisation of anthocyanins in the black soybean (Glycine max, L.) by HPLC-DAD-ESI/MS analysis, Food Chem., 2009, 112, 226–231 CrossRef.
- J. M. Awika, L. W. Rooney and R. D. Waniska, Anthocyanins from black sorghum and their antioxidant properties, Food Chem., 2005, 90, 293–301 CrossRef.
- V. L. Singleton and J. A. Rossi, Colorimetry of total phenolics with phosphomolybdic-phosphotungstic acid reagents, Am. J. Enol. Vitic., 1965, 16, 144–158 Search PubMed.
- S. Chen, H. Chen, J. Tian, Y. Wang, L. Xing and J. Wang, Chemical modification, antioxidant and alpha-amylase inhibitory activities of corn silk polysaccharides, Carbohydr. Polym., 2013, 98, 428–437 CrossRef PubMed.
- Z. Chen, J. Wang, W. Liu and H. Chen, Physicochemical characterization, antioxidant and anticancer activities of proteins from four legume species, J. Food Sci. Technol., 2017, 54, 964–972 CrossRef PubMed.
- W. Chang, C. Li and G. M. Hatch, Berberine treatment attenuates the palmitate-mediated inhibition of glucose uptake and consumption through increased 1,2,3-triacyl-sn-glycerol synthesis and accumulation in H9C2 cardiomyocytes, Biochim. Biophys. Acta, Mol. Cell Res., 2016, 1861, 352–362 CrossRef PubMed.
- A. Stellavato, M. Lamberti, A. V. A. Pirozzi, F. D. Novellis and C. Schiraldi, Myclobutanil worsens nonalcoholic fatty liver disease: an in vitro, study of toxicity and apoptosis on HepG2 cells, Toxicol. Lett., 2016, 262, 100–104 CrossRef PubMed.
- S. Salla, R. Sunkara, S. Ogutu, L. T. Walker and M. Verghese, Antioxidant activity of papaya seed extracts against H2O2 induced oxidative stress in HepG2 cells, LWT–Food Sci. Technol., 2016, 66, 293–297 CrossRef.
- R. Liang, Z. Zhang and S. Lin, Effects of pulsed electric field on intracellular antioxidant activity and antioxidant enzyme regulating capacities of pine nut (Pinus Koraiensis) peptide QDHCH in HepG2 cells, Food Chem., 2017, 237, 793–802 CrossRef PubMed.
- D. R. Matthews, J. P. Hosker, A. S. Rudenski, B. A. Naylor, D. F. Treacher and R. C. Turner, Homeostasis model assessment: insulin resistance and β-cell function from fasting plasma glucose and insulin concentrations in man, Diabetologia, 1985, 28, 412–419 CrossRef PubMed.
- R. Li, T. Liang, L. Xu, Y. Li, S. Zhang and X. Duan, Protective effect of cinnamon polyphenols against STZ-diabetic mice fed high-sugar, high-fat diet and its underlying mechanism, Food Chem. Toxicol., 2013, 51, 419–425 CrossRef PubMed.
- Q. Huang, L. Chen, H. Teng, H. Teng, H. Song, X. Wu and M. Xu, Phenolic compounds ameliorate the glucose uptake in HepG2 cells’ insulin resistance via activating AMPK: Anti-diabetic effect of phenolic compounds in HepG2 cells, J. Funct. Foods, 2015, 19, 487–494 CrossRef.
- L. A. Pham-Huy, H. He and C. Pham-Huy, Free radicals, antioxidants in disease and health, Int. J. Biomed. Sci., 2008, 4, 89–96 Search PubMed.
- A. Saha and S. Mazumder, An aqueous extract of Murraya koenigii leaves induces paraoxonase 1 activity in streptozotocin induced diabetic mice, Food Funct., 2013, 4, 420–425 Search PubMed.
- Y. P. Hwang, J. H. Choi, E. H. Han, H. G. Kim, J. H. Wee, K. O. Jung and H. G. Jeong, Purple sweet potato anthocyanins attenuate hepatic lipid accumulation through activating adenosine monophosphate-activated protein kinase in human HepG2 cells and obese mice, Nutr. Res., 2011, 31, 896–906 CrossRef PubMed.
- C. W. Hsieh, J. Y. Cheng, T. H. Wang, H. J. Wang and W. J. Ho, Hypoglycaemic effects of Ajuga extract in vitro and in vivo, J. Funct. Foods, 2014, 6, 224–230 CrossRef.
- K. Wang, Z. Tang, Z. Zheng, P. Cao, W. Shui, Q. Li and Y. Zhang, Protective effects of Angelica sinensis polysaccharide against hyperglycemia and liver injury in multiple low-dose streptozotocin-induced type 2 diabetic BALB/c mice, Food Funct., 2016, 7, 4889–4897 Search PubMed.
- S. Li, H. Chen, J. Wang, X. Wang, B. Hu and F. Lv, Involvement of the PI3 K/Akt signal pathway in the hypoglycemic effects of tea polysaccharides on diabetic mice, Int. J. Biol. Macromol., 2015, 81, 967–974 CrossRef PubMed.
- T. Matsukawa, T. Inaguma, J. Han, M. O. Villareal and H. Isoda, Cyanidin-3-glucoside derived from black soybeans ameliorate type 2 diabetes through the induction of differentiation of preadipocytes into smaller and insulin-sensitive adipocytes, J. Nutr. Biochem., 2015, 26, 860–867 CrossRef PubMed.
- V. Talagavadi, P. Rapisarda, F. Galvano, P. Pelicci and M. Giorgio, Cyanidin-3-o-β-glucoside and protocatechuic acid activate AMPK/mTOR/S6 K pathway and improve glucose homeostasis in mice, J. Funct. Foods, 2016, 21, 338–348 CrossRef.
- E. Sezik, M. Aslan, E. Yesilada and S. Ito, Hypoglycaemic activity of gentiana olivieri and isolation of the active constituent through bioassay-directed fractionation techniques, Life Sci., 2005, 76, 1223–1238 CrossRef CAS PubMed.
- S. Man, J. Ma, C. Wang, Y. Li, W. Gao and F. Lu, Chemical composition and hypoglycaemic effect of polyphenol extracts from litchi chinensis seeds, J. Funct. Foods, 2016, 22, 313–324 CrossRef CAS.
- C. J. F. Muller, E. Joubert, D. de Beer, M. Sanderson, C. J. Malherbe, S. J. Fey and J. Louw, Acute assessment of an aspalathin-enriched green rooibos (Aspalathus Linearis) extract with hypoglycemic potential, Phytomedicine, 2012, 20, 32–39 CrossRef CAS PubMed.
- L. Liu, D. Tang, H. Zhao, X. Xin and H. A. Aisa, Hypoglycemic effect of the polyphenols rich extract from rose rugosa thunb on high fat diet and STZ induced diabetic rats, J. Ethnopharmacol., 2017, 200, 174–181 CrossRef CAS PubMed.
- W. Liu, W. Lu, Y. Chai, Y. Liu, W. Yao and X. Gao, Preliminary structural characterization and hypoglycemic effects of an acidic polysaccharide serp1 from the residue of Sarcandra glabra, Carbohydr. Polym., 2017, 176, 140–151 CrossRef CAS PubMed.
- C. Guo, C. Zhang, L. Li, Z. Wang, W. Xiao and Z. Yang, Hypoglycemic and hypolipidemic effects of oxymatrine in high-fat diet and streptozotocin-induced diabetic rats, Phytomedicine, 2014, 21, 807–814 CrossRef CAS PubMed.
- Y. Pan, C. Wang, Z. Chen, W. Li, G. Yuan and H. Chen, Physicochemical properties and antidiabetic effects of a polysaccharide from corn silk in high-fat diet and streptozotocin-induced diabetic mice, Carbohydr. Polym., 2017, 164, 370–378 CrossRef CAS PubMed.
- U. J. Jung, N. I. Baek, H. G. Chung, M. H. Bang, J. S. Yoo, T. S. Jeong, K. T. Lee, Y. J. Kang, M. K. Lee, H. J. Kim, J. Y. Yeo and M. S. Choi, The anti-diabetic effects of ethanol extract from two variants of Artemisia princeps Pampanini in C57BL/KsJ-db/db mice, Food Chem. Toxicol., 2007, 45, 2022–2029 CrossRef CAS PubMed.
- D. Wang, X. Zhao and Y. Liu, Hypoglycemic and hypolipidemic effects of a polysaccharide from flower buds of Lonicera japonica in streptozotocin-induced diabetic rats, Int. J. Biol. Macromol., 2017, 102, 396–404 CrossRef CAS PubMed.
- X. Zhang, Q. Lv, S. Jia, Y. Chen, C. Sun, X. Li and K. Chen, Effects of flavonoid-rich Chinese bayberry (Morella rubra Sieb. et Zucc.) fruit extract on regulating glucose and lipid metabolism in diabetic KK-Ay mice, Food Funct., 2016, 7, 3130–3140 CAS.
- T. Takayanagi, M. Makino, N. Fukami, T. Itoh, H. Sasaki, M. Kusaka, T. Kenmochi, K. Hoshinaga and M. Itoh, Utility of glucagon stimulation test in type 1 diabetes after pancreas transplantation, Transplant Proc., 2014, 46, 967–969 CrossRef PubMed.
- M. Fizelova, M. Miilunpohja, A. J. Kangas, P. Soininen, J. Kuusisto, M. Ala-Korpela, M. Laakso and A. Stancakova, Associations of multiple lipoprotein and apolipoprotein measures with worsening of glycemia and incident type 2 diabetes in 6607 non-diabetic Finnish men, Atherosclerosis, 2015, 240, 272–277 CrossRef CAS PubMed.
- M. J. Kruger, N. Davies, K. H. Myburghb and S. Lecourc, Proanthocyanidins, anthocyanins and cardiovascular diseases, Food Res. Int., 2014, 59, 41–52 CrossRef CAS.
- D. P. Mertz, Atherosclerosis-index (LDL/HDL): risk indicator in lipid metabolism disorders, Med. Klin., 1980, 75, 159–161 CAS.
- H. E. Khoo, A. Azlan, S. T. Tang and S. M. Lim, Anthocyanidins and anthocyanins: colored pigments as food, pharmaceutical ingredients, and the potential health benefits, Food Nutr. Res., 2017, 61, 1361779 CrossRef PubMed.
|
This journal is © The Royal Society of Chemistry 2018 |
Click here to see how this site uses Cookies. View our privacy policy here.