DOI:
10.1039/C7FO01409K
(Paper)
Food Funct., 2018,
9, 463-475
Aronia–citrus juice (polyphenol-rich juice) intake and elite triathlon training: a lipidomic approach using representative oxylipins in urine
Received
12th September 2017
, Accepted 21st November 2017
First published on 22nd November 2017
Abstract
In the present study, we examined whether particular urinary oxylipins (isoprostanes (IsoPs), leukotrienes (LTs), prostaglandins (PGs), and thromboxanes (TXs)) in 16 elite triathletes could alter during 145 days of training. Within this time span, 45 days were dedicated to examining the effects of the intake of a beverage rich in polyphenols (one serving: 200 mL per day) supplemented in their diet. The beverage was a mixture of citrus juice (95%) and Aronia melanocarpa juice (5%) (ACJ). Fifty-two oxylipins were analyzed in the urine. The quantification was carried out using solid-phase extraction, liquid chromatography coupled with triple quadrupole mass spectrometry. The physical activity decreased the excretion of some PG, IsoP, TX, and LT metabolites from arachidonic acid, γ-dihomo-linolenic acid, and eicosapentaenoic acid. The ACJ also reduced the excretion of 2,3-dinor-11β-PGF2α and 11-dehydro-TXB2, although the levels of other metabolites increased after juice supplementation (PGE2, 15-keto-15-F2t-IsoP, 20-OH-PGE2, LTE4, and 15-epi-15-E2t-IsoP), compared to the placebo. The metabolites that increased in abundance have been related to vascular homeostasis and smooth muscle function, suggesting a positive effect on the cardiovascular system. In conclusion, exercise influences mainly the decrease in oxidative stress and the inflammation status in elite triathletes, while ACJ supplementation has a potential benefit regarding the cardiovascular system that is connected in a synergistic manner with elite physical activity.
Introduction
Currently, it is not clear whether polyphenol supplementation exerts beneficial effects on oxidative stress (OS) and/or the inflammation status in the area of sports.1,2 Many studies analyzing the effects of dietary polyphenols on human health have been performed in the last decade, with increasing numbers of reports studying flavonoids and polyphenols in general.3,4 The studies on polyphenol supplementation in exercise includes mainly extracts, juices, infusions, or increased intake of polyphenol-rich foods (including functional foods).1 In athletes of different disciplines, polyphenols have shown antioxidant potential that can be beneficial for the reduction of the effects of oxidative damage during intense exercise, apparently without an anti-inflammatory effect.4 Furthermore, it is also necessary to take into account the effect of physical exercise, since this external factor has shown a positive effect on lipid peroxidation and/or OS as a consequence of its chronic practice.5–8 In 2005, Petersen9 mentioned that regular exercise induces an anti-inflammatory response rather than a pro-inflammatory response. Regular exercise training promotes increases in enzymatic and non-enzymatic antioxidants in muscle fibers, resulting in improved endogenous protection against exercise-mediated oxidative damage.10
In the field of sports science and elite sports environment, biomarkers are used to make inferences about the athlete's underlying physiology and health, particularly in the context of adaptation to training and the impact of environmental stressors.11 Metabolomics and lipidomics data indicate that intensive and prolonged exercise is associated with extensive lipid mobilization and oxidation, including many components in the pathway of linoleic acid conversion and related oxidized derivatives or oxylipins.12 The lipid metabolism constitutes a network of pathways that are related at multiple biosynthetic hubs.13 Oxygenated lipids are collectively known as oxylipins.14 Eicosanoids, a subset of oxylipins, are signaling molecules that have been used as biomarkers for a global picture of changes in lipid peroxidation and vascular events as a consequence of chronic exercise and the supplementation of polyphenols.5–8,12–15 Eicosanoids are a family that includes prostaglandins (PGs), leukotrienes (LTs), thromboxanes (TXs), and isoprostanes (IsoPs), which are lipid mediators involved in the physiopathology of all organs, tissues, and cells.16,17 PGs and TXs, collectively termed prostanoids, are formed when arachidonic acid (AA), a 20-carbon unsaturated fatty acid, is released from the plasma membrane by phospholipases and metabolized by the sequential actions of prostaglandin G/H synthase, or cyclooxygenase (COX). TXA2 is synthesized from prostaglandin H2 (PGH2) by thromboxane synthase, and it is non-enzymatically degraded into biologically inactive thromboxane B2 (TXB2).18 On the other hand, there are four primary bioactive PGs generated in vivo: prostaglandin E2 (PGE2), prostacyclin (PGI2), prostaglandin D2 (PGD2), and prostaglandin F2α (PGF2α).18 Besides AA, another polyunsaturated fatty acid (PUFA) is dihomo-γ-linolenic acid (DGLA), a 20-carbon n-6 (C20:3 n-6) derived in vivo from α-linolenic acid (c18:3 n-6). Through a series of free radical reactions, COX metabolizes DGLA and AA to form various bioactive metabolites: namely, the 1 and the 2 serie of PGs (PG1 and PG2), respectively.19 The LTs also contain 20 carbons, but lack the 5-carbon ring structure.20 They are AA metabolites derived from the action of 5-LOX (5-lipoxygenase). The immediate product of 5-LOX is LTA4 (leukotriene A4), which is enzymatically converted into either LTB4 (leukotriene B4), by LTA4 hydrolase, or LTC4 (leukotriene C4), by LTC4 synthase.20 The glutathione conjugate forms are termed cys-LTs (cysteinyl leukotrienes) and include leukotriene C4 (LTC4), leukotriene D4 (LTD4), and leukotriene E4 (LTE4). The cys-LTs are potent bronchoconstrictors and vasoconstrictors.13 The biosynthesis of eoxins (EX), structural isomers of cys-LTs, is initiated via the 15-lipoxygenase (15-LOX) pathway. Also, there is another pathway that occurs in vivo through a free radical-mediated mechanism to yield a series of PG-like compounds termed IsoPs, independent of the catalytic activity of COX.21,22 The F2-isoprostanes (F2-IsoPs) are an in vivo index of OS.16 Furthermore, F1-phytoprostanes (F1-PhytoPs) and F3-IsoPs are also generated from α-linolenic acid (ALA) and eicosapentaenoic acid (EPA).23,24 Finally, the 3-serie prostanoids, derived from the COX oxidation of EPA, may mediate the anti-inflammatory effects of this fatty acid.25
Based on the preceding discussion, the primary goal of this randomized, double-blind, placebo-controlled, and crossover study was to ascertain the effects of a serving (200 mL) of Aronia–citrus juice (ACJ) on the generation and metabolism of oxylipins, using a lipidomic approach. Also, the study design allowed the assessment of the changes produced by elite training sessions. We screened biomarkers from AA via LOX (LTs, cysLTs, and EXs), as well as other IsoPs, PGs, and TXs that complement our schematic of oxylipins (52 lipid mediators (Fig. 1)).
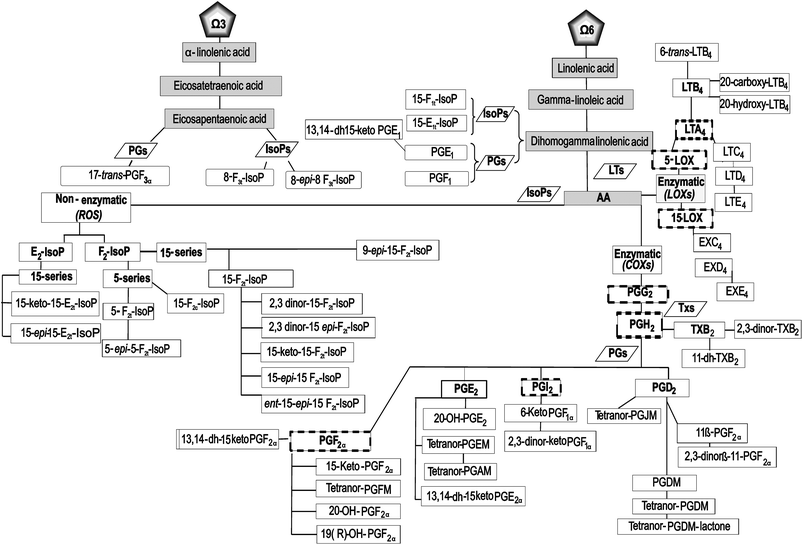 |
| Fig. 1 Flow chart: pathway of the oxylipins analyzed in this study. The nomenclature of the metabolites is described in the text. | |
Materials and methods
Physical characteristics of participants
The anthropometric measurements were made according to the methods of the International Society of Advancement of Kinanthropometry (ISAK),26 and all tests were performed by the same, internationally certified anthropometrist (Level 2 ISAK) with the objective of decreasing the technical errors of measurement. The body composition was determined by GREC Kinanthropometry consensus,27 using a model consisting of the determination of total fat by Withers’ formula,28 lean weight by using the procedure described by Lee et al.,29 and residual mass by the difference in the weight (Table 1).
Table 1 Physical and metabolic parameters and training loads of the triathletes
Physical characteristics |
C-B |
C–T |
Placebo |
ACJ |
CP-T |
Female |
Male |
Female |
Male |
Female |
Male |
Female |
Male |
Female |
Male |
Body mass index. Abbreviations: ACJ, Aronia–citrus juice; AS, abdominal skinfold; BS, biceps skinfold; CB, control baseline; CP-T, control post-treatment; CT, control training; FTS, front thigh skinfold; ICS, iliac crest skinfold; MCS, medial calf skinfold; SES, supraespinale skinfold; SS, subscapular skinfold; TS, triceps skinfold.
|
Years |
21.08 ± 3.0
|
19.0 ± 1.7 |
21.08 ± 3.0
|
19.0 ± 1.7 |
21.08 ± 3.0
|
19.0 ± 1.7 |
21.08 ± 3.0
|
19.4 ± 1.3 |
21.08 ± 3.0
|
19.6 ± 1.3 |
Weight (kg) |
54.8 ± 12.2
|
69 ± 6.2 |
54.8 ± 11.6
|
69 ± 6.4 |
56.2 ± 4.8
|
70.7 ± 6.9 |
54.4 ± 5.0
|
71.2 ± 4.6 |
53.1 ± 2.9
|
72.2 ± 6.8 |
Height (m) |
1.6 ± 0.1
|
1.8 ± 0.1 |
1.6 ± 0.1
|
1.8 ± 0.1 |
1.6 ± 0.1
|
1.8 ± 0.1 |
1.6 ± 0.1
|
1.8 ± 0.1 |
1.6 ± 0.1
|
1.8 ± 0.1 |
BMIa (kg m−2) |
21.2 ± 4.1
|
22.2 ± 1.0 |
21.2 ± 4.1
|
22.2 ± 1.0 |
20.7 ± 1.3
|
21.7 ± 1.4 |
21.6 ± 2.4
|
21.6 ± 1.3 |
20.5 ± 1.6
|
21.8 ± 1.7 |
Total fat (kg) |
8.7 ± 4.1
|
9.2 ± 2.8 |
8.9 ± 4.7
|
8.8 ± 2.6 |
9.2 ± 0.9
|
8.0 ± 1.7 |
7.5 ± 1.2
|
6.4 ± 2.8 |
7.3 ± 1.4
|
6.8 ± 1.2 |
Lean weight (kg) |
20.8 ± 3.6
|
31.4 ± 2.1 |
20.6 ± 2.7
|
30.5 ± 2.7 |
20.8 ± 2.4
|
31.6 ± 3.0 |
19.4 ± 2.8
|
33.8 ± 3.2 |
20.9 ± 2.0
|
32.4 ± 2.4 |
SS (mm) |
12.7 ± 6.7
|
9.6 ± 3.0 |
13.4 ± 8.2
|
9.5 ± 2.1 |
11.7 ± 2.5
|
9.1 ± 1.7 |
10.7 ± 1.9
|
8.6 ± 2.0 |
9.9 ± 2.8
|
8.6 ± 1.8 |
TS (mm) |
16.3 ± 2.3
|
8.9 ± 3.0 |
18.4 ± 3.8
|
9.7 ± 2.6 |
19.3 ± 5.4
|
8.7 ± 2.1 |
16.1 ± 4.6
|
7.4 ± 2.4 |
17.4 ± 4.6
|
7.3 ± 1.5 |
BS (mm) |
10.3 ± 2.8
|
5.4 ± 2.4 |
9.8 ± 3.2
|
4.7 ± 1.5 |
7.2 ± 0.4
|
4.1 ± 0.6 |
5.7 ± 1.0
|
4.5 ± 1.5 |
5.7 ± 1.3
|
3.7 ± 0.4 |
ICS (mm) |
19.7 ± 4.5
|
12.0 ± 2.6 |
17.1 ± 6.9
|
13.1 ± 4.1 |
20.9 ± 4.5
|
12.5 ± 4.2 |
17.3 ± 3.7
|
11.2 ± 3.4 |
13.7 ± 4.3
|
9.6 ± 2.5 |
SES (mm) |
14.3 ± 6.5
|
9.0 ± 2.6 |
14.4 ± 6.9
|
8.9 ± 2.8 |
15.0 ± 1.0
|
8.7 ± 2.5 |
12.8 ± 2.1
|
7.6 ± 1.9 |
11.6 ± 2.5
|
6.7 ± 1.4 |
AS (mm) |
23.1 ± 5.9
|
16.4 ± 8.0 |
23.6 ± 6.9
|
15.5 ± 6.8 |
24.5 ± 4.7
|
14.5 ± 5.9 |
21.3 ± 4.1
|
11.8 ± 5.2 |
17.9 ± 4.6
|
10.0 ± 3.7 |
FTS (mm) |
27.2 ± 5.2
|
14.9 ± 4.4 |
26.4 ± 5.0
|
14.0 ± 4.4 |
25.8 ± 3.6
|
11.5 ± 2.3 |
23.8 ± 12.5
|
10.1 ± 2.9 |
26.0 ± 5.4
|
10.0 ± 2.5 |
MCS (mm) |
14.8 ± 3.8
|
9.0 ± 3.0 |
13.9 ± 3.0
|
9.5 ± 3.1 |
15.7 ± 2.1
|
8.2 ± 2.1 |
12.5 ± 1.8
|
7.2 ± 2.3 |
14.4 ± 2.9
|
7.3 ± 1.8 |
Dietary intake
The calculation of the dietary parameters and caloric intake was accurately designed and overviewed during the experimental intervention by nutritionists, using specific software for the calculation (website: http://www.easydiet.es) and with the additional assistance of Spanish and USDA databases (http://www.bedca.net/ and http://www.nal.usda.gov/fnic/foodcomp/search/). The dietary assessment and planning were based on sports nutrition guidelines.30,31
Aronia–citrus juice and placebo beverage
The polyphenol-rich juice composition was based on a mixture of citrus juice (95%) with added Aronia melanocarpa juice (5%). This juice was developed on an industrial pilot scale (HERO Spain S.A., Alcantarilla, Murcia) with organoleptically-acceptable criteria to mimic the composition of the flavonoids of the original beverage developed by Gonzalez-Molina et al.32 The nutrient and caloric content of the ACJ that the triathletes consumed is summarized in Table 2, detailing the percentage contribution of the juice to the total diet.
Table 2 Dietary parameters: caloric intake of the triathletes during the study and nutritional composition of the Aronia–citrus juice (ACJ)
(A) |
Male triathletes |
Female triathletes |
Energy intake (kcal d−1) |
2820.0 ± 241.2 |
2072.6 ± 223.4 |
Carbohydrate (g d−1) |
326.1 ± 63.5 |
211.3 ± 43.9 |
Dietary fiber (g d−1) |
27.3 ± 7.4 |
15.5 ± 4.4 |
Sugar (g d−1) |
121.3 ± 33.9 |
80.5 ± 18.3 |
Proteins (g d−1) |
133.7 ± 12.9 |
83.5 ± 9.0 |
Total lipids (g d−1) |
113.7 ± 13.3 |
107.1 ± 14.4 |
SFAa (g d−1) |
33.5 ± 6.5 |
29.6 ± 4.4 |
MUFAb (g d−1) |
56.5 ± 5.5 |
56.6 ± 7.5 |
PUFAc (g d−1) |
16.9 ± 2.7 |
15.9 ± 6.7 |
Vitamin C (mg d−1) |
178.9 ± 71.9 |
135.0 ± 60.4 |
Vitamin A (μg d−1) |
2970.0 ± 913.9 |
1427.4 ± 573.1 |
Vitamin E (mg d−1) |
21.0 ± 5.6 |
13.9 ± 3.4 |
Vitamin D (mg d−1) |
988. ± 47.5 |
751.6 ± 163.0 |
Iron (mg d−1) |
20.9 ± 2.4 |
14.9 ± 2.6 |
Selenium (mg d−1) |
149.8 ± 21.5 |
103.0 ± 17.4 |
Water ingestion (mL d−1) |
1500d |
1500d |
(B) ACJ |
200 mL |
% |
(A) Dietary parameters and caloric intake of the triathletes during the study. Saturated fatty acids. Monounsaturated fatty acids. Polyunsaturated fatty acids. This was the daily water intake required; furthermore, the athletes drank extra liquids during the nutritional intervention (200 mL day−1 of ACJ or placebo), as well as during their sessions of training (400 mL to 600 mL hour−1 of water). (B) The nutritional composition of ACJ; %, contribution of the juice to the diet. The values of the phenolic content are mean ± standard deviation (n = 3), expressed as mg per 200 mL, and the phytochemical study of the juice was performed according to the procedure of Gonzalez-Molina (2008).32 |
Energy intake (kcal) |
76.0 |
2.6 |
Proteins (g) |
0.9 |
0.6 |
Carbohydrate (g) |
18.0 |
2.6 |
Sugar (g) |
6.6 |
5.2 |
Fat (g) |
0.1 |
0.1 |
|
Flavanones (mg) |
Eriocitrin |
22.9 ± 0.16 |
|
Hesperidin |
27.08 ± 0.28 |
|
|
Flavones (mg) |
Vicenin-2 |
1.18 ± 0.04 |
|
Diosmetin-6,8-di-O-glucoside |
15.5 ± 0.38 |
|
Diosmin |
<0.5 |
|
|
Anthocyanins (mg) |
Cyanidin 3-O-galactoside |
30.16 ± 0.20 |
|
Cyanidin 3-O-glucoside |
2.62 ± 0.04 |
|
Cyanidin 3-O-arabinoside |
18.36 ± 0.40 |
|
Cyanidin 3-O-xyloside |
2.22 ± 0.03 |
|
Total anthocyanins |
53.4 ± 0.70 |
|
|
Hydroxycinnamic acids (mg) |
Neochlorogenic acid |
39.44 ± 0.34 |
|
Chlorogenic acid |
29.38 ± 0.26 |
|
|
∑Quercetin derivatives (mg) |
8.62 ± 0.26 |
|
The placebo beverage composition was based on a mixture of water, authorized red dye, flavoring, and sweetener, possessing sensory characteristics close to those of ACJ (see Garcia-Flores et al.33,34 for further information about ACJ composition and nutritional planning).
Training load
Training load quantification was performed using the Objective Load Scale (ECOs) developed by Cejuela-Anta and Esteve-Lanao.35 The training loads designed for the triathletes in the present work were similar to those found in other studies.5,30,33,36 The method used in the current work allowed the quantification of the training loads in triathlon (swim, bike, run, and transitions).37 The values of daily and weekly training were determined and summarized to assess the ECOs of each volunteer, depending on their physical characteristics and the intensity of the training program (the ECOs data presented in this work are the average of the individual ECOs of the triathletes). The variations of the ECOs are shown in Fig. 2 for better orientation.
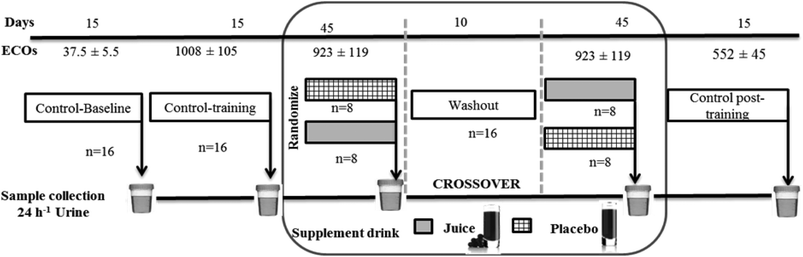 |
| Fig. 2 Study design: this crossover study was randomized, double-blind, and placebo-controlled. Sixteen athletes, randomly divided into two groups, were assigned to supplementation of either 200 mL of ACJ or 200 mL of placebo. After 45 days of supplementation and a 10-day washout period, the beverages were reversed. Three controls were used: baseline control, control training, and control post-training with a duration of 15 days. Urine samples were collected at the end of each stage. The training load quantification was performed using the Objective Load Scale (ECOs).5,33,36 | |
Study design
Sixteen triathletes (6 training women and 10 training men) from the University of Alicante (Spain) agreed to participate in the project. An elite athlete in the context of sports medicine is an athlete with potential for competing in the Olympics or as a professional athlete.38 The volunteers were non-smokers, had stable food habits, and did not receive any medication (the specific absence of acute administration of anti-inflammatory drugs) during the experimental procedure. The study was approved by the Bioethics Committee of the University Hospital of Murcia and was in accordance with the Declaration of Helsinki. All participants provided written informed consent to a protocol approved by the institution.39 The recruitment started on 28th–29th October 2010 and was completed on 24th–25th March 2011. This study had a randomized, double-blind, placebo-controlled, and crossover design (Fig. 2) and was previously approved by nutritional experts. We assumed an equal allocation of volunteers to each beverage using computer-generated simple randomization with consecutive codes linked to the preparation of the placebo or ACJ. An impartial outsider, without the knowledge of the study, helped us to select the randomization code and indicated the assignment order. The volunteers remained blinded throughout the study as well as the researchers responsible for the outcome measurements and the data analysis (see Garcia-Flores et al.33,34 for further information).
Urine sample collection and preparation
Twenty-four-hour urine samples were collected at the end of each stage (C-B: control baseline, C-T: control training, P: placebo intake stage, ACJ: Aronia–citrus juice intake stage, and CP-T: control post-training). All samples collected were immediately frozen (−80 °C) to preserve the sample integrity until the time of analysis.
Chemicals and analytes
Seven IsoPs derived from AA (15-F2t-IsoP, 15-keto-15-F2t-IsoP, 15-epi-15-F2t-IsoP, 2,3-dinor-15-F2t-IsoP, ent-15-epi-15-F2t-IsoP, 9-epi-15-F2t-IsoP, and 15-keto-15-E2t-IsoP); 31 enzymatic metabolites of AA (PGD2, PGDM (PGD metabolite), tetranor-PGDM lactone (tetranor-PGD metabolite lactone), 11-β-PGF2α, 2,3-dinor-11-β-PGF2α, tetranor-PGJM (tetranor-PGJ metabolite), tetranor-PGDM (tetranor-PGD metabolite), 6-keto-PGF1α, PGE2, 20-OH-PGE2, tetranor-PGEM (tetranor-PGE metabolite), tetranor-PGAM (tetranor-PGA metabolite), 13,14-dihydro-15-keto-PGE1, 13,14-dihydro-15-keto-PGE2, 13,14-dihydro-15-keto-PGF2α, PGF2α, tetranor-PGFM (tetranor-PGF metabolite), 20-OH-PGF2α, 19(R)-OH-PGF2α, 15-keto-PGF2α, thromboxane B2 (TXB2), 2,3-dinor-TXB2, 11-dehydro-thromboxane B2 (11-dh-TXB2), leukotriene (LT) B4, 20-carboxy-LTB4, 20-hydroxy-LTB4, 6-trans-LTB4, LTC4, LTE4, EXC4, and EXE4); four metabolites of DGLA (PGE1, PGF1α, 15-F1t-IsoP, and 15-E1t-IsoP); and one metabolite of EPA (17-trans-PGF3α) were purchased from Cayman Chemicals (Ann Arbor, MI, USA). The authentic markers [2H4]-13,14-dihydro-15-keto-PGE1, [2H4]-13,14-dihydro-15-keto-PGE2, [2H4]-13,14-dihydro-15-keto-PGF2α, [2H4]-6-keto-PGF1α, [2H4]-TXB2, [2H4]-20-carboxy-LTB4, [2H4]-LTB4, and [2H4]-8,12-iso-iPF2α-VI were also purchased from Cayman Chemicals.
Four IsoPs derived from AA (15-epi-15-E2t-IsoP, 2,3-dinor-15-epi-15-F2t-IsoP, 5-F2t-IsoP, and 5-epi-5-F2t-IsoP) and two metabolites of EPA (8-F3t-IsoPs and 8-epi-8-F3t-IsoPs) were synthesized according to our published procedures,40–44 while 2,3-dinor-6-keto-PGF1α, [2H3]-2,3-dinor-6-keto-PGF1α, EXD4, 15-F2c-IsoPs, and [2H4]-15-F2c-IsoPs were provided as described by Balgoma et al. (2013).45 The enzyme β-glucuronidase, type H2 from Helix pomatia, and BIS–TRIS (bis-(2-hydroxyethyl)-amino-tris(hydroxymethyl)-methane) were obtained from Sigma-Aldrich (St Louis, MO, USA). All LC-MS grade solvents were obtained from J.T. Baker (Phillipsburg, NJ, USA). The Strata X-AW, 100 mg per 3 mL SPE cartridges were purchased from Phenomenex (Torrance, CA, USA). Ammonium acetate, methoxyamine hydrochloride, and isopropanol were purchased from Sigma-Aldrich. Milli-Q ultrapure deionized water was used (Millipore Corporation, Billerica, MA). Methanol and acetonitrile were obtained from Rathburn (Walkerburn, Scotland, UK). Acetone, acetic acid, and formic acid were purchased from Fisher. Aqueous ammonia (25%, w/v) was obtained from Merck (Darmstadt, Germany).
UHPLC-MS/MS analyses
The samples were analyzed according to two methods described previously by Medina et al.46 and Balgoma et al.45 for the purpose of a deeper analysis of the generation and metabolism of oxylipins by our volunteers.
UHPLC-QqQ-MS/MS for thirty-seven metabolites
The separation of the metabolites present in the urine was performed using a UHPLC system coupled with a 6460 QqQ-MS/MS system (Agilent Technologies, Waldbronn, Germany), using the setup described by Medina et al.46 The main changes are as follows: after being clarified with MeOH/HCl (200 mM), the urine samples were centrifuged at 10
000 rpm for 5 min. The solid-phase extraction was as follows: (1) preconditioning of the cartridge with MeOH (2 mL) and then MilliQ water (2 mL); (2) loading of the urine sample; (3) washing of the cartridge with MilliQ water (4 mL); and (4) elution of the cartridge with MeOH (1 mL). Subsequently, the MeOH was evaporated from the extract by using a SpeedVac concentrator and the extract was reconstituted in 200 μL of the mobile phase (A
:
B) (90
:
10). The changes in the identification and quantification of the metabolites were as follows: chromatographic separation was carried out on an ACQUITY UPLC BEH C18 column (2.1 × 150 mm, 1.7 μm; Waters), the column temperatures being 6 °C (left) and 6 °C (right). The flow rate was 0.15 mL min−1, using the linear gradient scheme (t, %B): (0.00; 60), (7.00; 60), (7.01; 73), (10.00; 73), (10.01; 80), (18.00; 100), (19.00; 100), and (19.01; 60). The operating conditions for the MS parameters were as follows: gas flow: 8 L min−1, nebulizer: 30 psi, capillary voltage: 4000 V, nozzle voltage: 2750 V, gas temperature: 325 °C, and jet stream gas flow: 8 L min−1. The MS parameters were in the range of 50 to 160 V and the collision energy was in the range of 0 to 24 V. The acquisition time was 19.01 min for each sample, with a post-run of 3.0 min for the column equilibration. The quantification of the oxylipins was carried out by daily preparation of calibration curves (concentration range 3.9 nM to 1 μM) using standard solutions. The matrix effect, recovery of extraction, and overall process efficiency for each analyte were assessed using post-extraction addition, established by Matuszewski et al.47 The values were within the requested range for all the metabolites.
The sensitivity, precision, and accuracy were established with the same parameters provided by the Guidance for Industry-Bioanalytic Method Validation (the intraday and interday values were in the range of 80–120% for all the metabolites).48 The metabolites determined by using this method were: PGDM, PGD2, tetranor-PGDM lactone, 11-β-PGF2α, 2,3-dinor-11β-PGF2α, tetranor-PGDM, tetranor-PGJM, PGE2, 20-OH-PGE2, tetranor-PGEM, tetranor-PGFM, 15-keto-PGF2α, 20-OH-PGF2α, 19 (R)-OH-PGF2α, 2,3-dinor-6-keto-PGF1a, 6-keto-PGF1a, 15-F2t-IsoP, 15-keto-15-F2t-IsoP, 15-epi-15F2t-IsoP, 2,3-dinor-15-F2t-IsoP, ent-15-epi-15F2t-IsoP, 9-epi-15-F2t-IsoP, 2,3-dinor-15-epi-15F2t, 5-F2t-IsoP, 5-epi-5F2t-IsoP, 15-keto-15E2t-IsoP, 15-epi-15E2t-IsoP, 11-dh-TXB2, 17-trans-PGF3α, 8-F3t-IsoP, 8-epi-8-F3t-IsoP, PGE1, PGF1α, 15-E1t-IsoP, and 15-F1t-IsoP. The quantification of the IsoPs, PGs, and TXs detected was performed using authentic markers. Data acquisition and processing were performed using the MassHunter software version B.04.00 (Agilent Technologies).
UHPLC-TQ-MS/MS for sixteen metabolites
For the remaining 16 lipid metabolites (LTs, PGs, TXs, and IsoPs), two different analytical methods based on Balgoma et al.45 were employed, using the same analytical platform: ACQUITY UPLC coupled with a Xevo TQS mass spectrometry system (Waters, Milford, MA) (LC-MS/MS).
Statistical analysis
The metabolites were analyzed individually as well as by serie or family, using the excretion values (μg per 24 h) obtained throughout the study (C-B, C-T, placebo stage, ACJ stage, and CP-T). The 24 h urine was used for the absolute calculation of the amount of the LTs, EXs, IsoPs, PGs, and TXs excreted; the volume of urine excreted by the volunteers was 1212.42 ± 716.50 mL per 24 h, on average, over the assay. The data shown are the mean ± SD (Table 3), as well as the quartiles (upper values 75%, median 50%, and lower values 25%) (Fig. 3). We employed non-parametric statistical tests since the data did not satisfy the assumption of normality. The Friedman test was used; if the P-value was significant, the post hoc Wilcoxon signed-rank test was used to decide which groups were significantly different from each other. The Bonferroni correction was applied; this correction was calculated by dividing the P-value (P = 0.05) by the number of tests, namely 10 (if the metabolite was detected in all the stages). Thus, our results were adjusted to P ≤ 0.005. The statistical analyses were made using the SPSS 23.0 software package (LEAD Technologies, Inc., Chicago, USA). The graphs were plotted using the SigmaPlot 12.0 software package (Systat Software, Inc., SigmaPlot for Windows).
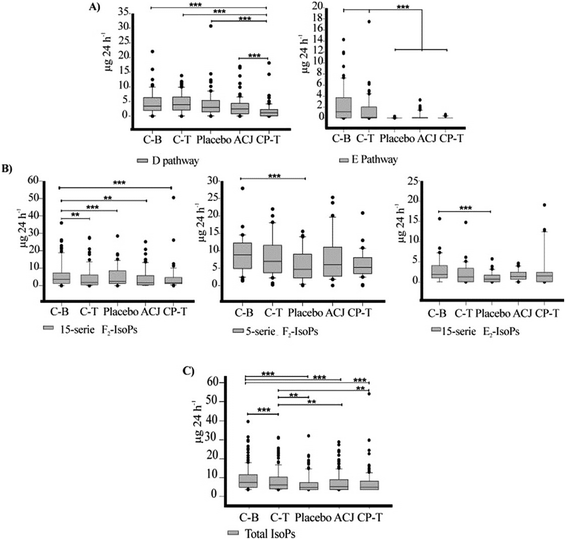 |
| Fig. 3 Box plots with quartiles (upper values 75%, median 50%, and lower values 25%) of the urinary oxylipins throughout the study (μg per 24 h). The level of statistical significance was set at P < 0.005 with the Bonferroni correction (** = P < 0.005 and *** = P < 0.001). (A) Prostaglandins by family, (B) isoprostanes by serie, and (C) total isoprostanes, both F2-isoprostanes and E2-isoprostanes. | |
Table 3 Urinary isoprostanes and prostaglandins (μg per 24 h) from arachidonic acid, dihomo-γ-linoleic acid, and eicosapentaenoic acid detected in the urine samples of triathletes
|
Analyte (μg per 24 h) |
Stage of study |
Friedman test |
C-B |
C-T |
P |
ACJ |
CP-T |
Mean |
SD |
Mean |
SD |
Mean |
SD |
Mean |
SD |
Mean |
SD |
χ
2
|
df |
Sig |
The data are shown as mean ± standard deviation (SD) in μg per 24 h. The volume of urine excreted by the volunteers was 1212.42 ± 716.50 mL per 24 h, on average, in all the periods. The statistical P-value from the Friedman test is indicated in italics and bold letters show the significant P-values. The mean values with letters in superscript were found in a reduced number of volunteers within the experimental groups; thus the number of volunteers was a = 2, b = 3, c = 4, d = 5, e = 6, and f = 7. Abbreviations: C-B: control baseline, C-T: control training, P: placebo, ACJ: Aronia–citrus juice, CP-T: control post-treatment. |
Arachidonic acid
|
PGs |
D pathway |
PGDM |
31.1
|
24.6 |
41.2
|
24.4 |
16.1
|
14.0 |
19.3
|
14.3 |
10.0
|
13.9 |
19.7
|
4
|
0.001
|
Tetranor-PGDM lactone |
2.4
|
2.4 |
1.1
|
1.2 |
1.2
|
0.6 |
0.9
|
1.1 |
1.4
|
1.7 |
3.8
|
4
|
0.430
|
11-β-PGF2α |
4.3
|
2.1 |
7.5
|
4.1 |
7.1
|
3.9 |
7.4
|
5.2 |
1.7
|
4.1 |
18.8
|
4
|
0.001
|
2,3-dinor-11β-PGF2α |
8.9
|
5.5 |
6.2
|
2.0 |
6.8
|
7.3 |
2.8
|
2.0 |
4.3
|
5.2 |
20.9
|
4
|
<0.001
|
Tetranor-PGDM |
3.2
|
2.5 |
3.8
|
2.7 |
2.7
|
1.6 |
2.0
|
1.2 |
0.6
|
0.7 |
21.3
|
4
|
<0.001
|
E pathway |
PGE2 |
0.51
|
0.50 |
0.15
|
0.14 |
0.04
|
0.08 |
0.19
|
0.30 |
0.44
|
0.08 |
13.5
|
4
|
0.009
|
20-OH-PGE2 |
3.8
|
4.6 |
2.0
|
2.1 |
2.1
b
|
0.6 |
0.9
|
1.0 |
4.3
c
|
3.1 |
4.2
|
2
|
0.122
|
Tetranor-PGEM |
2.6
|
2.2 |
1.2
|
1.7 |
0.9
c
|
0.9 |
2.4
d
|
1.6 |
3.0
c
|
2.6 |
— |
— |
— |
Tetranor-PGAM |
2.9
|
3.3 |
2.4
|
4.5 |
2.3
d
|
1.9 |
1.3
e
|
0.8 |
2.1
d
|
1.7 |
— |
— |
— |
13,14-dihydro-15-keto-PGF2α |
— |
— |
2.9
|
— |
6.1
|
— |
— |
— |
— |
— |
— |
— |
— |
13,14-dihydro-15-keto-PGE2α |
— |
— |
— |
— |
— |
— |
— |
— |
0.2
a
|
0.2 |
— |
— |
— |
F pathway |
Tetranor-PGFM |
0.9
|
0.4 |
1.8
|
— |
1.6
|
— |
— |
— |
— |
— |
— |
— |
|
PGF2α |
3.5
b
|
1.6 |
2.7
|
— |
2.7
|
— |
5.1
b
|
2.5 |
3.7
|
— |
— |
— |
— |
I pathway |
2,3-dinor-6-keto-PGF1α |
2.1
|
2.7 |
2.2
|
2.4 |
2.0
|
1.9 |
2.2
|
3.0 |
1.9
|
2.3 |
2.3
|
4
|
0.680
|
F2-Isoprostane |
15-serie |
15-F2t-IsoP |
3.2
|
0.7 |
2.7
|
0.5 |
2.5
|
0.5 |
2.1
|
0.6 |
1.6
|
0.4 |
16.1
|
4
|
0.002
|
15-keto-15-F2t-IsoP |
1.4
|
1.4 |
0.4
|
1.0 |
1.0
|
— |
0.2
|
0.4 |
3.02
d
|
1.9 |
6.1
|
2
|
0.046
|
15-epi-15-F2t-IsoP |
4.3
|
4.3 |
2.8
|
2.7 |
1.5
|
1.3 |
3.1
|
6.2 |
1.0
|
0.8 |
4.8
|
4
|
0.298
|
2,3-dinor-15-F2t-IsoP |
16.5
|
9.4 |
14.8
|
6.5 |
11.4
|
7.4 |
9.5
|
5.6 |
10.2
|
12.7 |
8.3
|
4
|
0.081
|
ent-15-epi-15-F2t-IsoP |
0.7
|
1.0 |
0.4
|
0.5 |
0.1
|
0.1 |
0.3
|
0.5 |
0.1
|
0.1 |
4.9
|
4
|
0.297
|
9-epi-15-F2t-IsoP |
2.7
|
1.6 |
1.4
|
0.8 |
1.0
|
0.4 |
1.3
|
0.9 |
1.2
|
0.8 |
15.1
|
4
|
0.004
|
2,3-dinor-15-epi-15-F2t-IsoP |
3.0
|
2.2 |
1.4
|
0.5 |
1.3
|
1.4 |
1.2
|
0.5 |
1.5
|
1.4 |
9.1
|
4
|
0.057
|
15-F2c-IsoPs |
8.4
|
4.3 |
8.2
|
4.9 |
6.4
|
2.9 |
7.0
|
3.7 |
5.3
|
3.3 |
5.4
|
4
|
0.250
|
5-serie |
5-F2t-IsoP |
11.2
|
5.6 |
10.7
|
5.8 |
9.0
|
4.3 |
11.9
|
6.8 |
7.5
|
4.7 |
4.5
|
4
|
0.332
|
5-epi-5F2t-IsoP |
7.2
|
4.6 |
5.5
|
4.5 |
2.9
|
2.0 |
4.7
|
3.4 |
4.9
|
2.5 |
13.3
|
4
|
0.010
|
E2-Isoprostane |
15-serie |
15-Keto-15-E2t-IsoP |
3.3
|
0.5 |
2.3
|
0.4 |
1.7
|
0.3 |
1.9
|
0.2 |
2.1
|
0.6 |
8.5
|
4
|
0.073
|
15-epi-15-E2t-IsoP |
2.7
|
4.1 |
2.1
|
3.8 |
2.0
b
|
1.6 |
1.3
|
1.5 |
3.5
|
6.1 |
1.0
|
3
|
0.785
|
LT |
LTB4 |
0.03
|
0.02 |
0.02
|
0.02 |
0.03
|
0.02 |
0.06
|
0.04 |
0.03
|
0.02 |
9.7
|
4
|
0.040
|
cys-LT |
LTE4 |
0.13
|
0.07 |
0.11
|
0.09 |
0.06
|
0.03 |
0.12
|
0.11 |
0.05
|
0.05 |
9.9
|
4
|
0.040
|
EX |
EXD4 |
— |
— |
2.1
b
|
2.6 |
0.1
|
— |
0.2
|
— |
— |
— |
— |
— |
— |
TXs |
TXB2 |
— |
— |
— |
— |
|
— |
0.1
|
— |
0.1
|
— |
|
— |
— |
11-dehydro-TXB2 |
0.3
|
0.2 |
0.5
|
0.2 |
0.3
|
0.2 |
0.2
|
0.1 |
0.2
|
0.1 |
21.8
|
4
|
<0.001
|
2,3-dinor-TXB2 |
3.3
f
|
0.7 |
3.1
e
|
0.5 |
2.9
f
|
0.4 |
2.1
e
|
0.5 |
2.4
d
|
1.0 |
— |
— |
— |
|
Eicosapentaenoic acid
|
PG |
17-trans-PGF3α |
1.1
|
1.7 |
1.2
|
1.7 |
0.7
|
1.0 |
0.2
|
0.4 |
2.9
b
|
2.8 |
1.52
|
3
|
0.676
|
IsoP |
8-F3t-IsoP |
3.2
|
2.3 |
0.6
a
|
0.1 |
1.0
b
|
0.4 |
1.6
d
|
1.0 |
—
|
—
|
|
|
|
|
Dihomo-γ-linolenic acid
|
PGs |
PGE1 |
0.3
|
0.2 |
0.6
|
0.3 |
0.5
|
0.3 |
0.4
|
0.2 |
0.1
|
0.1 |
29.6
|
4
|
<0.00
|
PGF1α |
2.1
f
|
0.4 |
0.05
|
— |
3.8
b
|
2.6 |
— |
— |
1.1
|
— |
— |
— |
— |
IsoP |
15-E1t-IsoP |
0.5
|
0.1 |
—
|
—
|
—
|
—
|
0.3
a
|
0.3 |
— |
— |
— |
— |
— |
Results and discussion
Currently, the evidence is insufficient to make recommendations for the use of polyphenol supplementation by elite athletes.1,4,49,50 So, we wanted to make an in-depth examination of the primary lipid peroxidation biomarkers using a study design which allows the observation of the effects of physical exercise and polyphenolic-rich beverage intake. A total of 52 oxylipins were screened in the triathletes’ urine (Table 3). The mass spectral information of the oxylipins identified was based on Medina et al.46 and Balgoma et al.45 In total, 37 metabolites – 17 PGs, 14 IsoPs, two LTs, one EX, and three TXs – were detected in the urine samples of the triathletes. Therefore, 15 metabolites (PGD2, tetranor-PGJM, 6-keto-PGF1α, 20-OH-PGF2α, 19(R)-OH-PGF2α, 15-keto-PGF2α, 15-F1t-IsoP, 8-epi-8-F3t-Isop, LTC4, EXC4, EXE4, 6-trans-LTB4, 20-carboxy-LTB4, 20-hydroxy-LTB4, and 13, 14-dihydro-15-keto-PGE1) were not detected.
Prostaglandin and thromboxane metabolites derived from arachidonic acid
Recent publications have demonstrated changes in lipid peroxidation as a consequence of chronic exercise.5–8 A previous study by our group showed a decrease in the values of urinary PGs (tetranor-PGEM and 11-β-PGF2α) after a chronic training program.5 Our current results are similar, showing a decline of these biomarkers due to the elite training program. In our urine samples, 17 PGs from different families were quantified. Our data show means in the range from 0.04 ± 0.08 μg per 24 h (PGE2) to 41.2 ± 24.4 μg per 24 h (PGDM). The PGs are potent oxylipins involved in numerous homeostatic biological functions and inflammation.18 The literature mentions that regular exercise induces an anti-inflammatory response rather than a pro-inflammatory response.4,9 In this context, the results for the concentrations of metabolites from the PGD2 pathway are notable since they have been implicated in both the development and resolution of inflammation. For the PGD2 pathway, the Friedman test revealed statistically significant differences (χ2 (4) = 42.143, P < 0.001). The CP-T value was significantly lower, compared to all other stages (Fig. 3A). Moreover, without the Bonferroni correction, the ACJ stage was different from C-T (Z = −2.155, P = 0.031). Considering the PGD2 metabolites individually, PGDM was the metabolite that showed the highest excretion levels. Prostaglandin D2 is a COX product of AA that activates D prostanoid receptors to modulate vascular, platelet, and leukocyte function in vitro.51 The Friedman test revealed statistically significant changes (Table 3) in this metabolite; the Wilcoxon test showed that the CP-T value was lower than that for C-T (Z = −3.237, P < 0.001). The 11-β-PGF2α content in the CP-T stage was significantly lower than that in all other stages (CB, Z = −3.124, P = 0.002; C-T, Z = −3.124, P < 0.001; placebo, Z = −3.237, P = 0.001; and ACJ, Z = −3.067, P = 0.002). The 2,3-dinor-11-β-PGF2α excretion in the ACJ stage was lower than that for C-B (Z = −2.953, P = 0.003) and C-T (Z = −3.124, P = 0.002). The ACJ stage also showed a lower value of this compound compared to the placebo stage, though this was not statistically significant when applying the correction (P = 0.009). In the last control stage, the excretion of tetranor-PGDM was decreased when compared to C-T (Z = −3.010, P = 0.003), placebo (Z = −3.233, P = 0.001), and ACJ (Z = −2.856, P = 0.004) (Table 3). According to research carried out by Morrow et al.,52 PGDM is a major urinary metabolite of PGD2 with a unique lower side-chain that readily undergoes reversible cyclization. In our study, the urinary excretion of PGDM was highest under basal conditions, but showed a decrease of about 70% by the end of the experiment. This suggests that in our triathletes there was a reduction in the inflammation status since the hallmark of inflammation is the enhanced secretion of pro-inflammatory immune mediators such as PGs.49,53 A study in humans using liquid chromatography-tandem mass spectrometry mentioned that tetranor-PGDM was much more abundant than the PGD2 metabolites 11β-PGF2α and 2,3-dinor-11β-PGF2α in the urine of healthy volunteers.51 In our elite triathletes, 11β-PGF2α and 2,3-dinor-11β-PGF2α (F-ring metabolites) were much more abundant than tetranor-PGDM (D-ring metabolite). This leads us to believe that physical exercise affects quantitatively the excretion of metabolites of this PGD pathway, when compared to non-athlete volunteers. Regarding the effect of ACJ intake on the excretion of PGD2 metabolites, we observed a positive influence, since 2,3-dinor-11β-PGF2α showed a significant decrease when compared to the first controls; also, the excretion of PGDM showed a significant reduction (in the placebo stage, it remained constant). Previous studies, both in vivo and in vitro, have also reported some influence on the cardiovascular system due to polyphenol supplementation in the diet.1,13,47 In addition, a study by our group analyzed the biomarker implicated in iron metabolism, hepcidin, and revealed that long-term training using ECOs reduces inflammation and, hence, could be responsible for the decrease of hepcidin in triathletes found in this study.54
The concentration of the metabolites from the PGE pathway showed a significant decrease after increased training, suggesting that physical exercise also played a role in the decline in the excretion of these metabolites. The levels of the metabolites of the PGE2 pathway in C-B and C-T were higher, but subsequently declined (χ2 (4) = 21.962, P = 0.001) (Fig. 3A). Also, we cannot rule out an effect of ACJ intake on inflammation since the excretion of PGE2 (detected in all the periods) increased in comparison with the placebo stage (0.04 ± 0.08 vs. 0.19 ± 0.30). The placebo period showed lower values than C-B (Z = −2.98, P = 0.003) and C-T (Z = −3.180, P = 0.001), although the excretion values did not decrease significantly between C-B and C-T (Z = −2.669, P = 0.008). The other three metabolites of the E pathway (20-OH-PGE2, tetranor-PGEM, and tetranor-PGAM) were mainly detected in the two control periods (C-B and C-T), but in the beverage intake stages and the CP-T stage, the number of volunteers that excreted these biomarkers decreased. 20-OH-PGE2 was excreted by the majority of the volunteers after the juice intake, compared to the placebo. PGE2 is involved in all processes leading to the classic signs of inflammation (redness, swelling, and pain), but also shows anti-inflammatory properties.18 For example, according to recent in vivo studies, this lipid mediator is related to numerous physiological and pathophysiological processes in the kidney,55 indicating a significant role in modulating the effect of vasopressin on the osmotic water reabsorption in the renal collecting duct cells – where it attenuates antidiuretic action.56 In addition, it has been mentioned that the induction of prostanoids during exercise alters clotting factors, increases vascular tone, and helps adapt muscle cells to contractile activity.57 Based on the above, our results suggest a potential effect of ACJ intake on the inflammatory process and vascular system.
Regarding the F and I pathways, the metabolites were scarcely detected in the urine samples or did not differ significantly during the study. Regarding the TXs, the primary enzymatic metabolite of TXA2 is 11-dehydro-TXB2 (11-dh-TXB2), which has been validated as a reliable and noninvasive biomarker-integrated index of in vivo platelet activation.58 A previous report observed that 22 sedentary volunteers subjected to standardized, aerobic, high-amount–high-intensity training for eight weeks showed significant decreases in the urinary excretion of 11-dh-TXB2.59 The authors related this result to platelet activation and hence it may be relevant to explain why long-term physical exercise is beneficial for the cardiovascular system. According to our results, the excretion of 11-dh-TxB2 showed a significant decrease in the ACJ (Z = −2.953, P = 0.003) and CP-T (Z = −3.069, P = 0.002) stages, compared to C-T (Table 3). The 11-dh-TXB2 decreased significantly in the last period when the training load was lower; ACJ also had a considerable influence, reducing the values, suggesting a cardiovascular benefit.
Leukotrienes
Two metabolites (LTB4 and LTE4) were detected in all the stages and in the majority of the volunteers. The Friedman test showed significant changes in LTB4 and the subsequent Wilcoxon signed-rank test revealed higher values in the ACJ stage compared with the placebo (Z = −2.166, P = 0.03), C-T (Z = −2.668, P = 0.008), and CP-T (Z = −2.166, P = 0.03) stages. However, no P-value was below 0.005. In contrast, LTE4 showed a significant decrease in the placebo stage, relative to the baseline values (Z = −2.784, P = 0.005). Also, the placebo stage differed from the ACJ stage (Z = −1.960, P = 0.05), but not significantly so after the Bonferroni correction. The excretion values of the CP-T stage were lower than those for C-B (Z = −2.668, P = 0.008) and C-T (Z = −1.931, P = 0.053), but not statistically so (Table 3). In summary, the urinary metabolites LTB4 and LTE4 showed significant changes; in particular, the ACJ stage presented higher values than the placebo phase. These findings are the opposite of those mentioned in the current literature, since most polyphenol intake studies have shown decreased excretion in healthy people.50,60 It has been demonstrated that flavonoids can modulate the activity of enzymes that are involved in the metabolism of AA in macrophages – such as phospholipase A2, COXs, and LOXs; the inhibition of these enzymes by flavonoids lowers the production of the mediators of inflammatory reactions.60 Yoon and Baek (2005)61 also mentioned that polyphenols are inhibitors of both COX and LOX and that a general rule is “more COX inhibitions and less LOX inhibitions with polyphenols that contain few hydroxyl substituents (with none in ring B)”. This suggests that polyphenols, including those in our juice rich in polyphenols, have more effect on an inflammatory cascade of COX-2, which allows the LOX branch to accelerate the formation of LTs. This explanation seems to describe to a certain extent the change produced in the excretion values in our study. On the other hand, due to the decline in the ECOs load, a decrease in the excretion of LTE4 was detected. Other reports have mentioned that elite athletes show an increased risk of respiratory symptoms related to asthma, especially those that participate in endurance sports – such as swimming, running, and cycling – and in winter sports. This risk to the respiratory system arises because, during physical activity, the elite athletes increase their water and heat loss through respiration.62 This has strong ties with the results of LTs since they play a key role in perpetuating airway inflammation – leading directly to airflow obstruction through the effects on vascular permeability, mucus production, and smooth muscle constriction.63 A training program can result in a depletion of LTs and/or a slow cys-LTs response to exercise, which may be responsible for the protective effect of training programs on respiratory symptoms.64 Our study shows that post-training could change the excretion of cys-LTs, and therefore might have an effect on the airway pathway.
Isoprostanes derived from arachidonic acid
The measurement of F2-IsoPs is known to be an index of OS in vivo.16 Regarding the level of total IsoPs derived from AA in urine, a significant reduction was observed, reflecting mainly the OS decrease in the CP-T stage (Fig. 3C). When the sum of all the IsoPs was submitted to the Friedman test, a significant P-value (χ2 (4) = 91.035, P ≤ 0.001) was obtained. The total IsoPs ranged from 6.10 ± 6.47 μg per 24 h (C-B) to 3.42 ± 5.9 μg per 24 h (CP-T). The Wilcoxon signed-rank test showed a tendency of the excretion to decline over the course of the study (Fig. 3C). The IsoPs showed significant variation in their urinary excretion when the values were analyzed by series: 15-F2t-IsoPs (χ2 (4) = 33.360, P ≤ 0.001), 5-F2t-IsoPs (χ2 (4) = 12.893, P = 0.012), and 15-E2-IsoPs (χ2 (4) = 14.484, P = 0.006) (Fig. 3B).
These data suggest that chronic exercise decreased the OS levels in our elite athletes. According to the review by Nikolaidis et al.,65 in most of the cases in which they analyzed this behavior, the levels of urinary F2-IsoP were decreased by chronic exercise. In other studies,5,66,67 physical activity was also the primary factor that decreased the urinary OS biomarker (IsoPs). The literature mentions that regular exercise training increases the levels of enzymatic and non-enzymatic antioxidants in muscle fibers, resulting in improved endogenous protection against exercise-mediated oxidative damage.10 Furthermore, in athletes of different disciplines, polyphenols have shown antioxidant potential that can be beneficial in the reduction of the effects of oxidative damage during intense exercise.4 In our study, considering the metabolites individually, we observed an increase of 15-epi-15-E2t-IsoP and 15-keto-15-F2t-IsoP, but this change was not linked to physical exercise directly since the increase was in the ACJ stage, when compared to the placebo. This result suggests a potential role for the compounds from ACJ intake in these IsoP pathways. Recent reports have shown that the E-type IsoPs are potent vasoconstrictors at low nanomolar concentrations.41 15-E2t-IsoP (also referred to as 8-iso-PGE2 or iPE2-III) was found to be a powerful and efficient constrictor in the ductus arteriosus of chicken, acting through the thromboxane receptor.68 Also, other studies with animals have shown both vasoconstrictive and vasodilatory effects of 15-E2t-IsoP, suggesting a biological activity of this molecule in the cardiovascular system.69 On the other hand, 15-keto-15-F2t-IsoP is a metabolite derived from 15-F2t-IsoP. In an animal study, it was demonstrated that this IsoP probably acted as a partial agonist at the TP-receptor, mediating contraction and inducing a weak endothelium-independent relaxation at high concentrations.70 Therefore, the increase in the abundance of these metabolites could reflect the participation of the compounds from ACJ – for example, the flavonoids (polyphenols)71 – or of proline betaine, ferulic acid, or other metabolic derivatives (nutritional biomarkers)72 in the stimulation of some IsoPs related to the effects on vascular smooth muscle. Also, it should not be forgotten that, along with phytochemicals, ACJ contains a variety of vitamins, minerals, and fiber that could have influenced this result.73,74
Metabolites derived from eicosapentaenoic acid and dihomo-γ-linolenic acid
Regarding metabolites derived from DGLA, PGE1α was detected and the Friedman test revealed significant changes among the experimental periods (χ2 (3) = 29.624, P ≤ 0.001). The Wilcoxon test showed that the CP-T value was significantly lower (C-T, Z = −3.408; placebo, Z = 3.294; ACJ, Z = −3.324, P = 0.001 in all cases) compared to most of the other stages (Table 3). According to the literature, through a series of free radical reactions, COX metabolizes DGLA and AA to form various bioactive metabolites – namely, the 1 and the 2 series of prostaglandins (PG1 and PG2), respectively. Unlike the PG2s, which are viewed as pro-inflammatory, the PG1s possess anti-inflammatory and anticancer activity.19 During our study, PGE1 was detected in all the stages, showing statistically significant differences (Table 3). These results suggest a decrease of this metabolite in urine when there is a decline in ECOs, although the values during C-T were higher than in C-B, since the acute physical exercise could have stimulated this pathway. PGE1 has been shown to possess anti-inflammatory properties and to modulate vascular reactivity.75 On the other hand, 15-E1t-IsoP was mainly detected in C-B (0.5 ± 0.1 μg per 24 h), suggesting that physical exercise is an external factor that could have influenced the diminution of its values.
Regarding the metabolites derived from EPA, 8-epi-8-F3t-IsoP was not detected and 8-F3t-IsoP was detected only during C-B (3.4 ± 2.3 μg per 24 h). The elite training decreased the values of 8-F3t-IsoP, suggesting again that physical exercise is an external factor that could influence the reduction of biomarkers concomitantly with the decline in the training loads of the athletes (CP-T). These IsoPs are formed by the free radical-induced peroxidation of EPA in vivo and in vitro. The F3-IsoPs are spontaneously generated in abundance in situ in response to OS and both are useful as biomarkers of OS.23,76
Conclusions
This study contributes to a better comprehension of the behavior of urinary biomarkers related to OS and inflammation status (IsoPs, LTs, PGs, and TXs) in athletes after an elite training period and supplementation of 200 mL of ACJ (a functional beverage rich in polyphenols). The findings indicate that physical exercise is an external factor that influenced mainly the OS biomarkers (F2-IsoPs) and inflammation biomarkers (11-dh-TxB2, PGE2, PGDM, tetranor-PGFM, PGF1α, PGE1, and LTE4) in triathletes. Furthermore, our collective results regarding ACJ intake show that supplementation stimulated the excretion of some metabolites related to vascular homeostasis and smooth muscle (15-epi-15-E2t-IsoPs, 15-keto-F2t-IsoP, 20-OH-PGE2, PGE2, LTE4, and LTB4), indicating a potential role in the cardiovascular system. This work could help to increase our knowledge about the effect of chronic exercise and sports drinks on human lipid metabolism. Moreover, it could aid the design of new beverages for athletes.
Author contribution
LA García-Flores carried out the analytical processes and wrote and discussed the present paper. S Medina, C Gómez, and C Wheelock supervised the analytical processes and developed the discussion of the paper. R Cejuela (coach) monitored the physical exercise training of the triathletes. J M Martínez-Sanz was the nutritionist of the triathletes and monitored the nutritional plan. C Oger, Jean-Marie Galano, and Thierry Durand provided the markers for the study and helped with the review of the manuscript. A Hernández-Sáez provided help in the analytical processes. Federico Ferreres helped with the experimental procedures linked to UHPLC-QqQ-MS/MS. Ángel Gil-Izquierdo and Sonia Medina designed, supervised, and discussed this research work.
Conflicts of interest
The authors declare that they have no conflict of interest.
Acknowledgements
This study was supported by the Project AGL2011-23690 (CICYT) (Spanish Ministry of Economy and Competitiveness). This work was partially funded by the “Fundación Séneca de la Región de Murcia” Grupo de Excelencia 19900/GERM/15. This work is included in the framework of the collaboration between the Spanish Research Council (CEBAS-CSIC) and CNRS-University of Montpellier as “Projets Internationaux de Cooperation Scientifique” (PICS-2015-261141). LAGF was granted a pre-doctoral FPI fellowship by the Spanish government as well as a scholarship that allowed the collaboration with Dr Craig Wheelock's group (Department of Medical Biochemistry and Biophysics (MBB)) at the Karolinska Institute. The authors are also grateful to the University of Alicante for its collaboration. Sonia Medina was appointed under a research contract from the Project AGL2011-23690 (CICYT).
References
- K. H. Myburgh, Polyphenol supplementation: benefits for exercise performance or oxidative stress?, Sports Med., 2014, 44(Suppl 1), S57–S70 CrossRef PubMed.
- T. T. Peternelj and J. S. Coombes, Antioxidant supplementation during exercise training: beneficial or detrimental?, Sports Med., 2011, 41, 1043–1069 CrossRef PubMed.
- J. M. Morillas-Ruiz, J. A. Villegas Garcia, F. J. López, M. L. Vidal-Guevara and P. Zafrilla, Effects of polyphenolic antioxidants on exercise-induced oxidative stress, Clin. Nutr., 2006, 25, 444–453 CrossRef CAS PubMed.
- A. Sureda, S. Tejada, M. Bibiloni Mdel, J. A. Tur and A. Pons, Polyphenols: well beyond the antioxidant capacity: polyphenol supplementation and exercise-induced oxidative stress and inflammation, Curr. Pharm. Biotechnol., 2014, 15, 373–379 CAS.
- S. Medina, R. Dominguez-Perles, R. Cejuela-Anta, D. Villano, J. M. Martinez-Sanz, P. Gil, C. Garcia-Viguera, F. Ferreres, J. I. Gil and A. Gil-Izquierdo, Assessment of oxidative stress markers and prostaglandins after chronic training of triathletes, Prostaglandins Other Lipid Mediators, 2012, 99, 79–86 CrossRef CAS PubMed.
- S. Lafay, C. Jan, K. Nardon, B. Lemaire, A. Ibarra, M. Roller, M. Houvenaeghel, C. Juhel and L. Cara, Grape extract improves antioxidant status and physical performance in elite male athletes, J. Sports Sci. Med., 2009, 8, 468–480 Search PubMed.
- E. I. Varamenti, A. Kyparos, A. S. Veskoukis, M. Bakou, S. Kalaboka, A. Z. Jamurtas, Y. Koutedakis and D. Kouretas, Oxidative stress, inflammation and angiogenesis markers in elite female water polo athletes throughout a season, Food Chem. Toxicol., 2013, 61, 3–8 CrossRef CAS PubMed.
- A. J. Braakhuis, W. G. Hopkins and T. E. Lowe, Effects of dietary antioxidants on training and performance in female runners, Eur. J. Sports Sci., 2014, 14, 160–168 CrossRef PubMed.
- A. M. Petersen and B. K. Pedersen, The anti-inflammatory effect of exercise, J. Appl. Physiol., 2005, 98, 1154–1162 CrossRef CAS PubMed.
- S. J. Stear, L. M. Burke and L. M. Castell, BJSM reviews: A–Z of nutritional supplements: dietary supplements, sports nutrition foods and Ergogenic aids for health and performance Part 3, Br. J. Sports Med., 2009, 43, 890–892 CrossRef CAS PubMed.
- N. A. Lewis, G. Howatson, K. Morton, J. Hill and C. R. Pedlar, Alterations in redox homeostasis in the elite endurance athlete, Sports Med., 2015, 45, 379–409 CrossRef PubMed.
- D. C. Nieman and S. H. Mitmesser, Potential impact of nutrition on immune system recovery from heavy exertion: A metabolomics perspective, Nutrients, 2017, 9, 513 CrossRef PubMed.
- D. Balgoma, A. Checa, D. G. Sar, S. Snowden and C. E. Wheelock, Quantitative metabolic profiling of lipid mediators, Mol. Nutr. Food Res., 2013, 57, 1359–1377 CAS.
- M. C. Noverr, J. R. Erb-Downward and G. B. Huffnagle, Production of eicosanoids and other oxylipins by pathogenic eukaryotic microbes, Clin. Microbiol. Rev., 2003, 16, 517–533 CrossRef CAS PubMed.
- M. Malaguti, C. Angeloni and S. Hrelia, Polyphenols in exercise performance and prevention of exercise-induced muscle damage, Oxid. Med. Cell. Longevity, 2013, 2013, 9 Search PubMed.
- L. J. Roberts and J. D. Morrow, Measurement of F(2)-isoprostanes as an index of oxidative stress in vivo, Free Radical Biol. Med., 2000, 28, 505–513 CrossRef CAS PubMed.
- C. D. Funk, Prostaglandins and leukotrienes: advances in eicosanoid biology, Science, 2001, 294, 1871–1875 CrossRef CAS PubMed.
- E. Ricciotti and G. A. FitzGerald, Prostaglandins and inflammation, Arterioscler., Thromb., Vasc. Biol., 2011, 31, 986–1000 CrossRef CAS PubMed.
- X. Wang, Y. Lin H Fau - Gu and Y. Gu, Multiple roles of dihomo-gamma-linolenic acid against proliferation diseases, Lipids Health
Dis., 2012, 11, 25 CrossRef CAS PubMed.
- R. C. Murphy and M. A. Gijon, Biosynthesis and metabolism of leukotrienes, Biochem. J., 2007, 405, 379–395 CrossRef CAS PubMed.
- G. L. Milne, H. Yin, K. D. Hardy, S. S. Davies and L. J. Roberts 2nd, Isoprostane generation and function, Chem. Rev., 2011, 111, 5973–5996 CrossRef CAS PubMed.
- K. Svanborg, M. Bygdeman and P. Eneroth, The F and 19-hydroxy F prostaglandins and their 8β-isomers in human seminal plasma: Data on chromatography and mass spectrometry, Biol. Mass Spectrom., 1983, 10, 495–498 CrossRef CAS PubMed.
- L. Gao, H. Yin, G. L. Milne, N. A. Porter and J. D. Morrow, Formation of F-ring isoprostane-like compounds (F3-isoprostanes) in vivo from eicosapentaenoic acid, J. Biol. Chem., 2006, 281, 14092–14099 CrossRef CAS PubMed.
- R. Imbusch and M. J. Mueller, Formation of isoprostane F2-like compounds (phytoprostanes F1) from α-linolenic acid in plants, Free Radical Biol. Med., 2000, 28, 720–726 CrossRef CAS PubMed.
- W. L. Smith, Cyclooxygenases, peroxide tone and the allure of fish oil, Curr. Opin. Cell Biol., 2005, 17, 174–182 CrossRef CAS PubMed.
- M. D. Cabañas Armesilla, I. Maestre López and A. Herrero de Lucas, in Introducción de la técnica antropométrica. Método, ed. C. d. Cineantropometría, CTO, Madrid, 2009 Search PubMed.
- J. R. Alvero Ramón, M. D. Cabañas-Armesilla, A. Herrero de Lucas, L. Martínez Riaza, C. Moreno Pascua, J. Porta Manzañido, M. Sillero Quintana and J. E. Sirvent Belando, Protocolo de valoración de la composición corporal para el reconocimiento médico-deportivo. Documento de Consenso del Grupo Español de Cineantropometría (GREC) de la Federación Española de Medicina del Deporte (FEMEDF), Arch. Med. Dep, 2010, 27, 330–334 Search PubMed.
- R. T. Withers, N. P. Craig, P. C. Bourdon and K. I. Norton, Relative body fat and anthropometric prediction of body density of male athletes, Eur. J. Appl. Physiol., 1987, 56, 191–200 CrossRef CAS PubMed.
- R. C. Lee, Z. Wang, M. Heo, R. Ross, I. Janssen and S. B. Heymsfield, Total-body skeletal muscle mass: development and cross-validation of anthropometric prediction models, Am. J. Clin. Nutr., 2000, 72, 796–803 CAS.
- B. E. Ainsworth, W. L. Haskell, M. C. Whitt, M. L. Irwin, A. M. Swartz, S. J. Strath, W. L. O'Brien, D. R. Bassett Jr., K. H. Schmitz, P. O. Emplaincourt, D. R. Jacobs Jr. and A. S. Leon, Compendium of physical activities: an update of activity codes and MET intensities, Med. Sci. Sports Exercise, 2000, 32, S498–S504 CrossRef CAS.
- A. E. Jeukendrup, R. L. Jentjens and L. Moseley, Nutritional considerations in triathlon, Sports Med., 2005, 35, 163–181 CrossRef PubMed.
- E. Gonzalez-Molina, D. A. Moreno and C. Garcia-Viguera, Aronia-enriched lemon juice: a new highly antioxidant beverage, J. Agric. Food Chem., 2008, 56, 11327–11333 CrossRef CAS PubMed.
- L. A. Garcia-Flores, S. Medina, R. Cejuela-Anta, J. M. Martinez-Sanz, A. Abellan, H.-G. Genieser, F. Ferreres and A. Gil-Izquierdo, DNA catabolites in triathletes: effects of supplementation with an aronia-citrus juice (polyphenols-rich juice), Food Funct., 2016, 7, 2084–2093 CAS.
- L. A. Garcia-Flores, S. Medina, C. Oger, J.-M. Galano, T. Durand, R. Cejuela, J. M. Martinez-Sanz, F. Ferreres and A. Gil-Izquierdo, Lipidomic approach in young adult triathletes: effect of supplementation with a polyphenols-rich juice on neuroprostane and F2-dihomo-isoprostane markers, Food Funct., 2016, 7, 4343–4355 CAS.
- R. Cejuela-Anta and J. Esteve-Lanao, Training load quantification in triathlon, J. Hum. Sports Exercise, 2011, 6, 218–232 CrossRef.
- S. Medina, R. Domínguez-Perles, C. García-Viguera, R. Cejuela-Anta, J. M. Martínez-Sanz, F. Ferreres and A. Gil-Izquierdo, Physical activity increases the bioavailability of flavanones after dietary aronia-citrus juice intake in triathletes, Food Chem., 2012, 135, 2133–2137 CrossRef CAS PubMed.
- J. Borresen and M. I. Lambert, The quantification of training load, the training response and the effect on performance, Sports Med., 2009, 39, 779–795 CrossRef PubMed.
-
McGraw-Hill, Concise Dictionary of Modern Medicine, 2002 Search PubMed.
-
S. Lorna, Clinical Trials: What Patients and Volunteers Need to Know, Oxford University Press, USA, 1st edn, 2010 Search PubMed.
- T. Durand, J.-L. Cracowski, A. Guy and J.-C. Rossi, Syntheses and preliminary pharmacological evaluation of the two epimers of the 5-F2t-isoprostane, Bioorg. Med. Chem. Lett., 2001, 11, 2495–2498 CrossRef CAS PubMed.
- Y. Brinkmann, C. Oger, A. Guy, T. Durand and J.-M. Galano, Total Synthesis of 15-D2t- and 15-epi-15-E2t-Isoprostanes, J. Org. Chem., 2010, 75, 2411–2414 CrossRef CAS PubMed.
- T. Durand, A. Guy, J.-P. Vidal and J.-C. Rossi, Total synthesis of (15R)- and (15S)-F2t-isoprostanes by a biomimetic process using the cyclization of acyclic dihydroxylated octa-5,7-dienyl radicals, J. Org. Chem., 2002, 67, 3615–3624 CrossRef CAS PubMed.
- A. Guy, T. Durand, A. Roland, E. Cormenier and J.-C. Rossi, Total synthesis of ent-15(RS)-2,3-dinor-5,6-dihydro-8-epi-PGF2α, Tetrahedron Lett., 1998, 39, 6181–6184 CrossRef CAS.
- A. Guy, C. Oger, J. Heppekausen, C. Signorini, C. De Felice, A. Fürstner, T. Durand and J.-M. Galano, Oxygenated metabolites of n-3 polyunsaturated fatty acids as potential oxidative stress biomarkers: total synthesis of 8-F3t-IsoP, 10-F4t-NeuroP and [D4]-10-F4t-NeuroP, Chem. – Eur. J., 2014, 20, 6374–6380 CrossRef CAS PubMed.
- D. Balgoma, J. Larsson, J. Rokach, J. A. Lawson, K. Daham, B. Dahlen, S. E. Dahlen and C. E. Wheelock, Quantification of lipid mediator metabolites in human urine from asthma patients by electrospray ionization mass spectrometry: controlling matrix effects, Anal. Chem., 2013, 85, 7866–7874 CrossRef CAS PubMed.
- S. Medina, R. Dominguez-Perles, J. I. Gil, F. Ferreres, C. Garcia-Viguera, J. M. Martinez-Sanz and A. Gil-Izquierdo, A ultra-pressure liquid chromatography/triple quadrupole tandem mass spectrometry method for the analysis of 13 eicosanoids in human urine and quantitative 24 hours values in healthy volunteers in a controlled constant diet, Rapid Commun. Mass Spectrom., 2012, 26, 1249–1257 CrossRef CAS PubMed.
- B. K. Matuszewski, M. L. Constanzer and C. M. Chavez-Eng, Strategies for the assessment of matrix effect in quantitative bioanalytical methods based on HPLC–MS/MS, Anal. Chem., 2003, 75, 3019–3030 CrossRef CAS PubMed.
-
FDA, U.S. Department of Health and Human Services Food and Drug Administration (2001) Guiadance for Industry: bioanalytical method validation, http://www.fda.gov/downloads/Drugs/Guidances.
- K. Appel, P. Meiser, E. Millan, J. A. Collado, T. Rose, C. C. Gras, R. Carle and E. Munoz, Chokeberry (Aronia melanocarpa (Michx.) Elliot) concentrate inhibits NF-kappaB and synergizes with selenium to inhibit the release of pro-inflammatory mediators in macrophages, Fitoterapia, 2015, 105, 73–82 CrossRef CAS PubMed.
- J. F. Reis, V. V. S. Monteiro, R. de Souza Gomes, M. M. do Carmo, G. V. da Costa, P. C. Ribera and M. C. Monteiro, Action mechanism and cardiovascular effect of anthocyanins: a systematic review of animal and human studies, J. Transl. Med., 2016, 14, 315 CrossRef PubMed.
- W. L. Song, M. Wang, E. Ricciotti, S. Fries, Y. Yu, T. Grosser, M. Reilly, J. A. Lawson and G. A. FitzGerald, Tetranor PGDM, an abundant urinary metabolite reflects biosynthesis of prostaglandin D2 in mice and humans, J. Biol. Chem., 2008, 283, 1179–1188 CrossRef CAS PubMed.
- J. D. Morrow, C. Prakash, J. A. Awad, T. A. Duckworth, W. E. Zackert, I. A. Blair, J. A. Oates and L. J. Roberts 2nd, Quantification of the major urinary metabolite of prostaglandin D2 by a stable isotope dilution mass spectrometric assay, Anal. Biochem., 1991, 193, 142–148 CrossRef CAS PubMed.
- G. Astarita, A. C. Kendall, E. A. Dennis and A. Nicolaou, Targeted lipidomic strategies for oxygenated metabolites of polyunsaturated fatty acids, Biochim. Biophys. Acta, 2015, 1851, 456–468 CrossRef CAS PubMed.
- D. Villaño, C. Vilaplana, S. Medina, F. Algaba-Chueca, R. Cejuela-Anta, J. Martínez-Sanz, F. Ferreres and A. Gil-Izquierdo, Relationship between the ingestion of a polyphenol-rich drink, hepcidin hormone, and long-term training, Molecules, 2016, 21, 1333 CrossRef PubMed.
- E. T. Olesen and R. A. Fenton, Is there a role for PGE2 in urinary concentration?, J. Am. Soc. Nephrol., 2013, 24, 169–178 CrossRef CAS PubMed.
- R. Nørregaard, T.-H. Kwon and J. Frøkiær, Physiology and pathophysiology of cyclooxygenase-2 and prostaglandin E2 in the kidney, Kidney Res. Clin. Pract., 2015, 34, 194–200 CrossRef PubMed.
- M. Blatnik and R. C. Steenwyk, Quantification of urinary PGEm, 6-keto PGF(1alpha) and 2,3-dinor-6-keto PGF(1alpha) by UFLC-MS/MS before and after exercise, Prostaglandins Other Lipid Mediators, 2010, 93, 8–13 CrossRef CAS PubMed.
- G. Davi and C. Patrono, Platelet activation and atherothrombosis, N. Engl. J. Med., 2007, 357, 2482–2494 CrossRef CAS PubMed.
- F. Santilli, N. Vazzana, P. Iodice, S. Lattanzio, R. Liani, R. G. Bellomo, G. Lessiani, F. Perego, R. Saggini and G. Davi, Effects of high-amount-high-intensity exercise on in vivo platelet activation: modulation by lipid peroxidation and AGE/RAGE axis, Thromb. Haemostasis, 2013, 110, 1232–1240 CrossRef CAS PubMed.
- P. C. Hollman, A. Cassidy, B. Comte, M. Heinonen, M. Richelle, E. Richling, M. Serafini, A. Scalbert, H. Sies and S. Vidry, The biological relevance of direct antioxidant effects of polyphenols for cardiovascular health in humans is not established, J. Nutr., 2011, 141, 989S–1009S CrossRef CAS PubMed.
- J.-H. Yoon and S. J. Baek, Molecular targets of dietary polyphenols with anti-inflammatory properties, Yonsei Med. J., 2005, 46, 585–596 CrossRef CAS PubMed.
- S. R. Del Giacco, D. Firinu, L. Bjermer and K.-H. Carlsen, Exercise and asthma: an overview, Eur. Clin. Respir. J., 2015, 2, 27984.
- T. S. Hallstrand and W. R. Henderson Jr., Role of leukotrienes in exercise-induced bronchoconstriction, Curr. Allergy Asthma Rep., 2009, 9, 18–25 CrossRef CAS PubMed.
- I. M. El-Akkary, Z. E.-K. Abdel-Fatah, M. E.-S. El-Seweify, G. A. El-Batouti, E. A. Aziz and A. I. Adam, Role of leukotrienes in exercise-induced bronchoconstriction before and after a pilot rehabilitation training program, Int. J. Gen. Med., 2013, 6, 631–636 Search PubMed.
- M. G. Nikolaidis, A. Kyparos and I. S. Vrabas, F2-isoprostane formation, measurement and interpretation: The role of exercise, Prog. Lipid Res., 2011, 50, 89–103 CrossRef CAS PubMed.
- M. J. Jackson, Free radicals in skin and muscle: damaging agents or signals for adaptation?, Proc. Nutr. Soc., 1999, 58, 673–676 CrossRef CAS PubMed.
- Z. Radak, Z. Zhao, E. Koltai, H. Ohno and M. Atalay, Oxygen consumption and usage during physical exercise: the balance between oxidative stress and ROS-dependent adaptive signaling, Antioxid. Redox Signaling, 2013, 18, 1208–1246 CrossRef CAS PubMed.
- S. van der Sterren and E. Villamor, Contractile effects of 15-E2t-isoprostane and 15-F2t-isoprostane on chicken embryo ductus arteriosus, Comp. Biochem. Physiol., Part A: Mol. Integr. Physiol., 2011, 159, 436–444 CrossRef PubMed.
- G. L. Milne, Q. Dai and L. J. Roberts Ii, The isoprostanes—25 years later, Biochim. Biophys. Acta, Mol. Cell Biol. Lipids, 2015, 1851, 433–445 CrossRef CAS PubMed.
- J.-L. Cracowski, L. Camus, T. Durand, P. Devillier, A. Guy, G. Hardy, F. Stanke-Labesque, J.-C. Rossi and G. Bessard, Response of rat thoracic aorta to F2-isoprostane metabolites, J. Cardiovasc. Pharmacol., 2002, 39, 396–403 CrossRef CAS PubMed.
- S. Medina, R. Dominguez-Perles, C. Garcia-Viguera, R. Cejuela-Anta, J. M. Martinez-Sanz, F. Ferreres and A. Gil-Izquierdo, Physical activity increases the bioavailability of flavanones after dietary aronia-citrus juice intake in triathletes, Food Chem., 2012, 135, 2133–2137 CrossRef CAS PubMed.
- R. Llorach, S. Medina, C. Garcia-Viguera, P. Zafrilla, J. Abellan, O. Jauregui, F. A. Tomas-Barberan, A. Gil-Izquierdo and C. Andres-Lacueva, Discovery of human urinary biomarkers of aronia-citrus juice intake by HPLC-q-TOF-based metabolomic approach, Electrophoresis, 2014, 35, 1599–1606 CrossRef CAS PubMed.
- A. Rahal, A. Kumar, V. Singh, B. Yadav, R. Tiwari, S. Chakraborty and K. Dhama, Oxidative stress, prooxidants, and antioxidants: the interplay, BioMed Res. Int., 2014, 2014, 19 Search PubMed.
- T. Turner and B. Burri, Potential nutritional benefits of current citrus consumption, Agriculture, 2013, 3, 170–187 CrossRef.
- G. Levin, K. L. Duffin, M. G. Obukowicz, S. L. Hummert, H. Fujiwara, P. Needleman and A. Raz, Differential metabolism of dihomo-gammalinolenic acid and arachidonic acid by cyclo-oxygenase-1 and cyclo-oxygenase-2: implications for cellular synthesis of prostaglandin E1 and prostaglandin E2, Biochem. J., 2002, 365(Pt 2), 489–496.
- J. Jamil, P. Bankhele, A. Salvi, J. E. Mannix, C. Oger, A. Guy, J.-M. Galano, T. Durand, Y. F. Njie-Mbye, S. E. Ohia and C. A. Opere, Role of the non-enzymatic metabolite of eicosapentaenoic acid, 5-epi-5-F3t-isoprostane in the regulation of [3H]d-aspartate release in isolated bovine retina, Neurochem. Res., 2014, 39, 2360–2369 CrossRef CAS PubMed.
Footnote |
† These two authors have contributed equally to this work. |
|
This journal is © The Royal Society of Chemistry 2018 |
Click here to see how this site uses Cookies. View our privacy policy here.