DOI:
10.1039/C7RA13111A
(Paper)
RSC Adv., 2018,
8, 10158-10162
Efficient protective activity of a planar catechin analogue against radiation-induced apoptosis in rat thymocytes†
Received
7th December 2017
, Accepted 2nd March 2018
First published on 13th March 2018
Abstract
About two thirds of biological damage due to low linear energy transfer (LET) radiation, such as X-rays and the plateau region of heavy-ion beams, is known to be caused by the hydroxyl radical (˙OH), the most powerful reactive oxygen species (ROS), generated via ionisation and excitation of water molecules. Thus, compounds having an efficient scavenging activity against ROS are expected to exhibit a radioprotective activity. A planar catechin analogue, where an isopropyl fragment was introduced into the catechol ring of (+)-catechin, showed an efficient protective effect against X-ray induced apoptosis in rat thymocytes compared to (+)-catechin. The planar catechin scavenged 2,2-diphenyl-1-picrylhydrazyl radicals (DPPH˙) solubilised in water by β-cyclodextrin about 10-fold faster than (+)-catechin in phosphate buffer (0.1 M, pH 7.4) at 298 K. Furthermore, the experimental log
P value of the planar catechin (1.22) is reported to be significantly larger than that of (+)-catechin (0.44). The higher radical-scavenging activity and lipophilicity of the planar catechin than those of (+)-catechin may contribute in part to the higher protective activity against X-ray-induced apoptosis in rat thymocytes.
Introduction
Ionising radiation is one of the effective methods for cancer treatment. To maintain the quality of life (QOL) of cancer patients, the demand for radiotherapy that can maintain the shape of internal organs has been increasing in recent years. However, the adverse effect of radiotherapy on normal tissues adjacent to the tumour cannot be ignored. If the tolerance of normal tissues toward radiation could be improved, higher doses of radiation could be employed.1 It is known that about two thirds of the biological damage due to low linear energy transfer (LET) radiation, such as X-rays and the plateau region of heavy-ion beams, is caused by the hydroxyl radical (˙OH), the most powerful reactive oxygen species (ROS), generated via ionisation and excitation of water molecules.2 Thus, compounds having a scavenging activity against ROS are expected to exhibit a radioprotective activity. Among radical scavengers, amifostine (WR-2721), a thiophosphate, shows a significant radioprotective activity and has been approved by the United States Food and Drug Administration (FDA) for patients undergoing radiotherapy. However, the toxicity of amifostine has greatly restricted its use.3
Because natural antioxidants exhibit efficient radical-scavenging activity and little toxicity, they have recently attracted considerable interest as candidates of radioprotectors.4 Recently, we have developed a simple and easy method for evaluation of radioprotective activity of chemical compounds using rat thymocytes and demonstrated that natural antioxidants, such as (+)-catechin, resveratrol, caffeic acid and quercetin, exhibit an efficient protective activity against X-ray-induced apoptosis in rat cymocytes.5 On the other hand, we have investigated chemical modifications of natural antioxidants to improve their radical-scavenging activity. Introduction of methyl groups at positions ortho and/or para to the phenolic hydroxyl group in antioxidants resulted in a significant enhancement of their radical-scavenging activity.6 Among the chemically modified antioxidants, a planar catechin analogue (Fig. 1), where an isopropyl fragment was introduced into (+)-catechin, exhibited 5-fold more potent radical-scavenging activity compared to (+)-catechin in acetonitrile and a protective effect against Fenton reaction induced DNA damage with no pro-oxidant effect.7 We also reported that the radical-scavenging reaction of (+)-catechin in acetonitrile proceeds via an electron transfer from (+)-catechin to the radical to produce the corresponding radical cation of (+)-catechin, followed by proton transfer.8 The electron-donating isopropyl group at the C6′ position (Fig. 1) in the planar catechin may stabilise the radical cation intermediate, resulting in such an enhanced radical-scavenging activity. In fact, the oxidation potential of the planar catechin is reported to be significantly lower than that of (+)-catechin.7c Furthermore, Bernini et al. reported that a methylated planar catechin derivative is much more easily oxidized than a methylated (+)-catechin derivative.9 The planar catechin also shows several biological activities, such as remarkable α-glucosidase inhibition, and anti-virus and anti-tumour activities.7d,10 We report herein that the enhanced radioprotective activity of the planar catechin against X-ray induced apoptosis in rat thymocytes compared to that of (+)-catechin. The biological data using rat thymocytes together with chemical data, such as radical-scavenging rates of the catechins as well as their lipophilicity provide fundamental and valuable information to develop effective radioprotective agents without toxicity.
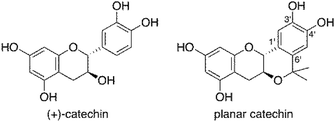 |
| Fig. 1 Chemical structures of catechins. | |
Experimental
Materials
(+)-Catechin was purchased from Sigma and purified by column chromatography on silica gel (7
:
3
:
1 toluene–acetone–methanol). The planar catechin was synthesised according to the procedure reported in the literatures.7a,d,e 2,2-Diphenyl-1-picrylhydrazyl radical (DPPH˙) was commercially obtained from Wako Pure Chemical Ind. Ltd., Japan. β-Cyclodextrin (β-CD) was purchased from Tokyo Chemical Industry Co., Ltd., Japan, recrystallised from water and dried under vaccum at 313 K. The water used in this study was freshly prepared with a Milli-Q system (Millipore Direct-Q UV3). DPPH˙ was solubilised in water by β-CD according to the procedure reported in the literatures.11
Animals
All animal experiments were performed in National Institute of Radiological Sciences (NIRS), conformed to institutional guidelines, and were approved by the Institutional Animal Care and Use Committee of NIRS. Male Wister-MS rats were obtained at 8 weeks of age from Japan SLC, Inc. The rats were housed in a temperature- and humidity-controlled room at 23 ± 1 °C and 55 ± 5%, respectively, and maintained for on a 12 h light–dark cycle. The rats were kept two per cage. They received acidified water and diet (MB-1, Funabashi Farm Co., Japan) ad libitum during the experimental period.
Preparation of thymocyte samples
The thymus from 10–15 week old rats was washed in cold Dulbecco's phosphate-buffered saline (Sigma) to remove extra fat and placed in Roswell Park Memorial Institute medium (RPMI 1640) (Sigma) supplemented with 10% fetal bovine serum (FBS). The thymus was cut and squeezed to thymocytes by using tweezers. These cells were suspended through nyon mesh and then centrifuged at 1500 rpm for 5 min at 4 °C with centrifuge (SAKUMA M-160-IV). The cell pellet was resuspended in PRMI 1640 with 10% FBS and the number of cells were counted under a microscope (OLYMPUS IX-70). The cells were seeded in 48 wells plates at a density of 5.0 × 105 cells per well on ice.
X-ray irradiation
Cells were irradiated with X-rays using a Shimadzu Pantak HF-320 at a dose rate of 1.2 Gy min−1. (effective energy 80 keV, tube voltage 200 kV, tube current 20 mA, 0.5 mm aluminum + 0.5 mm copper filter). The radiation dose was determined using a dose meter. The typical microscopic images of the thymocytes before and after X-ray irradiation are shown in our previous paper.5a
Flow cytometry analysis
After irradiation, the cells were incubated for 4 h at 37 °C with 5% CO2. After incubation, the size and number of thymocytes were measured on a flow cytometer FACS Calibur (Becton Dickinson Company). At least 10
000 cells were measured for each sample, and the data were analysed with BD CellQuest Pro software (Becton Dickinson Company).
Spectral and kinetic measurements
The rates of DPPH˙-scavenging reactions by catechins in phosphate buffer (0.1 M, pH 7.4) were determined by monitoring the absorbance change at 527 nm due to DPPH˙ after mixing of β-CD-solubilised DPPH˙ (DPPH˙/β-CD) in water (Milli-Q) with a phosphate buffer solution (0.2 M, pH 7.4) containing catechins at a volumetric ratio of 1
:
1 using a stopped-flow technique on a UNISOKU RSP-1000-02NM spectrophotometer at 298 K. Therefore, the final concentration of the phosphate buffer is 0.1 M. The kobs values were determined by a least-square curve fit using an Apple MacBook Pro personal computer. The first-order plots of ln(A − A∞) vs. time (A and A∞ are denoted as the absorbance at the reaction time and the final absorbance, respectively) were linear until three or more half-lives with the correlation coefficient ρ > 0.999.
Results and discussion
Protective activity of catechins against X-ray induced apoptosis in rat thymocytes
The radioprotective activity of two catechins in Fig. 1 was examined using the assessment method reported in the leteratures.5 The radioprotective activity of chemical compounds is estimated by quantifying the frequency of apoptosis induced by radiation in rat thymocytes. Thymocytes from rats were placed in cell culture medium (RPMI 1640) supplemented with 10% FBS. The catechins were dissolved in DMSO as a cosolvent and applied to the cell suspensions (1, 10, 100 or 1000 μM). The thymocytes were pretreated with either DMSO 0.1% or the catechins with DMSO 0.1% as a cosolvent. After 2 Gy X-ray irradiation, the cells were incubated for 4 h at 37 °C with 5% CO2. After incubation, the size and number of thymocytes were measured by the flow cytometer. Fig. 2 shows the distribution of the cell size after X-ray irradiation (0 or 2 Gy) with or without catechins (1 mM) (see the Fig. S1, ESI† for other concentrations). The control cells show a sharp peak at around 400 with a small peak at around 250 (Fig. 2a), while 2 Gy X-ray irradiation resulted in the decrease of the peak height at around 400 accompanied by an increase in a peak at around 250 (Fig. 2b). Fig. 2d and f shows the thymocytes treated with 1 mM (+)-catechin and planar catechin, respectively, before X-ray irradiation, where the decrease in the peak at around 400 is significantly inhibited, suggesting that these catechins protects the rat thymocytes. Fig. 2c and e show that 1 mM catechins themselves did not induce apoptosis in the thymocytes. The white and gray bars in Fig. 3 show percentages of the apoptotic cells without and with X-ray irradiation, respectively. When the white bar in the presence of a compound is larger than that in its absence (control), the compound shows toxicity to the thymocytes without X-ray irradiation. Thus, no toxicity was observed by both (+)-catechin and the planar catechin (Fig. 3). On the other hand, a smallar gray bar in the presence of a compound indicates that the compound shows a radioprotective activity. In the control sample, 2 Gy of X-ray irradiation induced apoptosis in about 40% of the thymocytes, while about 20% of the thymocytes died without X-ray irradiation (Fig. 3). The apoptosis induced by X-ray irradiation was efficiently suppressed by the planar catechin pretreatment in a concentration-dependent manner as shown in Fig. 3. The ratio-modification factor (RMF) is defined as the ratio of apoptotic cells with chemicals divided by the ratio of apoptotic cells without chemicals in order to quantify the radioprotective efficiency of the chemicals. The lower RMF values of the compounds are, the higher the radioprotective activity becomes. In this study, 10, 100 and 1000 μM planar catechin exhibited 0.7, 0.1 and 0 of RMF, respectively (Table 1). In contrast, 10, 100 and 1000 μM (+)-catechin showed 0.9, 0.9 and 0.4 RMF, respectively (Table 1). Thus, the planar catechin showed significantly more efficient radioprotective activity against X-ray induced apoptosis in rat thymocytes compared to (+)-catechin.
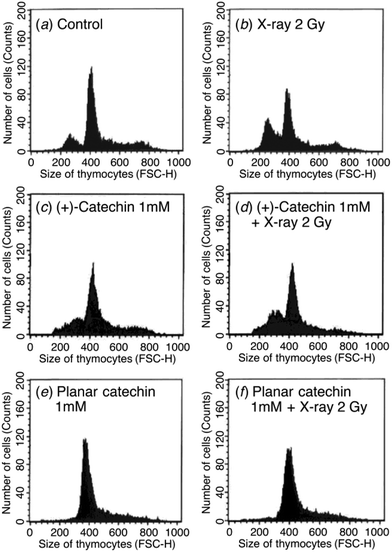 |
| Fig. 2 Flow cytometer analysis of the rat thymocytes. (a) Cells were incubated in the presence of 0.1% DMSO for 4 h. (b) Cells were incubated in the presence of 0.1% DMSO for 4 h after 2 Gy X-ray irradiation. (c) Cells were incubated in the presence of 1 mM (+)-catechin and 0.1% DMSO as a cosolvent for 4 h. (d) Cells were incubated in the presence of 1 mM (+)-catechin and 0.1% DMSO as a cosolvent for 4 h after 2 Gy X-ray irradiation. (e) Cells were incubated in the presence of 1 mM planar catechin and 0.1% DMSO as a cosolvent for 4 h. (f) Cells were incubated in the presence of 1 mM planar catechin and 0.1% DMSO as a cosolvent for 4 h after 2 Gy X-ray irradiation. | |
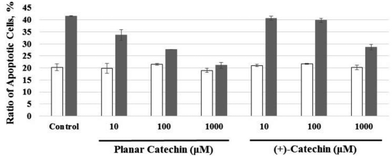 |
| Fig. 3 Effects of various concentrations of the planar catechin and (+)-catechin on apoptosis in rat thymocytes without (white bars) and with (gray bars) 2 Gy X-ray irradiation. | |
Table 1 RMF values of catechins
Concentration/μM |
Ratio of dead cells/% |
RMF protectivity |
No IR |
X-ray 2 Gy |
Control |
20.25 ± 1.37 |
41.51 ± 0.11 |
1.0 ± 0.01 |
Planar catechin 10 |
19.80 ± 2.11 |
33.66 ± 2.33 |
0.7 ± 0.03 |
100 |
21.45 ± 0.37 |
27.66 ± 0.05 |
0.1 ± 0.02 |
1000 |
18.98 ± 0.88 |
21.09 ± 1.22 |
0 ± 0.03 |
(+)-Catechin 10 |
21.01 ± 0.42 |
40.65 ± 0.80 |
0.9 ± 0.04 |
100 |
21.63 ± 0.17 |
39.76 ± 0.86 |
0.9 ± 0.04 |
1000 |
20.23 ± 0.93 |
28.62 ± 1.05 |
0.4 ± 0.05 |
Radical-scavenging activity of the planar catechin in phosphate buffer
Recently, we have reported that 2,2-diphenyl-1-picrylhydrazyl radical (DPPH˙), a stable radial frequently used as a reactivity model of ROS,12–16 could successfully be solubilised in water using β-cyclodextrin (β-CD).11 This enables us to evaluate the radical-scavenging activity of antioxidants in buffer solutions under physiological conditions. Thus, the radical-scavenging activity of (+)-catechin and the planar catechin was investigated using β-CD-solubilised DPPH˙ (DPPH˙/β-CD) in phosphate buffer (0.1 M, pH 7.4). Upon mixing of (+)-catechin in phosphate buffer solution (0.2 M, pH 7.4) with a water (Milli-Q) solution of DPPH˙/β-CD at a volumetric ration of 1
:
1 on a stopped-flow spectrophotometer, the absorption band at 527 nm due to DPPH˙/β-CD immediately decreased with a clear isosbestic point at 429 nm as shown in Fig. 4. The decay of the absorbance at 527 nm obeyed pseudo-first-order kinetics, when the concentration of (+)-catechin was higher than a 10-fold excess of the DPPH˙/β-CD concentration (inset of Fig. 4). The pseudo-first-order rate constant (kobs) linearly increased as the (+)-catechin concentration ([(+)-catechin]) increased (Fig. 5). Based on the slope of the linear plot, the second-order-rate constant (k) for the reaction between (+)-catechin and DPPH˙/β-CD was determined in phosphate buffer (0.1 M, pH 7.4) at 298 K to be 9.9 × 102 M−1 s−1. The k value for the reaction between the planar catechin and DPPH˙/β-CD was also determined in the same manner to be 9.6 × 103 M−1 s−1, which is almost 10-fold larger compared to the case of (+)-catechin. Thus, the planar catechin exhibits a much stronger radical-scavenging activity than (+)-catechin in phosphate buffer (0.1 M, pH 7.4).
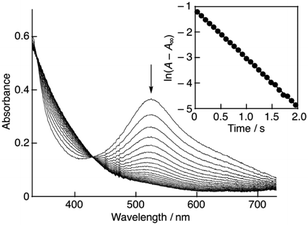 |
| Fig. 4 Spectral change (interval: 20 ms) observed during the reaction of (+)-catechin (1.9 × 10−3 M) with DPPH˙/β-CD (3.2 × 10−5 M) in phosphate buffer (0.1 M, pH 7.4) at 298 K. Inset: the pseud-first-order plot based on the absorbance at 527 nm. | |
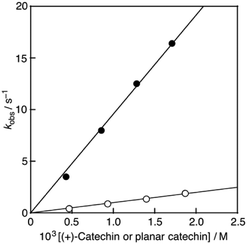 |
| Fig. 5 Plots of kobs vs. [(+)-catechin] (open circles) and [planar catechin] (closed circles). | |
Moreover, the experimental log
P value of the planar catechin (1.22) is reported to be significantly larger than that of (+)-catechin (0.44),17 indicating that the lipophilicity as well as membrane permeability of the planar catechin is higher compared to (+)-catechin.
Conclusions
The planar catechin exhibited a significantly more efficient radioprotective activity against the radiation-induced apoptosis in rat thymocytes compared to (+)-catechin. The higher radical-scavenging activity as well as lipophilicity of the planar catechin may contribute in part to such a higher radioprotective activity than (+)-catechin. To sophisticate the radiation cancer therapy, it is of considerable importance to develop radioprotectors without adverse side effects for normal tissues around tumours. The results obtained in this study suggest that the planar catechin may be one of the promising lead compounds that can be used in combination with radiation cancer therapy. Further experiments are underway to investigate the structure–activity relationships for the effective radioprotective agents using natural and synthetic antioxidants.
Conflicts of interest
There are no conflicts to declare.
Acknowledgements
This work was supported by the FACS support team and animal experiment support team in NIRS. This work was partially supported by Grant-in-Aid (No. 17K18375 to E. S., 26460056 to I. N. and 15K18901 to K. I.) from the Ministry of Education, Culture, Sports, Science and Technology, Japan.
Notes and references
- H. Stone, J. Moulder, C. Coleman, K. Ang, M. Anscher, M. Barcellos-Hoff, W. Dynan, J. Fike, D. Grdina, J. Greenberger, M. Hauer-Jensen, R. Hill, R. Kolesnick, T. Macvittie, C. Marks, W. McBride, N. Metting, T. Pellmar, M. Purucker, M. Robbins, R. Schiestl, T. Seed, J. Tomaszewski, E. Travis, P. Wallner, M. Wolpert and D. Zaharevitz, Radiat. Res., 2004, 162, 711 CrossRef CAS PubMed.
- Radiobiology for the Radiologist, ed. E. J. Hall and A. J. Giaccia, Lippincott Williams & Wilkins, Philadelphia, PA, 7th edn, 2012, ch. 1, pp. 3–11 Search PubMed.
- E. A. Bump and K. Malaker, Radioprotectors: Chemical, Biological, and Clinical Perspectives, CRC Press, Boca Raton, FL, 1997 Search PubMed.
- V. Fuchs-Tarlovsky, Nutrition, 2013, 29, 15 CrossRef CAS PubMed.
-
(a) E. Sekine-Suzuki, I. Nakanishi, T. Shimokawa, M. Ueno, K. Matsumoto and T. Murakami, Anal. Chem., 2013, 85, 7650 CrossRef CAS PubMed;
(b) E. Sekine, T. Shimokawa, M. Ueno, E. Nakamura, M. Nakawatari, T. Murakami, T. Imai, K. Anzai, K. Matsumoto and I. Nakanishi, Jpn. pat., 5794491, 2015;
(c) S. Uzura, E. Sekine-Suzuki, I. Nakanishi, M. Sonoda and S. Tanimori, Bioorg. Med. Chem. Lett., 2016, 26, 3886 CrossRef CAS PubMed.
-
(a) K. Fukuhara, I. Nakanishi, A. Matsuoka, T. Matsumura, S. Honda, M. Hayashi, T. Ozawa, N. Miyata, S. Saito, N. Ikota and H. Okuda, Chem. Res. Toxicol., 2008, 21, 282 CrossRef CAS PubMed;
(b) K. Imai, I. Nakanishi, A. Ohno, M. Kurihara, N. Miyata, K. Matsumoto, A. Nakamura and K. Fukuhara, Bioorg. Med. Chem. Lett., 2014, 24, 2582 CrossRef CAS PubMed;
(c) K. Imai, I. Nakanishi, K. Ohkubo, Y. Ohba, T. Arai, M. Mizuno, S. Fukuzumi, K. Matsumoto and K. Fukuhara, RSC Adv., 2017, 7, 17968 RSC.
-
(a) K. Fukuhara, I. Nakanishi, H. Kansui, E. Sugiyama, M. Kimura, T. Shimada, S. Urano, K. Yamaguchi and N. Miyata, J. Am. Chem. Soc., 2002, 124, 5952 CrossRef CAS PubMed;
(b) K. Fukuhara, I. Nakanishi, T. Shimada, K. Ohkubo, K. Miyazaki, W. Hakamata, S. Urano, T. Ozawa, H. Okuda, N. Miyata, N. Ikota and S. Fukuzumi, Chem. Res. Toxicol., 2003, 16, 81 CrossRef CAS PubMed;
(c) I. Nakansihi, K. Ohkubo, K. Miyazaki, W. Hakamata, S. Urano, T. Ozawa, H. Okuda, S. Fukuzumi, N. Ikota and S. Fukuzumi, Chem. Res. Toxicol., 2004, 17, 26 CrossRef PubMed;
(d) W. Hakamata, I. Nakanishi, Y. Masuda, T. Shimizu, H. Higuchi, Y. Nakamura, S. Saito, S. Urano, T. Oku, T. Ozawa, N. Ikota, N. Miyata, H. Okuda and K. Fukuhara, J. Am. Chem. Soc., 2006, 128, 6524 CrossRef CAS PubMed;
(e) K. Imai, I. Nakanishi, K. Anzai, T. Ozawa, N. Miyata, S. Urano, H. Okuda, A. Nakamura and K. Fukuhara, Chem. Lett., 2011, 40, 1417 CrossRef CAS;
(f) M. Mizuno, I. Nakanishi, S. Matsubayashi, K. Imai, T. Arai, K. Matsumoto and K. Fukuhara, Bioorg. Med. Chem. Lett., 2017, 27, 1041 CrossRef CAS PubMed;
(g) M. Mizuno, I. Nakanishi, K. Matsumoto and K. Fukuhara, Bioorg. Med. Chem. Lett., 2017, 27, 5010 CrossRef CAS PubMed.
- I. Nakanishi, K. Miyazaki, T. Shimada, K. Ohkubo, S. Urano, N. Ikota, T. Ozawa, S. Fukuzumi and K. Fukuhara, J. Phys. Chem. A, 2002, 106, 11123 CrossRef CAS.
-
(a) R. Bernini, F. Crisante, P. Gentili, F. Morana, M. Pierini and M. Piras, J. Org. Chem., 2011, 76, 820 CrossRef CAS PubMed;
(b) R. Bernini, F. Crisante, P. Gentili, S. Menta, F. Morana and M. Pierini, RSC Adv., 2014, 4, 8183 RSC.
- W. Hakamata, M. Muroi, T. Nishio, T. Oku, A. Takatsuki, H. Osada, K. Fukuhara, H. Okuda and M. Kurihara, J. Appl. Glycosci., 2006, 53, 149 CrossRef CAS.
-
(a) I. Nakanishi, K. Ohkubo, K. Imai, M. Kamibayashi, Y. Yoshihashi, K. Matsumoto, K. Fukuhara, K. Terada, S. Itoh, T. Ozawa and S. Fukuzumi, Chem. Commun., 2015, 51, 8311 RSC;
(b) I. Nakanishi, K. Ohkubo, M. Kamibayashi, Y. Ogawa, T. Ozawa, K. Matsumoto and S. Fukuzumi, ChemistrySelect, 2016, 1, 3367 CrossRef CAS;
(c) I. Nakanishi, S. Yamashita, T. Shimokawa, M. Kamibayashi, E. Sekine-Suzuki, M. Ueno, Y. Ogawa, T. Ozawa and K. Matsumoto, Org. Biomol. Chem., 2018, 16, 1272 RSC.
- M. S. Blois, Nature, 1958, 181, 1199 CrossRef CAS.
-
(a) B. Li and D. A. Pratt, Free Radical Biol. Med., 2015, 82, 187 CrossRef CAS PubMed;
(b) K. U. Ingold and D. A. Pratt, Chem. Rev., 2014, 114, 9022 CrossRef CAS PubMed.
- R. Amorati and L. Valgimigli, Free Radical Res., 2015, 49, 633 CrossRef CAS PubMed.
- M. C. Foti, J. Agric. Food Chem., 2015, 63, 8765 CrossRef CAS PubMed.
- K. Pyrzynska and A. Pękal, Anal. Methods, 2013, 5, 4288 RSC.
- B. Poaty, S. Dumarçay and D. Perrin, Eur. Food Res. Technol., 2009, 230, 111 CrossRef CAS.
Footnote |
† Electronic supplementary information (ESI) available: Flow cytometric figures. See DOI: 10.1039/c7ra13111a |
|
This journal is © The Royal Society of Chemistry 2018 |
Click here to see how this site uses Cookies. View our privacy policy here.